Clinical analysis of the epidemiology and changes in inflammatory indexes of Mycoplasma pneumonia in acute and recovery stage pediatric patients
Introduction
Mycoplasma is the smallest pathogenic microorganism between bacteria and virions that can survive independently (1). So far, 16 species of mycoplasma have been found to act in the human body. Among them, Mycoplasma pneumoniae (MP) is the main mycoplasma that can cause respiratory tract infection (2). Today, the number of children and adolescents with community-acquired pneumonia (CAP) with MP is on the rise. Relevant statistical reports show that the number of children under the age of 5 infected with MP is increasing year by year, which is not conducive to the healthy growth of children (3,4). The widespread distribution of MP pathogens in nature has become a common cause of acute upper and lower respiratory tract infections in children of all ages. According to research statistics, the incidence of MP infection is 9.6–66.7%, which is more common in summer and autumn, and there is no obvious regional difference. Therefore, it will cause sporadic or small-scale epidemics, and the global epidemic trend is 3–5 years/time (5,6). The incubation period of MP infection is 1–3 weeks or more, and the severity of lung inflammation varies in different children. In recent years, studies all over the world have shown that MP infection can not only cause respiratory symptoms, but also cause pulmonary complications, hypoxemia, and multi-system functional damage such as circulation, digestion, and nerves, and even endanger life, seriously reducing children’s quality of life and life safety (7,8).
The onset of MP is slow, and the initial symptoms are mainly headache, loss of appetite, fatigue, and general malaise. Fever occurs 2–3 days later (9), the most prominent manifestation is paroxysmal irritating dry cough at night, and vomiting with pus and blood-like sticky sputum may occur in the late stage. However, most pulmonary signs are inconspicuous, such as wet and dry lung rales (10,11). It has been clinically found that infants and young children are mostly affected by MP and cause severe clinical symptoms such as dyspnea, wheezing, and pulmonary rales. MP infection has been reported to be closely associated with recurrent respiratory tract infections, infectious mononucleosis, recurrent wheezing, and even bronchial asthma in children (12,13).
At present, the pathogenesis of MP is summarized as the theory of MP acting on respiratory epithelial cells and immune damage (14). The main mechanisms of MP are as follows: adhesion of MP, direct invasion of MP, direct cellular damage caused by MP, and the ability of MP to induce systemic immune inflammatory response (15-18). In addition, MP will stimulate the body to produce specific IgG, IgE, and IgM antibodies, increase complement C1q and C3, and a variety of cytokines are disordered, resulting in aggravated inflammatory response and lung injury. More and more researchers believe that the pathogenesis of MP is related to a variety of inflammatory mediators and cytokines, suggesting that there are some abnormal concentrations of inflammatory factors in the acute phase of MP, resulting in low and disordered cellular immunity (19).
In summary, this work further explored the current epidemiology of MPP and investigated the roles of inflammatory cytokines in acute and convalescent phases, including serum tumor necrosis factor-α (TNF-α), interleukin-6 (IL-6), interleukin-8 (IL-8), interleukin-10 (IL-10), and procalcitonin (PCT) in the pathogenesis of MP. Different from previous studies, this work conducted a specific analysis of the related indicators of pleural effusion and pulmonary fibrosis without concurrent pleural effusion, which was more comprehensive. It aimed to provide a theoretical basis for early clinical diagnosis and rational immunotherapy. We present the following article in accordance with the MDAR reporting checklist (available at https://tp.amegroups.com/article/view/10.21037/tp-22-416/rc).
