Sivelestat improves clinical outcomes and decreases ventilator-associated lung injury in children with acute respiratory distress syndrome: a retrospective cohort study
Introduction
Acute respiratory distress syndrome (ARDS) has high morbidity and mortality rates in critically ill patients (1). An international prospective study of 145 pediatric intensive care units in 27 countries reported that the mortality rate of pediatric patients with severe ARDS was 33% (2). ARDS has also been reported to be a major cause of death among coronavirus disease 2019 (COVID-19) patients (3,4).
Due to a lack of consensus on the clinical diagnostic criteria for pediatric ARDS (PARDS), pediatricians often refer to adult diagnostic criteria when diagnosing pediatric patients (5-7). In 2015, the concept of PARDS was defined and diagnostic criteria were issued by the Pediatric Acute Lung Injury Consensus Conference (PALICC) (8,9). Due to differences in the pathophysiology, etiology, and treatment of ARDS between children and adults, the PALICC definition may be more appropriate than the previous Berlin definitions in terms of pediatric morbidity and prognosis (10-13). Further, as the PALICC definition is partially based on the Berlin definition, the pathobiology and practice patterns of PARDS are considered similar to those of adults (14,15).
PARDS has multiple etiologies, including infection, trauma, chemical poison inhalation, and other serious clinical conditions (16,17); however, infection (including pneumonia and sepsis), which can cause dysfunction (18), remains the leading cause of PARDS (2,13). Treatment measures for ARDS may be individualized and include prone ventilation, lung-protective ventilation (LPV), low tidal volume (VT) high-frequency ventilation, and neuromuscular blocking agents (19). The standardized implementation of these measures can benefit patients and improve outcomes. Due to a lack of targeted drugs to treat ARDS-associated lung inflammation, some patients experience the continuous aggravation of lung inflammation, which eventually progresses into irreversible lung injury. The severity of the pulmonary inflammatory response is associated with disease mortality, intensive care unit (ICU) stay time, and ventilator-free days (20).
In a randomized cohort study (21), patients with ARDS were divided into hypo-inflammatory sub-phenotype and hyper-inflammatory sub-phenotype groups. The hyper-inflammatory sub-phenotype was associated with increased inflammatory biomarkers, a higher prevalence of shock, and worse clinical outcomes, and the overexpression of interleukin (IL)-6 and IL-8 (14). This hyper-inflammatory response is positively correlated with the severity of ARDS, and multiple studies have reported similar findings (22-24). The chemotactic effect of IL-8 results in leukocyte aggregation in the lungs and respiratory distress leads to a chain of pathophysiological changes and ultimately causes ARDS (25). Reducing the aggregation of leukocytes in the lungs can reduce the incidence of acute lung injury, and the severity of the inflammatory response (26).
Neutrophil elastase is a neutrophil-specific serine protease secreted from the primary granules and plays an important role in inflammation (27,28). In physiological conditions, elastase helps remove bacteria, cleans up damaged tissue, and promotes tissue regeneration (29). However, the overexpression of neutrophil elastase, which is a protease enzyme, is one of the main factors of lung consolidation and dysfunctional oxygenation in ARDS patients. Sivelestat, a neutrophil elastase inhibitor, reduces elastase activation and inhibits neutrophil aggregation by reducing the inflammatory response and the concentrations of IL-8 and tumor necrosis factor-α (TNF-α) (29-31). Numerous studies have concluded that sivelestat is therapeutically effective in treating ARDS, organ transplantation, tumors, and trauma (29,30,32). High neutrophil ratios have been found to be associated with increased disease severity and mortality in critically ill COVID-19 patients (33). Several studies have shown that sivelestat reduces mortality and the incidence of ventilator-induced lung injury (VILI) associated with COVID-19 (31,33,34). Sivelestat is generally used in adult patients, but it has been widely used in pediatric cases as well. Meanwhile, few adverse events have been reported in children (35-37).
In the present study, we hypothesized that neutrophil elastase inhibitors, such as sivelestat, not only reduce lung injury by inhibiting neutrophil elastase activity, but also inhibit localized neutrophil proliferation by reducing the inflammatory response and IL-8 concentration and improving the prognosis of pediatric patients with ARDS. We present the following article in accordance with the STROBE reporting checklist (available at https://tp.amegroups.com/article/view/10.21037/tp-22-441/rc).
