Mechanism of the promotion of GEFS+ by the STAT3-mediated expression of interleukin-6
Introduction
Genetic epilepsy with febrile seizures plus (GEFS+) was developed from the concept of generalized epilepsy with febrile seizures (FS) plus, and this condition is usually considered as a genetic “ion channelopathy” (1,2). Sheffer for the first time proposed that GEFS+ is a familial autosomal dominant genetic disease with high incidence in children (3). In 2001, the International League against Epilepsy officially labeled GEFS+ a new type of epilepsy syndrome (4). The genotypes and phenotypes of GEFS+ have certain heterogeneity, owing to the influence of various complex factors, at least 4 genetic loci include SCNB1 (C121W), GABRG2 (K289M and R43Q), SCN1A (R1648H and T875M) and MASS1 (S2652X) (5,6) were related to the onset of GEFS+, it is possible for the heterozygote patients of autosomal dominant inheritance to appear in different phenotypes, involving various epilepsy syndromes, and partial seizures are independent of FS (7). Characteristically, GEFS+ involves an extensive range of clinical manifestations, including the types of epilepsy associated with FS, such as autosomal dominant genetic FS and partial epilepsy with febrile seizures plus (PEFS+) (8,9). Among them, FS is the most common convulsive disease in children, and it usually occurs at the age of 3 months to 5 years old. A sudden outbreak of convulsive symptoms may occur when the body temperature abnormally rises above 38 ℃. The overall prevalence of FS in children is 2–5%, and patients are often found to have a family history of FS, showing a clear hereditary property. Corresponding family-based linkage analysis has shown that the autosomal dominant inheritance of the disease is accompanied by different penetrance, with related gene loci located on chromosomes 19p and 8q13-21 (10). Meanwhile, FS plus (FS+) is featured by afebrile generalized tonic-clonic seizures (GTCS) in children over 6 years old and occasional FS or FS+ with other systemic seizures, such as loss of consciousness, muscle spasm, and atonic seizures. Although less frequent, cases such as afebrile tonic-clonic seizures (TCS), juvenile myoclonic epilepsy, myoclonic absence epilepsy (MAE), and Dravet syndrome may occur in the clinical setting (11,12). Clinical studies have shown that the brain gradually matures as children age, and various clinical symptoms of GEFS+ can disappear spontaneously. However, a small number of patients experience the continuous presence of symptoms into adulthood. Nearly one-third of patients using anti-epileptic drugs (AEDs) experience no improvement in the symptoms of epilepsy, thus aggravating their poor prognosis (13,14). Considering the complexity of clinical manifestations of FS-related epilepsy, especially some severe phenotypes with high difficulty in treatment and poor prognosis, an effective therapeutic approach that can widely deal with and alleviate various clinical symptoms of GEFS+ should be developed to reduce the pain of children and their families.
The pathological process of epilepsy may exhibit an intimate association with a series of cytokines, including inflammatory factors (15). In the pathogenesis of epilepsy with FS, a series of complex inflammatory cascade reactions can result in the accumulation of pro-inflammatory cytokines, blood-brain barrier disruption, and the generalization of neural excitations in the brain, thus increasing the susceptibility of epilepsy and aggravating the damage of nerve cells (16). Moreover, children with recurrent FS may have cellular and humoral immune dysfunction that can enhance the symptoms of epilepsy with FS (17,18). Therefore, immunomodulation and cytokine expression regulation are important to GEFS+ treatment, prognosis improvement, prevention, and treatment. In a study of patients with Rasmussen encephalitis, the activation of microglia, astrocytes, endothelial cells of the blood-brain barrier, peripheral immune cells, and a series of inflammatory mediators could induce chronic encephalitis, and some patients with encephalitis with immune activation were accompanied with epilepsy, similar to autoimmune disease that might result in a high incidence of epilepsy (19). Moreover, limbic encephalitis is an important factor that affects epileptic seizures (20). Therefore, some cytokines and inflammatory signals may be involved in the regulation of some epileptic disorders. Additionally, the classic ketogenic diet therapy for children and adolescents with intractable epilepsy can achieve antiepileptic effect by regulating the expression levels of cytokines (21). In terms of its mechanism of action, the proposed therapy can induce the activation of nuclear hormone receptor peroxisome proliferator-activated receptor α through the high proportion of fatty acid intake. As a transcription regulator, this receptor can downregulate the expression of nuclear factor (NF)-κB-mediated pro-inflammatory factors to inhibit various seizures and thus mediate the treatment of epilepsy by inhibiting the inflammatory response.