Methods
Participants
In this work, 120 children with MP were selected as the research objects and set as the experimental group. The inclusion criteria were set as follows: the clinical diagnosis of all MP children was based on the inclusion criteria (20) of practical pediatrics. The specific criteria were set as follows: (I) persistent and severe dry cough with obvious signs on X-ray; (II) most white blood cell levels were normal or slightly increased, and erythrocyte sedimentation rate (ESR) was elevated; (III) treatment with penicillin, streptomycin, and sulfa drugs had been ineffective; (IV) serum gelatin particle agglutination (PA) test: single MP antibody titer was 1:160 or higher; (V) enzyme-linked immunosorbent assay (ELISA): single determination of serum MP-immunoglobulin M (IgM) antibody positive. The selected cases were not accompanied by pulmonary tuberculosis, bronchial asthma, and other respiratory diseases. This work also examined chest and abdominal imaging, electrocardiogram, cardiac enzymes, and liver function in all children. X-rays revealed pulmonary fibrosis in 36 of the 40 children with pleural effusion, all of whom were free of antibiotics, corticosteroids, and immunosuppressants before testing.
There were 100 healthy children in the control group without organic diseases and no history of acute infectious diseases in the past 3 months. The patients in this study and their families fully understood the situation and signed the informed consent. The study was conducted in accordance with the Declaration of Helsinki (as revised in 2013). The Medical Ethics Committee of The Third Affiliated Hospital of Jinzhou Medical University was aware of and approved the study (No. KX2022021).
Main experimental reagents
The main reagents required for the experiment are shown in Table 1.
Table 1
Name of experimental reagent | Reagent source |
---|---|
IL-6, IL-8 ELISA reagent kits | Innogenetics, Belgium |
TNF-α, IL-10 reagent kits | Changchun Xinhuayu Biological Equipment Company |
PCT detection kit | Roche Diagnostics (Shanghai) |
TNF-α, tumor necrosis factor-α; IL-6, interleukin 6; IL-8, interleukin 8; IL-10, interleukin 10; PCT, procalcitonin; ELISA, enzyme-linked immunosorbent assay.
Professionals acted in accordance with the experimental protocol, and employed ELISA at 492 nm wavelength detection absorbance (A) value, drew a protein standard curve, and found the corresponding value on the standard curve. In this experiment, the 3-hole operation was used to reduce the experimental error.
Main experimental instruments
The main experimental instruments required in the experiment are shown in Table 2.
Table 2
Experimental instrument name | Experimental instrument source |
---|---|
Low-temperature high-speed centrifuge | Heraeus, Germany |
DH4000A electrothermal thermostat | Tianjin Taiste Instrument Co., Ltd., China |
ELISA-ELM 3000 automatic enzyme marker | DRG, US |
−20 ℃ low humidity refrigerator | China Haier Group |
ELISA, enzyme-linked immunosorbent assay.
In this study, the most suitable and commonly used instruments in recent years were selected to optimize the accuracy of our experimental operation and results, and to provide a good foundation for the clinical application and promotion of this study in the future.
Experimental methods
A total of 220 children were included in this work. According to the diagnostic criteria, they were divided into MMP group (n=120 cases) and control group (n=100 healthy children). Specimen collection method: in fasting state, 3 mL of peripheral venous blood was aseptically drawn from MP children in the experimental group and control group, and the serum was collected after centrifugation and frozen for further use. Of these, 40 children with pleural effusion underwent thoracentesis to collect pleural effusion specimens, which were stored in a −20 ℃ refrigerator and examined uniformly. Detection method: the double antibody sandwich enzyme-linked immunosorbent assay (ELISA) method was used to measure the TNF-α, TGF-β1, IL-2, IL-6, IL-8, IL-10, and IL-13.
Observation indicators
- According to the age range, the children were divided into three age groups: 0–1, 2–6, and 7–14 years old. Among the 120 MPP patients, the number of children in different age groups was counted, and the distribution of MP children of different genders was also analyzed to analyze the epidemiological distribution of MPP.
- The serum TNF-α, IL-6, IL-8, IL-10, and PCT levels of the two groups of children were recorded and compared and analyzed.
- It should record the pulmonary fibrosis, serum and pleural fluid levels of TNF-α, IL-6, IL-8, IL-10, and the changes of PCT on chest X-ray and CT in children with MPP with pleural effusion.