Methods
Patients were enrolled in this retrospective study if they were aged 28 days to 18 years old and met the diagnostic criteria for PARDS. Patients were excluded if they met any of the following exclusion criteria: (I) were a newborn (aged <28 days) or were aged >18 years; (II) had abnormal circulatory perfusion due to cardiogenic diseases; (III) had a congenital organic acid metabolism disorder; (IV) had an immunodeficiency disease; and/or (V) had undergone chemotherapy or immunosupportive therapy. All the pediatric patients were hospitalized in the Intensive Care Department at the Affiliated Children’s Hospital of Xi’an Jiaotong University, in Shaanxi, China. The study was conducted in accordance with the Declaration of Helsinki (as revised in 2013). The study was approved by the Ethics Committee of the Affiliated Children’s Hospital of Xi’an Jiaotong University (No. 20220081), and informed consent was taken from all the patients’ guardians.
The control group data were collected from the Hospital’s Electronic Case Information System between March 2017 to January 2020 for patients clinically diagnosed with PARDS. The case group data were also collected for patients who received sivelestat treatment; these patients were observed between February 2020 to February 2022. In order to reduce systematic bias due to background factors, we only included patients with ARDS associated with community-acquired pneumonia, and there were no significant differences in demographic and basic clinical data characteristics between two groups. Meanwhile, we conducted a subgroup analysis of some basic variables in different age groups to make our study more rigorous.
For new PARDS cases, the diagnostic criteria for PARDS had to be met within 24 h of admission. Patients were eligible for inclusion in this study if they had been newly diagnosed with PARDS during the study week. Patients were diagnosed with PARDs if they met the following PALICC criteria: (I) developed hypoxemia within 7 days of a clinical insult; (II) had respiratory failure that could not be fully explained by fluid overload or cardiac failure; (III) had chest imaging scans that revealed new infiltrates or infiltrates consistent with pulmonary parenchymal disease; and (IV) had minimal hypoxemia that required respiratory support by mechanical ventilation.
The main safety outcomes were the ratio of partial arterial pressure of oxygen (PaO2) to fractional concentration of inspired oxygen (FiO2; i.e., the PaO2/FiO2 ratio) and the ratio of blood oxygen saturation to FiO2 (SF ratio). Respiratory support by mechanical ventilation was as follows: (I) for non-invasively ventilated patients, the PaO2/FiO2 ratio is ≤300, or the SF ratio is ≤264 with a full-face mask or nasal mask and continuous positive airway pressure or bilevel positive airway pressure ≥5 cmH2O; and (II) for invasively ventilated patients, the oxygenation index is ≥4 or oxygenation saturation index is ≥5. For patients receiving invasive mechanical ventilation, hypoxemia was determined using the PALICC oxygenation index or oxygenation saturation index severity groups. Severe hypoxemia is an oxygenation index of ≥16, or an oxygenation saturation index of ≥12.3. For non-invasively ventilated patients, the PALICC definition does not stratify hypoxemia severity; thus, we used the Berlin definition where severe hypoxemia in non-invasively ventilated patients is a PaO2/FiO2 ratio of ≤100 or an SF ratio of ≤150. Additional outcomes of interest were changes of inflammatory mediators, lung static compliance and mortality.
All the patients were clinically managed according to standard PALICC PARDS criteria, and received treatment measures, such as prone ventilation, LPV strategy, low VT high-frequency ventilation, and neuromuscular blocking agents.
We collected the demographic data of all the patients, etiological cultures, their pediatric Sequential Organ Failure Assessment score (38), the Murray score, their PaO2/FiO2 ratio, central venous pressure, arterial oxygen saturation (SaO2), mean arterial pressure, and details of the primary disease.
All the patients were examined within 1 hour of admission for C-reactive protein, routine blood tests, procalcitonin, and IL-8; these were also reviewed at 12-hour intervals. Ventilator parameters were adjusted according to the SaO2, and we conducted a comprehensive evaluation every 8 h, and recorded the results. The primary outcome measure was 28-day mortality. The secondary outcomes included the number of ventilator-free days, extracorporeal membrane oxygenation assistance rate, and ICU stay time.