In the present study, a mouse model of specific signal transducer and activator of transcription 3 (STAT3) expression inhibition was used to simulate epilepsy with FS+. This study aimed to verify the potential clinical application value of inflammatory cytokine inhibition therapy in GEFS+ treatment by immune blocking agents and targeted therapy for some inflammatory cytokines. By using the multi-omics and chromosomal microarray methods, novel pathogenic factors in the GEFS+ patients may be found in the follow-up studies, and our study may provide a new way for the effective clinical treatment and prevention of GEFS+. We present the following article in accordance with the ARRIVE reporting checklist (available at https://tp.amegroups.com/article/view/10.21037/tp-22-333/rc).
Methods
Construction of the mouse model
Specific pathogen-free (SPF) one-month-old male C57BL/6 mice (n=16, 8 male and 8 female. The mice were randomly selected for the subsequent experiments) were obtained from Kunshan Yunxuan Laboratory Animal Technology Company Limited (Suzhou, China) and kept under SPF conditions. All animal studies were performed according to the guidelines of the Guidance for Animal Experimentation of Zhejiang Sci-Tech University, and the protocol was approved by the Institutional Animal Care and Use Committee of the Zhejiang Sci-Tech University (No. 201804017). Professor Chen Yuan was aware of the group allocation at the different stages of the experiment. All surgeries were performed under isoflurane anesthesia, and the anesthetized mice were mounted in a stereotaxic frame. The mice received intraperitoneal injection of 55 mg/kg of pentylenetetrazol (PTZ) (22) and were subsequently video-monitored for 20 minutes. The latency to GTCS and deaths were recorded. The epileptiform activity was induced by tail vein injection of glutamatergic analog kainic acid (KA; 10 µM; Sigma-Aldrich, St. Louis, MO, USA). To record the hippocampal electroencephalogram (EEG), we implanted the mice with electrodes and catheters. After the skull was exposed, three holes were drilled on the skull (1.7 mm posterior and 1.0 mm left and right of the bregma; center of the occipital), and the surface of the surgical site was covered and sealed with dental cement. The severity of behavioral seizures was scored according to the modified Racine scale (23) as follows: (I) oral and facial movements; (II) head nodding; (III) forelimb clonus and a lordotic posture; (IV) rearing with forelimb clonus; and (V) rearing, forelimb clonus, and falling. The mice used in our study were euthanized painlessly by decapitation after the experiments. The protocols used in the study were prepared before the study without registration.
Inhibitory effect of Stattic on STAT3 phosphorylation
Stattic is a small non-peptide molecule, which can effectively inhibit the activation and nuclear translocation of STAT3 (24). Stattic can inhibit the binding of DNA to STAT3 dimer selectively and thus suppress STAT3 Tyr705 phosphorylation. In our experiment, Stattic (Selleck, Houston, TX, USA) was selected as the inhibitor of STAT3 activation in mouse samples from the experimental group. It was dissolved into 40 µM mother liquor using dimethyl sulfoxide (DMSO) and refrigerated at −30 ℃ for further use. It was administered through intraperitoneal injection at the concentration of 100 µM/kg. The inhibition effect of STAT3 phosphorylation was verified by western blot analysis.