Statistical analysis
All data were statistically analyzed using SPSS 17.0 software (IBM Corp., Chicago, IL, USA). The test results were expressed as mean ± standard deviation (). The two groups were compared by t-test. When P<0.05, the difference was considered statistically significant.
Results
Distribution of MP children in different age groups
Table 3 showed the distribution of children in the three age groups, 0–1, 1–3, 3–7, and 7–14 years old. The results showed that the number of patients in each age group were 28 (23.33%), 34 (28.33%), 42 (35%), and 16 (13.33%). The ratio was significantly higher than other age groups (P<0.05).
Table 3
Age group | Cases | Proportion (%) |
---|---|---|
0–1 year old (baby) | 28 | 23.33 |
1–3 years old (infancy) | 34 | 28.33 |
3–7 years old (preschool) | 42 | 35.00* |
7–14 years old (school age) | 16 | 13.33 |
* indicated that there was a statistical difference (P<0.05). MP, Mycoplasma pneumoniae.
Distribution of children with MP by gender
Table 4 showed the gender distribution of 120 children with MP. Among them, the number of MPP in male children was 64 (53.33%), and the number of MPP in female children was 56 (46.67%). After comparative analysis, there was no significant difference in the proportion of MPP children between the two (P>0.05).
Table 4
Gender | Cases | Proportion (%) |
---|---|---|
Male | 64 | 53.33 |
Female | 56 | 46.67 |
MP, Mycoplasma pneumoniae.
Basic information of children
A total of 220 children were included in this clinical study. The basic characteristics of the MPP group (120 cases) were as follows: 64 males and 56 females, 1–14 years old (7.1±1.4 years). There were 100 healthy controls, 55 males and 45 females, with an average age (6.8±1.5 years). There was no significant difference in age and gender between the two groups (P>0.05).The general situation and X-ray and computed tomography (CT) examination results of the two groups of children in the acute and convalescent stages are shown in Figures 1-3.
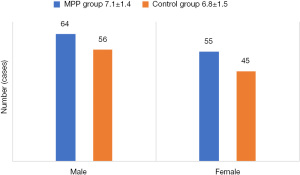
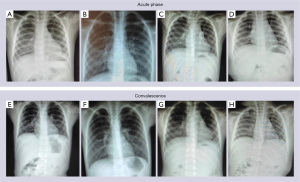
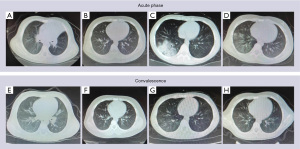
Serum concentrations of TNF-α, IL-6, IL-8, IL-10, and PCT in children with MPP
The concentrations of TNF-α, IL-6, IL-8, IL-10, and PCT in the serum of 120 children with MPP in the acute stage and recovery stage and those of control group participants are shown in Figure 4. To more intuitively reflect the changes in serum concentrations of TNF-α, IL-6, IL-8, IL-10, and PCT in the acute phase and the recovery phase, a line graph (Figure 5) was drawn.
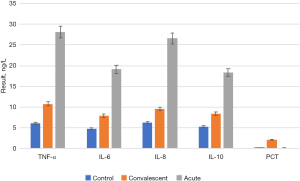
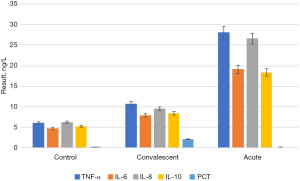
By comparing Figure 4 and Figure 5, it was found that the serum concentrations of TNF-α, IL-6, IL-8, IL-10, and PCT in children with MPP at the acute stage were significantly higher than those in the recovery stage and the control group (P<0.05). Serum concentrations of TNF-α, IL-6, IL-8, IL-10, and PCT in the recovery stage were significantly lower than those in the acute stage (P<0.05), but still higher than those in the control group. There was no significant difference between the recovery period and the control group (P>0.05).