Statistical analysis
The data were analyzed using SPSS software (version 21.0). The Chi-square test was used for the categorical variables. The continuous variables with normal distribution are presented as the means ± standard deviations, and comparisons between the 2 groups were performed using the independent sample t-test. The continuous variables with abnormal distribution are presented as the medians [interquartile ranges (IQRs)]. The 28-day cumulative survival rate was evaluated using the Kaplan-Meier and log-rank test. Statistical significance was defined at P<0.05.
Results
Comparative analysis of the baseline data
In total, 212 children with PARDS were enrolled in the study, of whom 125 were allocated to the case group and received the sivelestat treatment, and 87 were allocated to the control group. There were no statistically significant differences in the general demographic and clinical characteristics between the case group and the control group (Table 1), nor in clinical characteristics and ventilator parameters (Table 2).
Table 1
Variables | Sivelestat group | Control group | P value |
---|---|---|---|
Patients | 125 | 87 | |
Gender (male/female) | 67/58 | 42/45 | 0.445 |
Age (years) | 6.3 [0.4, 8.9] | 5.8 [0.3, 9.1] | 0.521 |
Weight (kg) | 18 [8, 31] | 20 [10, 37] | 0.433 |
Classification of pathogenic | |||
Gram-negative bacteria | 14 | 6 | 0.759 |
Gram-positive bacteria | 11 | 8 | 0.932 |
Virus infection | 22 | 14 | 0.990 |
Others | 17 | 11 | 0.840 |
Primary disease | |||
Sepsis | 37 | 26 | 0.964 |
Inhalation pulmonary injury | 18 | 12 | 0.901 |
Trauma | 11 | 9 | 0.705 |
Hemorrhagic fever with renal syndrome | 31 | 23 | 0.788 |
Severe acute pancreatitis | 11 | 6 | 0.616 |
Others | 16 | 9 | 0.586 |
Data presented as median [Q1, Q3] or N.
Table 2
Variables | Sivelestat group | Control group | P value |
---|---|---|---|
Patients | 125 | 87 | |
Clinical indicators characteristics | |||
pSOFA score | 9±8 | 10±6 | 0.324 |
Murry score | 3.65±0.31 | 3.71±0.25 | 0.236 |
PaO2/FiO2 (mmHg) | 159±39 | 171±42 | 0.594 |
SaO2 (%) | 78±24 | 83±14 | 0.081 |
CVP (mmHg) | 11±4 | 12±5 | 0.108 |
MAP (mmHg) | 50±17 | 52±13 | 0.356 |
Ventilator parameters characteristics | |||
FiO2 (%) | 75±21 | 72±24 | 0.200 |
PEEP (cmH2O) | 13±3 | 12±5 | 0.081 |
Ppeak (cmH2O) | 35±5 | 36±4 | 0.122 |
Pplat (cmH2O) | 31±4 | 30±5 | 0.108 |
Pdrive (cmH2O) | 18±3 | 19±4 | 0.122 |
Data presented as mean ± SD or N. pSOFA, pediatric sequential organ failure assessment; Murry score, acute lung injury score; PaO2/FiO2, arterial oxygen partial pressure/fraction of inspired oxygen; SaO2, arterial oxygen saturation; CVP, central venous pressure; MAP, mean arterial pressure; PEEP, positive end expiratory pressure; Ppeak, peak pressure; Pplat, plateau pressure; Pdrive, drive pressure; SD, standard deviation.
PaO2/FiO2, SaO2, and Cst
The severity of ARDS was determined by PaO2/FiO2. There were no significant differences in PaO2/FiO2 (Figure 1A) between the 2 groups at 24 h post-admission, or in SaO2 (see Figure 1B). The case group had higher PaO2/FiO2 and SaO2 than the control group at 48 h (89±6 vs. 85±4; P=0.014), and 72 h (92±6 vs. 88±8; P<0.001). The children included in the study were stratified by age to compare the lung static compliance (Cst) at different time points between the 2 groups in the different age subgroups. There was no significant difference in the changes of Cst among each subgroup at 24 h post-admission. Across the different age subgroups, the lung Cst of the case group was significantly higher than that of the control group at 48 h post-admission (up to 3 years of age: 14±2 vs. 10±2; P=0.013; Figure 2A; 3–6 years of age, 19±3 vs. 15±2; P=0.007; Figure 2B; 6+ years of age: 49±5 vs. 36±4; P<0.001; Figure 2C), and 72 h post-admission (up to 3 years of age: 15±4 vs. 12±3; P=0.021; 3–6 years of age: 25±5 vs. 17±4; P<0.001; 6+ years of age: 49±5 vs. 36±4; P<0.001).