Real-time quantitative polymerase chain reaction
The total RNA was extracted from the serum samples derived from each group, then the RNA was reverse transcribed to complementary DNA (cDNA) as the templates. Reverse transcription, quantitative polymerase chain reaction (qPCR) normalization, and efficiency correction on β-actin RNA were performed as described in our earlier publication (25). Primers for interleukin-6 (IL-6), tumor necrosis factor (TNF)-α, IL-1β, complement factor H (CFH), and β-actin were obtained from Sangon Biotechnology (Shanghai, China). The primer sequences were as follows: IL-6 F: 5'-TAGTCCTTCCTACCCCAATTTCC-3', R: 5'-TTGGTCCTTAGCCACTCCTTC-3'; TNF-α F: 5'-CCCTCACACTCAGATCATCTTCT-3', R: 5'-GCTACGACGTGGGCTACAG-3'; IL-1β F: 5'-GCAACTGTTCCTGAACTCAACT-3', R: 5'-ATCTTTTGGGGTCCGTCAACT-3'; CFH F: 5'-AGGCTCGTGGTCAGAACAAC-3', R: 5'-GTTAGACGCCACCCATTTTCC-3'; β-actin F: 5'-GGCTGTATTCCCCTCCATCG-3', R: 5'-CCAGTTGGTAACAATGCCATGT-3'.
Western blot
The IL-6, TNF-α, IL-1β, STAT3, and the reference protein [glyceraldehyde-phosphate dehydrogenase (GAPDH)] samples were separated by 12% sodium dodecyl sulfate-polyacrylamide gel electrophoresis (SDS-PAGE), transferred onto 0.45-µm polyvinylidene difluoride (PVDF) membranes (Bio-Rad Laboratories, Hercules, CA, USA) for 90 minutes at 350 mA, and then blocked with tris-buffered saline with Tween 20 (TBST) buffer (50 mM Tris-HCl, 150 mM NaCl, and 0.1% Tween-20, pH 7.4) containing 5% non-fat dry milk (w/v) at 4 ℃ overnight. After washing thrice with TBST for 30 minutes, the blots were incubated with rabbit anti-phospho-STAT3 Ab (1:1,000), rabbit anti-TNF-α Ab (1:1,000), rabbit anti-IL-1β Ab (1:1,000), rabbit anti-STAT3 Ab (1:1,000), rabbit anti-IL-6 Ab (1:1,000), or rabbit anti-GAPDH Ab (1:3,000) (Abcam, Cambridge, MA, USA). The blots were washed thrice with TBST for 30 minutes, and then incubated for 1 hour with the HRP-conjugated goat anti-rabbit IgG Ab (Absin) at room temperature. After washing with TBST, the immunoreactive proteins were visualized using a chemical luminescent immunodetection system (Tanon 4500, Tanon Technology Company, Shanghai, China).
Enzyme-linked immunosorbent assay for IL-6 and CFH quantity analysis
The amount of IL-6 protein in the serum samples and CFH in the hippocampus samples was examined by enzyme-linked immunosorbent assay (ELISA). Each sample was coated onto microtiter wells overnight at 4 ℃. After blocking with 2% bovine serum albumin (BSA) for 2 hours, the wells were incubated with rabbit anti-mIL-6 Ab (or rabbit anti-CFH Ab) for 2 hours at 37 ℃, followed by incubation with the HRP-conjugated goat anti-rabbit Ab (Abcam, China) for 1 hour at 37 ℃. Then, the visualization was developed using a tetramethylbenzidine (TMB) substrate, and the absorbance (A450) value was read by using the Synergy H1 hybrid reader (BioTek Instruments, Winooski, VT, USA).
Flow cytometric analysis
The peripheral blood samples (300 µL each) were derived from the GEFS+ and the control groups using the lymphocyte separation medium to obtain the peripheral blood lymphocytes, and the lymphocytes were analyzed by flow cytometry. The cells were stained for 30 minutes on ice with the following fluorescence-labeled antibodies (1 µg/2×106 cells) obtained from Abcam: anti-mouse CD3-FITC, anti-mouse CD4-FITC, and anti-mouse CD8-PE. The other two groups (GEFS+ and the control groups) of samples with the same amount of cells were stained for 30 minutes on ice with non-related IgG-FITC as the negative control (background values). The lymphocyte typing was conducted with reference to the product manuals (BBI Life Sciences, Shanghai, China). The cells were washed twice with cold D-Hanks and then more than 10,000 cells from each group were analyzed on BD Accuri C6 [Becton, Dickinson, and Co. (BD), Franklin Lakes, NJ, USA]. Data analysis was performed using BD Accuri C6 software (BD).