Detection of TNF-α, IL-6, IL-8, IL-10, and PCT concentrations in pleural effusion and serum of children with and without pulmonary fibrosis
The concentrations of TNF-α, IL-6, IL-8, IL-10, and PCT in the pleural effusion and serum of children with and without pulmonary fibrosis are shown in Figure 6. To more intuitively reflect the changes in the concentrations of TNF-α, IL-6, IL-8, IL-10, and PCT in pleural effusion and serum of children with and without pulmonary fibrosis, a three-dimensional (3D) broken line graph was drawn (Figures 7,8).
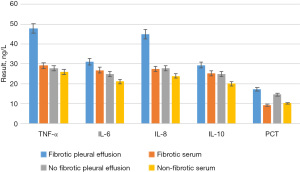
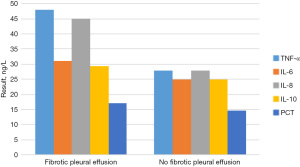
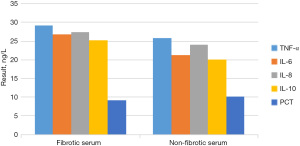
By comparing Figure 6, Figure 7 and Figure 8, it was found that among the 40 children with pleural effusion, chest X-ray examination indicated that 36 of them had pulmonary fibrosis. The concentrations of TNF-α and IL-8 in pleural effusion were significantly higher than those in the group without pulmonary fibrosis, and the differences were statistically significant (P<0.05), yet those of IL-6, IL-10, and PCT were not statistically significant. The concentrations of TNF-α, IL-8, and PCT in pleural fluid of the children with pulmonary fibrosis were significantly higher than those in serum (P<0.05), and those of IL-6 and IL-10 had no statistical significance. The concentrations of TNF-α, IL-6, IL-8, IL-10, and PCT in pleural fluid were not significantly different from those in serum in 6 cases without pulmonary fibrosis (P>0.05).
Discussion
In recent years, the incidence of MP infection has been on the rise. In this work, an epidemiological analysis was conducted on the age and gender of onset of MP. The results showed that the proportion of children aged 3–7 years was significantly higher than that of other age groups (P<0.05), suggesting that preschool children aged 3–7 years were a high-risk group of MPP, and the incidence and gender distribution of MPP were not significantly correlated. This is consistent with the statistical results of most relevant studies. Most studies suggest that the predisposing age of MP is around 5 years old (21,22), and 5 years old is in the middle stage of 3–7 patients, which is in line with the research conclusions. In addition, the study also conducted a lot of analysis and exploration on the changes of inflammatory indicators of MP. The results showed that the serum concentrations of TNF-α, IL-6, IL-8, IL-10, and PCT in MP-infected children were significantly higher than those in the control group, which was consistent with some existing Chinese and international literature reports (23). It was suggested that TNF-β, IL-2, IL-4, IL-7, IL-9, and PCT play key roles in MP infection. Similar to bacterial or viral infection, MP infection can also stimulate a variety of immunoactive cells to produce cytokines, resulting in body damage. Moreover, this study also showed that 36 of the 40 children with pleural effusion had pulmonary fibrosis according to chest X-ray examination, and the concentrations of TNF-α and IL-8 in pleural effusion were significantly higher than those in the children without pulmonary fibrosis (P<0.05). There were statistically significant differences in TNF-α, IL-8, and PCT in pleural effusion samples of children with pulmonary fibrosis compared with serum samples (P<0.05). The concentrations of TNF-α, IL-6, IL-8, IL-10, and PCT in pleural effusion patients without pulmonary fibrosis were not significantly different from those in serum (P>0.05). This confirms that TNF-α, IL-6, IL-8, IL-10, and PCT play an important role in the formation of pulmonary fibrosis in children with MPP, which is consistent with relevant reports from China and globally (24,25).