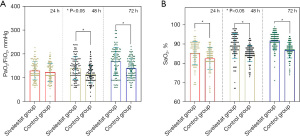
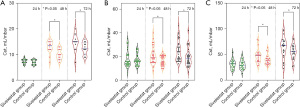
FiO2, Ppeak, Pplat, and Pdrive
Compared to the control group, the case group displayed decreased FiO2 at 16 h (55±12 vs. 68±20; P<0.001), 24 h (50±16 vs. 65±18; P<0.001), 48 h (50±5 vs. 58±16; P<0.001), and 72 h (46±7 vs. 55±15; P<0.001) post-admission (see Figure 3A). The airway peak pressure (Ppeak) of the case group was lower than that of the control group at 16 h (32±6 vs. 38±7; P<0.001), 24 h (32±4 vs. 37±6; P<0.001), 48 h (30±5 vs. 35±6; P<0.001), and 72 h (28±2 vs. 32±7; P<0.001) post-admission (see Figure 3B). There were significant differences in plateau pressure (Pplat) between the 2 groups. The Pplat of the case group was lower than that of the control group at 24 h (24±3 vs. 28±7; P<0.001), 48 h (21±4 vs. 26±7; P<0.001), and 72 h (20±2 vs. 25±6; P<0.001) post-admission (see Figure 3C). The drive pressure (Pdrive) of the case group was lower (see Figure 3D) than that of the control group at 16 h (15±2 vs. 20±2; P<0.001), 24 h (13±5 vs. 18±3; P<0.001), 48 h (12±4 vs. 18±5; P<0.001), and 72 h (12±73 vs. 16±4; P<0.001) post-admission.
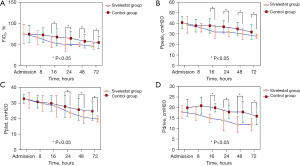
Leucocyte counts, C-reactive protein, procalcitonin, IL-8, and mortality
Comparisons of the indicators related to inflammatory response between case group and control group. This study showed that leucocyte counts, C-reactive protein, and procalcitonin in two groups were 8±3 vs. 10±4, 20±14 vs. 32±20, 20±12 vs. 27±14, all P<0.05 at 48 h; and 7±3 vs. 9±3, 23±20 vs. 27±14, 26±19 vs. 40±20, all P<0.05 at 72 h post-admission, respectively (see Figures 4A-4C). Additionally, the IL-8 of the case group was lower than that of the control group at 48 and 72 h post-admission (all P<0.05; see Figure 5A). The IL-8 of the deceased group was higher than that of the survival group at 24, 48, and 72 h, and the difference between the 2 groups was significant (all P<0.05; see Figure 5B). The overall 28-day mortality rate was 25.47% (54/212); 25 children died in the case group (n=125), and 29 children died in the control group (n=87; Table 3). The survival analysis showed that the cumulative survival of the case group was higher than that of the control group (log-rank test: χ2=4.811; P=0.028; see Figure 6).
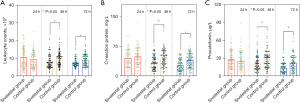
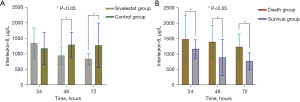
Table 3
Variables | Sivelestat group | Control group | P value |
---|---|---|---|
Mortality | 25 | 29 | 0.028 |
Free ventilator days | 11±4 | 8±4 | 0.000 |
Barotrauma | 31 | 42 | 0.000 |
ICU stay time, day | 26±9 | 32±13 | 0.000 |
ECMO supported | 12 | 9 | 0.121 |
Data presented as mean ± SD or N. ICU, intensive care unit; ECMO, extracorporeal membrane oxygenation; SD, standard deviation.