Statistical analysis
All experiments included western blots, ELISA and flow cytometry, etc. were repeated at least three times, respectively. The differences between the means of the groups were statistically evaluated using Student’s t-test, and the data were expressed as the mean ± SD. The criteria for statistical significance used were *P<0.05, **P<0.01, and ***P<0.001 (GraphPad Prism 6.0; GraphPad Software, San Diego, CA, USA). The data were analyzed with the software SPSS 19.0 (IBM Corp., Armonk, NY, USA).
Results
Increased expression of inflammatory-related factors in GEFS+ modeling mice
After i.p. injection of PTZ, the behavioral seizures of the mouse models were continuously monitored for 6 hours with video recording. The continuous seizure activity of the modeling mice was recorded and it characterized as that persisting beyond 1.5 hours after waking from anesthesia. The secondary generalized convulsive seizures were spasmodically repeated and recorded by the EEG. The control mice (Normal group) received an i.p. injection of isopyknic sterile phosphate buffer saline (PBS). The typical frequent abnormal waves of model mice compared with the control group are shown in Figure 1A. The serum samples were derived from the normal mice (n=8) and the mice carrying GEFS+ (n=8). The qPCR and western bolt analysis results showed that the transcriptional levels of IL-6, IL-1β, and TNF-α in GEFS+ mice were significantly increased compared with those in normal mice (Figure 1B), the expression level of TNF-α showed no significant change, and the levels of IL-6 and IL-1β in the GEFS+ serum remarkably increased compared with the control sample (Figure 1C). According to the results of flow cytometry analysis of peripheral blood lymphocytes in each group, compared to the normal mice [the amount of cluster of differentiation 4+ (CD4+) cells was 41.0%±2.7%, n=3], the amount of GEFS+ CD4+ cells (36.0%±3.3%, n=3) and the CD4+/cluster of differentiation 8+ (CD4+/CD8+) ratio were decreased, whereas the CD8+ increased in the GEFS+ group (from 18.5%±2.2% to 33.9%±4.7%), with significant statistical difference compared with the control group (normal mice, P<0.01, Figure 1D). Hence, with epileptic seizures, a typical inflammatory reaction may occur in mice, and this condition may induce feedback regulation of inflammatory reaction and hence aggravate epileptic seizures.
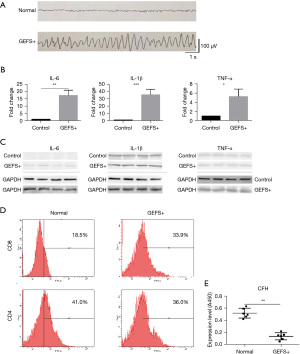
Moreover, in similarity with the temporal lobe epilepsy (TLE) rat model, we found that enhancive proinflammatory factors such as IL-1β, TNF-α, and IL-6 could consequently reduce the expression level of CFH in the hippocampus of GEFS+ mice (Figure 1E), and thus render IL-6, IL-1β, and CFH the inflammatory loop circuit in epileptic seizure in the GEFS+ model. These results showed that these inflammatory factors have a potential correlation with the symptoms of GEFS+, making them the novel therapeutic targets for GEFS+.
Effect of increased IL-6 level on the susceptibility of GEFS+
The dysfunction of the cell-mediated immunity occurs after repeated FS (15). Therefore, we determined the effect of increased expression levels of IL-6 and IL-1β in the susceptibility of GEFS+ mice to epileptic seizure. To confirm the pivotal role of IL-6 and IL-1β in promoting seizures of GEFS+ mice, 8 GEFS+ mice were intranasally administrated with the recombinant mouse IL-6 (mIL-6) and IL-1β (mIL-1β) (100 ng recombinant cytokines dissolved in 0.9% saline for each mouse), another 8 GEFS+ mice intranasally administrated with 0.9% saline were set as the control group. Then, the behavioral seizures of the mice were continuously monitored for 24 hours with video recording, while the electrode-implanted mice also received EEG monitoring at the same time. Interestingly, although we observed a significant increase in the abnormal wave forms after administrating mIL-6 and mIL-1β (Figure 2A), in comparison with the mIL-6-intake mice, convulsive seizures did not increase following mIL-1β administration. Epileptiform activity was induced in the hippocampal and limbic regions by intranasal administration of IL-6 and i.p. injection of KA as detected by EEG monitoring (Figure 2B). Thus, IL-6 is a potential target for GEFS+ therapies.