The multi-dominant cytokine TNF-α is produced by mononuclear macrophages. It is a biologically active inflammatory mediator with physiological functions such as regulating immune response and promoting cell growth and differentiation. Its biological activity is transmitted through specific receptors on the cell surface. In general, at low concentrations in the body, MP infection can induce macrophages to produce TNF-α in vivo. It not only increases inflammatory response, but also collaborates with infection to produce mediators to stimulate lymphocytes and mononuclear macrophages to produce excess of other cytokines and release them into the blood, including increased serum levels of TNF-α, IL-6, IL-8, IL-10, and PCT (26). The results of this study showed that serum TNF-α concentration in children with MPP in the acute stage was significantly higher than that in those in the convalescent stage and control group (P<0.05), indicating that TNF-α plays an important role in MPP. This is mainly because MP infection stimulates lung macrophages to release a large amount of TNF-α, resulting in lung injury, and at the same time, the injection into the blood leads to the damage of the extrapulmonary tissues. This also indicates that the determination of serum TNF-α concentration can reflect the severity of the disease, which is of great significance to judge the disease and guide clinical treatment. The cytokine IL-6 can be produced spontaneously by mononuclear macrophages, endothelial cells, lymphocytes, and so on, or under the stimulation of a variety of factors, and can also be produced by various cells induced by TNF-α and IL-1. It is a very important non-specific inflammatory factor in MP-infected diseases, and it participates in the regulation of the pathological process of pulmonary inflammatory response, mainly by inducing the growth and differentiation of immune cells such as T cells and B cells, and the secretion of immunoglobulin (27). The results of this study showed that the concentration of IL-6 decreased in MPP children during recovery, which was higher than that in the control group, but the difference was not statistically significant (P>0.05). According to the conclusion that IL-6 only increased in the acute phase and returned to normal in the recovery period, it was interpreted that the excessive secretion of IL-6 in the acute phase of some children leads to excessive production of autoantibodies in children, but the excessive production of IL-6 is not continuous. The recovery period can be reduced, as can the corresponding autoantibodies. This field is different from chronic and recurrent autoimmune diseases.
As a multi-source inflammatory cytokine, IL-8 is mainly derived from monocyte macrophages, T lymphocytes, vascular endothelial cells, fibroblasts, and so on, and it is a chemokine of neutrophils. The main biological function of IL-8 is chemotaxis and activation of neutrophils, and degranulation and release of superoxide and lysosomal enzymes. Studies have shown that the infiltrating cells of MPP are mainly monocyte-macrophages, lymphocytes, and neutrophils (28-30). After MP enters the body, monocytes appear on the surface of the respiratory tract, which results in complications of varying degrees other than lung disease, suggesting that airway epithelial cells and alveolar macrophages produce a large number of inflammatory mediators, such as IL-8, which were released into the blood, leading to various organ/tissue injuries (31). In this study, chest X-ray and CT examination showed that IL-8 levels in serum and pleural effusion in children with pulmonary fibrosis were significantly higher than those in children without fibrosis, further suggesting that IL-8, as an inflammatory mediator, is related to a variety of inflammatory mechanisms and plays an important role in the occurrence and development of MP. In recent years, the extensive immune effects of the homologous dimer somatic factor IL-10 have been discovered. It can be produced by monocyte-macrophages, mast cells, B cells, T cells, and dendritic cells, and belongs to a long chain of cytokines family. The basic function of IL-10 is to reduce the production of cytokines by TH1 cells, and it is an immunomodulatory molecule with immunomodulatory, immunosuppressive, and anti-inflammatory activities (32). Therefore, it is also called a cytokine synthesis inhibitory factor (CSIF). This study detected the changes of serum IL-10 concentration in children with MPP, which can provide a reliable theoretical basis for clinical diagnosis and treatment. At the same time, PCT is a new inflammatory marker discovered by Lafarga et al. (33) proposed that serum PCT can reflect the inflammatory index of bacterial infection. The concentration of PCT in serum of healthy people is very low, and PCT can be detected in plasma of healthy people after intravenous injection of low-dose endotoxin for 2 hours, and then concentration continues to rise. The peak value was reached at 12 to 120 hours, and recovered to normal 2 to 3 days later. The PCT levels increased with the progression of the disease. However, PCT levels decrease rapidly after treatment and can be used as an indicator of bacterial infection (34).