Discussion
ARDS represents a serious threat to human health. In addition to being a medical problem, with the progress of COVID-19, ARDS has become a public health problem on a global scale. The main clinical manifestations of ARDS are high levels of neutrophil infiltration in the interstitium and alveoli, resulting in interstitium and alveolar edema, hyperemia, alveolar hyaline membrane formation, and alveolar atrophy, causing an imbalance of pulmonary ventilation and the blood flow ratio, leading to refractory hypoxemia (31).
Persistent hypoxemia and severe lung consolidation make it difficult to effectively treat the disease. A recent study has found that elastase released by neutrophils participate in the degradation of major components of the extracellular matrix, such as elastin, type IV collagen, and proteoglycan, a process closely associated with lung injury (31). Mechanically assisted ventilation can improve patients’ oxygenation state; however, unreasonable parameters can cause a multitude of complications, and even patients death.
In ARDS patients, the risk of superimposed VILI merits LPV strategies (31,39). Low VT, optimal positive end expiratory pressure (PEEP), and low Pplat are important concerns that can be addressed with LPV strategies (39-41). Lower VT, optimal PEEP, and lower Pplat can prevent frank overinflation (volutrauma/barotrauma), decrease tide-induced mechanical strain in heterogeneous lung regions, reduce intrapulmonary shunt and the collapse of small airways and alveoli (atelectrauma) (8), and reduce cellular and extracellular matrix injury (11). Some multicenter trials have demonstrated that LPV strategies improve the survival of patients with ARDS (42-44).
In our study, the patients in the case group showed significant improvements in terms of the ventilator parameters after 48 h of medication treatment, and these improvements were correlated with the duration of medication. In addition, with the extension of medication time, the airway Ppeak, Pplat, and Pdrive of patients in the case group decreased significantly, compared to those of the control group, especially at 48 and 72 h post-admission. Pulmonary Cst was significantly improved across different age groups compared to the control group with the change of medication duration. Thus, sivelestat appears to reduce lung consolidation and improve lung tissue compliance and oxygenation by reducing neutrophil elastase activity. As lung compliance improves, the body becomes less dependent on mechanical ventilation, resulting in a decline in the ventilator parameters.
Most patients required a high concentration of oxygen to maintain adequate oxygenation. Previous cohort studies and animal studies have found that the higher the concentration of oxygen inhaled, the more serious the alveolar damage (45). Additionally, research has shown that the longer the duration for which oxygen is inhaled, the greater the degree of irreversible lung damage (39,41). In the case group, FiO2 decreased significantly compared to the control group, thereby reducing the risk of hyperoxia and oxygen toxicity. Based on these observations, we suggest that sivelestat reduces the degree of damage to alveolar and interstitial lesions and improves ventilation/oxygenation status. In our study, the variation difference of PaO2/FiO2 between the 2 groups also indirectly supported these results. According to a previous study (20), sivelestat treatment might improve PaO2/FiO2 to some extent, which could help to better determine the efficacy of sivelestat in ARDS patients and help physicians select appropriate treatment strategies. Thus, we concluded that when medical intervention factors change the alveolar/interstitial lesions of patients and improve the ventilation/blood flow imbalance, the body’s dependence on mechanical ventilation is gradually reduced, and the risk of hyperoxia and VILI can be reduced or prevented.
By inhibiting neutrophil aggregation, adhesion, and infiltration, sivelestat reduces pulmonary exudation and pulmonary edema. Sivelestat has been shown to inhibit the release of inflammatory mediators, such as IL-8 and TNF-α, thereby suppressing the inflammatory response and improving lung injury symptoms (46,47). In our study, IL-8 concentration was significantly more reduced in the patients who received sivelestat than those in the control group, and it was also higher in the survival group at different time points than in the deceased group. These results are consistent with previous studies, and effectively prove that the severity of lung injury and the prognosis of ARDS are related to the overexpression of IL-8.
A multicenter clinical study in Japan showed that patients who received sivelestat treatment had shorter mechanical ventilation requirements than those who did not, and the 180-day survival rate was significantly increased (48). This is also consistent with our findings. The findings of the present study suggest that sivelestat therapy has a significant effect on 28-day mortality. The survival analysis showed that the cumulative survival of the case group was higher than that of the control group. However, almost all previous trials reported a reduction in 28-day mortality, albeit the reduction was not statistically significant (20). This may be because the effect of sivelestat on 28-day mortality is affected by specific clinical conditions, such as age, disease status, hemodialysis, and methylprednisolone use (49).