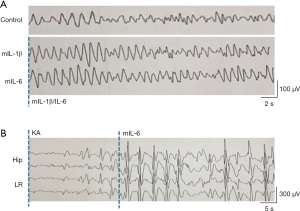
Inhibitory effect of STAT3 suppression on IL-6 expression and GEFS+ outbreak
To explore whether STAT3 could affect seizures by regulating the expression of IL-6 in a GEFS+ mouse model, we administered different concentrations of the STAT3 phosphorylation inhibitor Stattic into GEFS+ mice via i.p. injection. The results of western blot and ELISA showed that the expression of IL-6 significantly decreased under the action of Stattic (Figure 3A,3B). With the inhibition of IL-6 expression, the abnormal seizure electrical signal of GEFS+ mice was significantly inhibited. Meanwhile, EEG signals showed that compared with STAT3-activated mice, no obvious epileptic activity occurred in the hippocampal and limbic regions of STAT3-inhibited GEFS+ mice, accompanied by delayed and flat successive spike waves (Figure 3C).
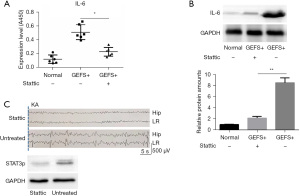
Preliminary study on GEFS+ treatment with targeted inhibition of IL-6 expression
An anti-IL-6 monoclonal antibody (Abcam) was injected intraperitoneally in GEFS+ model mice to evaluate the effect of the neutralizing activity of proinflammatory cytokine IL-6 on the decrease of the critical value of GEFS+ attack. Following the different injection dosages of IL-6 monoclonal antibody, the abnormal seizure electrical signal of GEFS+ mice was remarkably inhibited. Moreover, EEG signals showed that the successive spike waves of GEFS+ mice with the injection of IL-6 monoclonal antibody became delayed and flat compared with that of mice without IL-6 monoclonal antibody injection (Figure 4A), showing the absence of wet-dog shakes in behavior. Correspondingly, after IL-6 was neutralized by anti-IL-6 Ab, the ratio of CD3+/CD8+ T cells in peripheral blood mononuclear cells (PBMCs) remarkably decreased in the GEFS+ mice according to flow cytometry analysis (Figure 4B), and the ratio of CD4/CD8 in anti-IL-6 Ab neutralized GEFS+ mice increased compared with the control group (GEFS+ mice, Figure 4C).
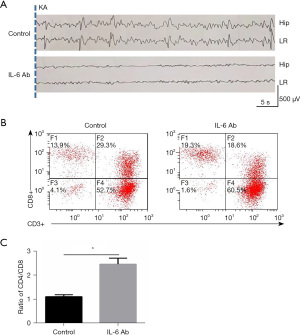
The above experiments may lay a foundation for exploring the effective concentration of an IL-6 antibody to inhibit future outbreaks of GEFS+. Its application in the clinical treatment of GEFS+ may provide a new insight into the effective treatment of GEFS+ and inhibition of epilepsy with FS by integrating with joint therapy by using AEDs.
Discussion
At present, there is still insufficient research on the inflammatory status in various types of epileptic seizures; it is unclear whether the inflammatory mechanism plays a key role in epilepsy. Meanwhile, there have been few studies on the severity of epileptic symptoms caused by the differences in the expression levels of individual inflammatory factors, especially IL-6 (26), Choi et al. (27) suggested that the postictal serum IL-6 levels were not significantly associated with the genotypes of IL-6-572 (rs1800796) in GEFS+, no more potential genetic risk factors in postictal IL-6 were found in the previous studies. Accordingly, this study analyzed the clinical data of early GEFS+ to explore its immunological characteristics and the biological mechanism of promoting GEFS+ attack. In our experiment, epilepsy with FS+ was stimulated by a gene mutation-based mouse model, followed by the application of immune blocking agents and targeted therapy based on some inflammatory cytokines, which was designed to verify the potential clinical application value of inflammatory factor suppressive therapy in GEFS+ treatment.