The results of this study indicate that in children with MPP, the detection of PCT level can indicate the presence of bacterial infection and the severity of infection, which has a reference role in guiding the selection and adjustment of antibacterial drugs and reflecting the effectiveness of antibacterial drugs.
Conclusions
In general, the onset of MPP is slow, and the pathological changes are mainly interstitial pneumonia and acute bronchiolitis. The main clinical manifestation is cough, and the symptoms of infection and poisoning are light, the signs are few, hypoxia and dyspnea are not obvious, and the clinical application of antibiotics is not satisfactory. Some cases became complicated with pleural effusion, and chest X-ray and CT examination suggested pulmonary fibrosis or extrapulmonary complications. Due to the existence of some common antigens between MP and some human tissues, autoantibodies of corresponding tissues could be formed after infection, resulting in impaired immune functions of multiple systems. It is generally believed that in the early stage of infection, the serum concentrations of TNF-α, IL-6, IL-8, IL-10, and PCT in children with pneumonia are increased due to inflammatory stimulation, but with the improvement and cure of the disease, the concentrations are significantly reduced or close to normal. The peak duration of TNF-α and IL-8 was shorter than that of IL-6 and IL-10, and PCT. Although they had increased in the early stage, they soon decreased or approached normal. In recent years, studies have confirmed that cellular immunity plays an important role in the pathogenesis of MP, especially cytokines such as TNF-α, IL-6, IL-8, IL-10, and PCT as well as inflammatory mediators. It is currently believed that the higher the concentration of TNF-α, IL-6, IL-8, IL-10, and PCT in the serum of children infected with MP, the more serious the development of the disease, and the degree of elevation is related to the severity and course of the disease.
Acknowledgments
Funding: None.
Footnote
Reporting Checklist: The authors have completed the MDAR reporting checklist. Available at https://tp.amegroups.com/article/view/10.21037/tp-22-416/rc
Data Sharing Statement: Available at https://tp.amegroups.com/article/view/10.21037/tp-22-416/dss
Conflicts of Interest: All authors have completed the ICMJE uniform disclosure form (available at https://tp.amegroups.com/article/view/10.21037/tp-22-416/coif). The authors have no conflicts of interest to declare.
Ethical Statement: The authors are accountable for all aspects of the work in ensuring that questions related to the accuracy or integrity of any part of the work are appropriately investigated and resolved. The study was conducted in accordance with the Declaration of Helsinki (as revised in 2013). The study was approved by the Research Ethics Committee of the Third Affiliated Hospital of Jinzhou Medical University (No. KX2022021). The patients in this study and their families fully understood the situation and signed the informed consent.
Open Access Statement: This is an Open Access article distributed in accordance with the Creative Commons Attribution-NonCommercial-NoDerivs 4.0 International License (CC BY-NC-ND 4.0), which permits the non-commercial replication and distribution of the article with the strict proviso that no changes or edits are made and the original work is properly cited (including links to both the formal publication through the relevant DOI and the license). See: https://creativecommons.org/licenses/by-nc-nd/4.0/.