The present study had some limitations. First, it was a non-double-blind, observational, retrospective cohort study with a large time span; thus the period/duration of data collection may not be sufficient. Longer observation periods may be required. Second, it was a single-center study with relatively few patients, which may have affected the results. Finally, the precise design of targeted therapy is needed for drug effectiveness studies, and future multicenter studies with larger sample sizes need to be conducted to further confirm our findings.
Conclusions
In addition to reducing the release pulmonary inflammatory mediators in ARDS patients, neutrophil elastase inhibitors, such as sivelestat, also reduce the titer and activity of neutrophil elastase. Further, sivelestat is a targeted therapy that can change the imbalance of ventilation/blood flow ratio, thereby improving the prognosis of ARDS patients.
Acknowledgments
Funding: This work was supported by the Natural Science Basic Research Plan in Shaanxi Province of China (No. 2021JM-560), the Medical Research Program of the Xi’an Science and Technology Bureau (No. 21YXYJ0009), and the Xi’an Children’s Hospital Scientific Research Project (No. 2020C15).
Footnote
Reporting Checklist: The authors have completed the STROBE reporting checklist. Available at https://tp.amegroups.com/article/view/10.21037/tp-22-441/rc
Data Sharing Statement: Available at https://tp.amegroups.com/article/view/10.21037/tp-22-441/dss
Conflicts of Interest: All authors have completed the ICMJE uniform disclosure form (available at https://tp.amegroups.com/article/view/10.21037/tp-22-441/coif). The authors have no conflicts of interest to declare.
Ethical Statement: The authors are accountable for all aspects of the work in ensuring that questions related to the accuracy or integrity of any part of the work are appropriately investigated and resolved. The study was conducted in accordance with the Declaration of Helsinki (as revised in 2013). The research protocol was approved by the Ethics Committee of the Affiliated Children’s Hospital of Xi’an Jiaotong University (No. 20220081), and the patients’ guardians provided written informed consent.
Open Access Statement: This is an Open Access article distributed in accordance with the Creative Commons Attribution-NonCommercial-NoDerivs 4.0 International License (CC BY-NC-ND 4.0), which permits the non-commercial replication and distribution of the article with the strict proviso that no changes or edits are made and the original work is properly cited (including links to both the formal publication through the relevant DOI and the license). See: https://creativecommons.org/licenses/by-nc-nd/4.0/.
References
- Schouten LR, Veltkamp F, Bos AP, et al. Incidence and Mortality of Acute Respiratory Distress Syndrome in Children: A Systematic Review and Meta-Analysis. Crit Care Med 2016;44:819-29. [Crossref] [PubMed]
- Khemani RG, Smith L, Lopez-Fernandez YM, et al. Paediatric acute respiratory distress syndrome incidence and epidemiology (PARDIE): an international, observational study. Lancet Respir Med 2019;7:115-28. [Crossref] [PubMed]
- Cao W, Fang Z, Hou G, et al. The psychological impact of the COVID-19 epidemic on college students in China. Psychiatry Research 2020; [Crossref] [PubMed]
- Yang X, Yu Y, Xu J, et al. Clinical course and outcomes of critically ill patients with SARS-CoV-2 pneumonia in Wuhan, China: a single-centered, retrospective, observational study. Lancet Respir Med 2020;8:475-81. [Crossref] [PubMed]
- Ashbaugh DG, Bigelow DB, Petty TL, Levine BE. Acute respiratory distress in adults. Lancet 1967;2:319-23. [Crossref] [PubMed]
- nard GR, Artigas A, Brigham KL, et al. Report of the American-European Consensus conference on acute respiratory distress syndrome: definitions, mechanisms, relevant outcomes, and clinical trial coordination. Consensus Committee. J Crit Care 1994;9:72-81.