As evidenced by prior research (28), epileptic seizure can induce the activation of glial cells and other non-neuronal cells, and then stimulate the expression of a large number of inflammatory-related factors, such as IL, interferon, and TNF. It may further promote the inflammatory immune environment in the course of epileptic seizures. According to the published clinical research (29), glucocorticoid and adrenocorticotropic hormones could play an effective therapeutic role in intractable epilepsy in children, and a great deal of glial cell hyperplasia was found in the epileptogenic focus of the brain. This may suggest that inflammation might play an essential role in inducing the pathogenesis of epilepsy and affecting its severity. Furthermore, Lehtimäki et al. (30) discovered in their experiment that the expression of IL-6R and the level of its signal transduction factor Gp130 were significantly increased in the forebrain of epileptic rats. Intranasal administration of IL-6 could also increase the severity of induced seizures in rats (31). Additionally, through the analysis of clinical data, serum IL-6 level was shown to be significantly higher in chronic TLE patients than that of normal group, which also exhibited an obvious increased trend compared with other epilepsy patients without TLE (32), suggesting that the type of epileptic seizure may have an impact on the level of IL-6. Nevertheless, it remains unknown whether serum IL-6 level changes during epileptic seizures in patients with GEFS+ and whether STAT3-IL-6 can promote epileptic seizures in those patients. In our study, a mouse GEFS+ model was established in C57BL/6 mice by using the epileptogenic agent PTZ. The corresponding results revealed that the levels of IL-6 and IL-1β in serum were increased significantly along with typical epileptic versus after KA stimulation (Figure 1C). Meanwhile, according to the analysis of the types of peripheral blood lymphocytes in GEFS+ mice, the proportion of CD4+ lymphocytes decreased significantly, while that of CD8+ lymphocytes increased in GEFS+ mice (Figure 1D). This may reveal the presence of typical inflammatory reaction in mice with epileptic seizures. Besides, intranasal administration of IL-6 to GEFS+ mice further increased the susceptibility of epilepsy, suggesting that inflammatory response may play a feedback regulation role to aggravate epileptic seizures.
Furthermore, in our experiment, following the injection of Stattic, a specific STAT3 phosphorylation inhibitor, into GEFS+ mice, the abnormal electrical signals of epileptic seizures in GEFS+ mice were also significantly inhibited with the suppression of IL-6 expression (Figure 3). At the same time, there was an inhibition of the epileptic activity of mice to a certain extent. A similar phenomenon was observed in GEFS+ mice after intraperitoneal injection of an anti-IL-6 monoclonal antibody. The above results may suggest that the upregulation of IL-6 mediated by STAT3 may be involved in the mechanism of epileptic seizures in GEFS+ mice. Significantly, our study preliminarily reveals the mechanism of some certain cytokines in promoting GEFS+ outbreak, and elucidates the molecular mechanism of inflammatory signaling pathway STAT3-IL-6 involved in the regulation of GEFS+ pathogenesis. This study revealed that the monoclonal antibody can inhibit the expression of pro-inflammatory factors and further prevent GEFS+ attack, which supports that IL-6 is one of the important factors that aggravate the clinical symptoms of GEFS+. The findings of the present study may provide some valuable experimental evidence for further understanding of the key role of cytokines in the development of GEFS+.
Acknowledgments
Funding: This research was funded by Zhejiang Provincial Public Welfare Technology Application Research Project, China (No. LGF18H090012).
Footnote
Reporting Checklist: The authors have completed the ARRIVE reporting checklist. Available at https://tp.amegroups.com/article/view/10.21037/tp-22-333/rc
Data Sharing Statement: Available at https://tp.amegroups.com/article/view/10.21037/tp-22-333/dss
Conflicts of Interest: All authors have completed the ICMJE uniform disclosure form (available at https://tp.amegroups.com/article/view/10.21037/tp-22-333/coif). The authors have no conflicts of interest to declare.