References
- Fernández-Huerta M, Barberá MJ, Serra-Pladevall J, et al. Mycoplasma genitalium and antimicrobial resistance in Europe: a comprehensive review. Int J STD AIDS 2020;31:190-7. [Crossref] [PubMed]
- Kumar S, Roy RD, Sethi GR, et al. Mycoplasma pneumoniae infection and asthma in children. Trop Doct 2019;49:117-9. [Crossref] [PubMed]
- Kumar S. Mycoplasma pneumoniae: A significant but underrated pathogen in paediatric community-acquired lower respiratory tract infections. Indian J Med Res 2018;147:23-31. [Crossref] [PubMed]
- Leli C, Mencacci A, Latino MA, et al. Prevalence of cervical colonization by Ureaplasma parvum, Ureaplasma urealyticum, Mycoplasma hominis and Mycoplasma genitalium in childbearing age women by a commercially available multiplex real-time PCR: An Italian observational multicentre study. J Microbiol Immunol Infect 2018;51:220-5. [Crossref] [PubMed]
- Wan Q, Liu X, Zeng Z, et al. Aptamer Cocktail to Detect Multiple Species of Mycoplasma in Cell Culture. Int J Mol Sci 2020;21:3784. [Crossref] [PubMed]
- Poddighe D. Mycoplasma pneumoniae-related extra-pulmonary diseases and antimicrobial therapy. J Microbiol Immunol Infect 2020;53:188-9. [Crossref] [PubMed]
- Jambhekar A, Robin E, Le Boedec K. A systematic review and meta-analyses of the association between 4 mycoplasma species and lower respiratory tract disease in dogs. J Vet Intern Med 2019;33:1880-91. [Crossref] [PubMed]
- Lee H, Yun KW, Lee HJ, et al. Antimicrobial therapy of macrolide-resistant Mycoplasma pneumoniae pneumonia in children. Expert Rev Anti Infect Ther 2018;16:23-34. [Crossref] [PubMed]
- Shah SS. Mycoplasma pneumoniae as a Cause of Community-Acquired Pneumonia in Children. Clin Infect Dis 2019;68:13-4. [PubMed]
- Volokhov DV, Grózner D, Gyuranecz M, et al. Mycoplasma anserisalpingitidis sp. nov., isolated from European domestic geese (Anser anser domesticus) with reproductive pathology. Int J Syst Evol Microbiol 2020;70:2369-81. [Crossref] [PubMed]
- Zhou Y, Wang J, Chen W, et al. Impact of viral coinfection and macrolide-resistant mycoplasma infection in children with refractory Mycoplasma pneumoniae pneumonia. BMC Infect Dis 2020;20:633. [Crossref] [PubMed]
- Liu J, He R, Wu R, et al. Mycoplasma pneumoniae pneumonia associated thrombosis at Beijing Children’s hospital. BMC Infect Dis 2020;20:51. [Crossref] [PubMed]
- Søndergaard MJ, Friis MB, Hansen DS, et al. Clinical manifestations in infants and children with Mycoplasma pneumoniae infection. PLoS One 2018;13:e0195288. [Crossref] [PubMed]
- He J, Liu M, Ye Z, et al. [Corrigendum] Insights into the pathogenesis of Mycoplasma pneumoniae Mol Med Rep 2018;17:4155. (Review). [PubMed]
- Kenri T, Kawakita Y, Kudo H, et al. Production and characterization of recombinant P1 adhesin essential for adhesion, gliding, and antigenic variation in the human pathogenic bacterium, Mycoplasma pneumoniae. Biochem Biophys Res Commun 2019;508:1050-5. [Crossref] [PubMed]
- Launes J, Paetau A, Linnavuori K, et al. Direct invasion of the brain parenchyma by Mycoplasma pneumoniae. Acta Neurol Scand 1997;95:374. [Crossref] [PubMed]
- Hagemann L, Gründel A, Jacobs E, et al. The surface-displayed chaperones GroEL and DnaK of Mycoplasma pneumoniae interact with human plasminogen and components of the extracellular matrix. Pathog Dis 2017; [Crossref] [PubMed]