Ethical Statement: The authors are accountable for all aspects of the work in ensuring that questions related to the accuracy or integrity of any part of the work are appropriately investigated and resolved. This study was approved by the Institutional Animal Care and Use Committee of the Zhejiang Sci-Tech University (No. 201804017). All animal studies were performed according to the guidelines of the Guidance for Animal Experimentation of Zhejiang Sci-Tech University.
Open Access Statement: This is an Open Access article distributed in accordance with the Creative Commons Attribution-NonCommercial-NoDerivs 4.0 International License (CC BY-NC-ND 4.0), which permits the non-commercial replication and distribution of the article with the strict proviso that no changes or edits are made and the original work is properly cited (including links to both the formal publication through the relevant DOI and the license). See: https://creativecommons.org/licenses/by-nc-nd/4.0/.
References
- Zhang T, Chen M, Zhu A, et al. Novel mutation of SCN9A gene causing generalized epilepsy with febrile seizures plus in a Chinese family. Neurol Sci 2020;41:1913-7. [Crossref] [PubMed]
- Kaya MA, Erin N, Bozkurt O, et al. Changes of HMGB-1 and sTLR4 levels in cerebrospinal fluid of patients with febrile seizures. Epilepsy Res 2021;169:106516. [Crossref] [PubMed]
- Scheffer IE, Berkovic SF. Generalized epilepsy with febrile seizures plus. A genetic disorder with heterogeneous clinical phenotypes. Brain 1997;120:479-90. [Crossref] [PubMed]
- Engel J JrInternational League Against Epilepsy (ILAE). A proposed diagnostic scheme for people with epileptic seizures and with epilepsy: report of the ILAE Task Force on Classification and Terminology. Epilepsia 2001;42:796-803. [Crossref] [PubMed]
- Belhedi N, Bena F, Mrabet A, et al. A new locus on chromosome 22q13.31 linked to recessive genetic epilepsy with febrile seizures plus (GEFS+) in a Tunisian consanguineous family. BMC Genet 2013;14:93. [Crossref] [PubMed]
- Camfield P, Camfield C. Febrile seizures and genetic epilepsy with febrile seizures plus (GEFS+). Epileptic Disord 2015;17:124-33. [Crossref] [PubMed]
- Fortin O, Vincelette C, Chénier S, et al. Copy number variation in genetic epilepsy with febrile seizures plus. Eur J Paediatr Neurol 2020;27:111-5. [Crossref] [PubMed]
- Scheffer IE, Zhang YH, Jansen FE, et al. Dravet syndrome or genetic (generalized) epilepsy with febrile seizures plus? Brain Dev 2009;31:394-400. [Crossref] [PubMed]
- Heron SE, Regan BM, Harris RV, et al. Association of SLC32A1 Missense Variants With Genetic Epilepsy With Febrile Seizures Plus. Neurology 2021;96:e2251-60. [Crossref] [PubMed]
- Racacho LJ, McLachlan RS, Ebers GC, et al. Evidence favoring genetic heterogeneity for febrile convulsions. Epilepsia 2000;41:132-9. [Crossref] [PubMed]
- Herini ES. Generalized epilepsy with febrile seizures plus (GEFS+) spectrum: clinical manifestations and SCN1A mutations in Indonesian patients. Epilepsy Res 2010;90:132-9. [Crossref] [PubMed]
- Marini C, Scheffer IE, Nabbout R, et al. The genetics of Dravet syndrome. Epilepsia 2011;52:24-9. [Crossref] [PubMed]
- Perucca E, French J, Bialer M. Development of new antiepileptic drugs: challenges, incentives, and recent advances. Lancet Neurol 2007;6:793-804. [Crossref] [PubMed]
- Perucca E. Antiepileptic drugs: evolution of our knowledge and changes in drug trials. Epileptic Disord 2019;21:319-29. [PubMed]
- Yu Q, Zhao MW, Yang P. LncRNA UCA1 Suppresses the Inflammation Via Modulating miR-203-Mediated Regulation of MEF2C/NF-κB Signaling Pathway in Epilepsy. Neurochem Res 2020;45:783-95. [Crossref] [PubMed]
- Librizzi L, Ravizza T, Vezzani A, et al. Expression of Il-1 beta induced by epileptiform activity in the isolated guinea pig brain in vitro. 2010;51:18.