- Fan H, Lu B, Yang D, et al. Distribution and Expression of IL-17 and Related Cytokines in Children with Mycoplasma pneumoniae Pneumonia. Jpn J Infect Dis 2019;72:387-93. [Crossref] [PubMed]
- Wang Y, Li H, Shi Y, et al. miR-143-3p impacts on pulmonary inflammatory factors and cell apoptosis in mice with mycoplasmal pneumonia by regulating TLR4/MyD88/NF-κB pathway. Biosci Rep 2020;40:BSR20193419. [Crossref] [PubMed]
- Yoon SH, Min IK, Ahn JG. Immunochromatography for the diagnosis of Mycoplasma pneumoniae infection: A systematic review and meta-analysis. PLoS One 2020;15:e0230338. [Crossref] [PubMed]
- Ning G, Wang X, Wu D, et al. The etiology of community-acquired pneumonia among children under 5 years of age in mainland China, 2001-2015: A systematic review. Hum Vaccin Immunother 2017;13:2742-50. [Crossref] [PubMed]
- Kawakami N, Namkoong H, Saito F, et al. Epidemiology of macrolide-resistant Mycoplasma pneumoniae by age distribution in Japan. J Infect Chemother 2021;27:45-8. [Crossref] [PubMed]
- Fourour S, Marois-Créhan C, Martelet L, et al. Intra-Species and Inter-Species Differences in Cytokine Production by Porcine Antigen-Presenting Cells Stimulated by Mycoplasma hyopneumoniae, M. hyorhinis, and M. flocculare. Pathogens 2019;8:34. [Crossref] [PubMed]
- Wang H, Zhou Q, Dai W, et al. Lung Microbiota and Pulmonary Inflammatory Cytokines Expression Vary in Children With Tracheomalacia and Adenoviral or Mycoplasma pneumoniae Pneumonia. Front Pediatr 2019;7:265. [Crossref] [PubMed]
- Remmelts HH, Meijvis SC, Biesma DH, et al. Dexamethasone downregulates the systemic cytokine response in patients with community-acquired pneumonia. Clin Vaccine Immunol 2012;19:1532-8. [Crossref] [PubMed]
- Li G, Fan L, Wang Y, et al. High co-expression of TNF-α and CARDS toxin is a good predictor for refractory Mycoplasma pneumoniae pneumonia. Mol Med 2019;25:38. [Crossref] [PubMed]
- Tian F, Chen LP, Yuan G, et al. Differences of TNF-α, IL-6 and Gal-3 in lobar pneumonia and bronchial pneumonia caused by mycoplasma pneumoniae. Technol Health Care 2020;28:711-9. [Crossref] [PubMed]
- Huang H, Gao Y, Nie K, et al. Macrophage migration inhibitory factor meditates MPP+/MPTP-induced NLRP3 inflammasome activation in microglia cells. Nan Fang Yi Ke Da Xue Xue Bao 2021;41:972-9. [PubMed]
- Li N, Mu YP, Chen J, et al. Value of absolute counts of lymphocyte subsets in the early prediction of refractory Mycoplasma pneumoniae pneumonia in children. Zhongguo Dang Dai Er Ke Za Zhi 2019;21:511-6. [PubMed]
- Chen Z, Shao X, Dou X, et al. Role of the Mycoplasma pneumoniae/Interleukin-8/Neutrophil Axis in the Pathogenesis of Pneumonia. PLoS One 2016;11:e0146377. [Crossref] [PubMed]
- Lee KE, Kim KW, Hong JY, et al. Modulation of IL-8 boosted by Mycoplasma pneumoniae lysate in human airway epithelial cells. J Clin Immunol 2013;33:1117-25. [Crossref] [PubMed]
- Ding S, Wang X, Chen W, et al. Decreased Interleukin-10 Responses in Children with Severe Mycoplasma pneumoniae Pneumonia. PLoS One 2016;11:e0146397. [Crossref] [PubMed]
- Lafarga MA, Ezquerra J, Ferrández A, et al. Phage count in the waters of the Canal Imperial de Aragón and the Ebro River in Saragossa. Microbiologia 1993;9:43-52. [PubMed]
- Chen P, Huang Z, Chen L, et al. The relationships between LncRNA NNT-AS1, CRP, PCT and their interactions and the refractory mycoplasma pneumoniae pneumonia in children. Sci Rep 2021;11:2059. [Crossref] [PubMed]
(English Language Editor: J. Jones)