- Fabene PF, Navarro Mora G, Martinello M, et al. A role for leukocyte-endothelial adhesion mechanisms in epilepsy. Nat Med 2008;14:1377-83. [Crossref] [PubMed]
- Vezzani A, Balosso S, Ravizza T. Inflammation and epilepsy. Handb Clin Neurol 2012;107:163-75. [Crossref] [PubMed]
- Wirenfeldt M, Clare R, Tung S, et al. Increased activation of Iba1+ microglia in pediatric epilepsy patients with Rasmussen's encephalitis compared with cortical dysplasia and tuberous sclerosis complex. Neurobiol Dis 2009;34:432-40. [Crossref] [PubMed]
- Spatola M, Dalmau J. Seizures and risk of epilepsy in autoimmune and other inflammatory encephalitis. Curr Opin Neurol 2017;30:345-53. [Crossref] [PubMed]
- Cullingford T. Peroxisome proliferator-activated receptor alpha and the ketogenic diet. Epilepsia 2008;49:70-2. [Crossref] [PubMed]
- Huang X, Zhou C, Tian M, et al. Overexpressing wild-type γ2 subunits rescued the seizure phenotype in Gabrg2+/Q390X Dravet syndrome mice. Epilepsia 2017;58:1451-61. [Crossref] [PubMed]
- Van Erum J, Van Dam D, De Deyn PP. PTZ-induced seizures in mice require a revised Racine scale. Epilepsy Behav 2019;95:51-5. [Crossref] [PubMed]
- Boengler K, Ungefug E, Heusch G, et al. The STAT3 inhibitor stattic impairs cardiomyocyte mitochondrial function through increased reactive oxygen species formation. Curr Pharm Des 2013;19:6890-5. [Crossref] [PubMed]
- Jiang X, Liu G, Hu Z, et al. cGAMP inhibits tumor growth in colorectal cancer metastasis through the STING/STAT3 axis in a zebrafish xenograft model. Fish Shellfish Immunol 2019;95:220-6. [Crossref] [PubMed]
- Alapirtti T, Lehtimäki K, Nieminen R, et al. The production of IL-6 in acute epileptic seizure: A video-EEG study. J Neuroimmunol 2018;316:50-5. [Crossref] [PubMed]
- Choi J, Choi SA, Kim SY, et al. Association Analysis of Interleukin-1β, Interleukin-6, and HMGB1 Variants with Postictal Serum Cytokine Levels in Children with Febrile Seizure and Generalized Epilepsy with Febrile Seizure Plus. J Clin Neurol 2019;15:555-63. [Crossref] [PubMed]
- Vezzani A, Balosso S, Ravizza T. Neuroinflammatory pathways as treatment targets and biomarkers in epilepsy. Nat Rev Neurol 2019;15:459-72. [Crossref] [PubMed]
- Dressler A, Benninger F, Trimmel-Schwahofer P, et al. Efficacy and tolerability of the ketogenic diet versus high-dose adrenocorticotropic hormone for infantile spasms: A single-center parallel-cohort randomized controlled trial. Epilepsia 2019;60:441-51. [Crossref] [PubMed]
- Lehtimäki KA, Peltola J, Koskikallio E, et al. Expression of cytokines and cytokine receptors in the rat brain after kainic acid-induced seizures. Brain Res Mol Brain Res 2003;110:253-60. [Crossref] [PubMed]
- Kalueff AV, Lehtimaki KA, Ylinen A, et al. Intranasal administration of human IL-6 increases the severity of chemically induced seizures in rats. Neurosci Lett 2004;365:106-10. [Crossref] [PubMed]
- Uludag IF, Duksal T, Tiftikcioglu BI, et al. IL-1β, IL-6 and IL1Ra levels in temporal lobe epilepsy. Seizure 2015;26:22-5. [Crossref] [PubMed]
(English Language Editor: J. Jones)