- Ferguson ND, Fan E, Camporota L, et al. The Berlin definition of ARDS: an expanded rationale, justification, and supplementary material. Intensive Care Med 2012;38:1573-82. [Crossref] [PubMed]
- Pediatric Acute Lung Injury Consensus Conference Group. Pediatric acute respiratory distress syndrome: consensus recommendations from the Pediatric Acute Lung Injury Consensus Conference. Pediatr Crit Care Med 2015;16:428-39. [Crossref] [PubMed]
- Khemani RG, Smith LS, Zimmerman JJ, et al. Pediatric acute respiratory distress syndrome: definition, incidence, and epidemiology: proceedings from the Pediatric Acute Lung Injury Consensus Conference. Pediatr Crit Care Med 2015;16:S23-40. [Crossref] [PubMed]
- Khemani RG, Rubin S, Belani S, et al. Pulse oximetry vs. PaO2 metrics in mechanically ventilated children: Berlin definition of ARDS and mortality risk. Intensive Care Med 2015;41:94-102. [Crossref] [PubMed]
- Parvathaneni K, Belani S, Leung D, et al. Evaluating the Performance of the Pediatric Acute Lung Injury Consensus Conference Definition of Acute Respiratory Distress Syndrome. Pediatr Crit Care Med 2017;18:17-25. [Crossref] [PubMed]
- Wong JJ, Phan HP, Phumeetham S, et al. Risk Stratification in Pediatric Acute Respiratory Distress Syndrome: A Multicenter Observational Study. Crit Care Med 2017;45:1820-8. [Crossref] [PubMed]
- Gupta S, Sankar J, Lodha R, et al. Comparison of Prevalence and Outcomes of Pediatric Acute Respiratory Distress Syndrome Using Pediatric Acute Lung Injury Consensus Conference Criteria and Berlin Definition. Front Pediatr 2018;6:93. [Crossref] [PubMed]
- Flori H, Dahmer MK, Sapru A, et al. Comorbidities and assessment of severity of pediatric acute respiratory distress syndrome: proceedings from the Pediatric Acute Lung Injury Consensus Conference. Pediatr Crit Care Med 2015;16:S41-50. [Crossref] [PubMed]
- Sapru A, Flori H, Quasney MW, et al. Pathobiology of acute respiratory distress syndrome. Pediatr Crit Care Med 2015;16:S6-22. [Crossref] [PubMed]
- Flori HR, Glidden DV, Rutherford GW, et al. Pediatric acute lung injury: prospective evaluation of risk factors associated with mortality. Am J Respir Crit Care Med 2005;171:995-1001. [Crossref] [PubMed]
- Bem RA, Kneyber MC, van Woensel JB. Respiratory syncytial virus-induced paediatric ARDS: why we should unpack the syndrome. Lancet Respir Med 2017;5:9-10. [Crossref] [PubMed]
- Stylianou-Riga P, Boutsikou T, Kouis P, et al. Maternal and neonatal risk factors for neonatal respiratory distress syndrome in term neonates in Cyprus: a prospective case-control study. Ital J Pediatr 2021;47:129. [Crossref] [PubMed]
- Thompson BT, Chambers RC, Liu KD. Acute Respiratory Distress Syndrome. N Engl J Med 2017;377:562-72. [Crossref] [PubMed]
- Pu S, Wang D, Liu D, et al. Effect of sivelestat sodium in patients with acute lung injury or acute respiratory distress syndrome: a meta-analysis of randomized controlled trials. BMC Pulm Med 2017;17:148. [Crossref] [PubMed]
- Calfee CS, Delucchi K, Parsons PE, et al. Subphenotypes in acute respiratory distress syndrome: latent class analysis of data from two randomised controlled trials. Lancet Respir Med 2014;2:611-20. [Crossref] [PubMed]
- Famous KR, Delucchi K, Ware LB, et al. Acute Respiratory Distress Syndrome Subphenotypes Respond Differently to Randomized Fluid Management Strategy. Am J Respir Crit Care Med 2017;195:331-8. [Crossref] [PubMed]
- Delucchi K, Famous KR, Ware LB, et al. Stability of ARDS subphenotypes over time in two randomised controlled trials. Thorax 2018;73:439-45. [Crossref] [PubMed]
- Sinha P, Delucchi KL, Thompson BT, et al. Latent class analysis of ARDS subphenotypes: a secondary analysis of the statins for acutely injured lungs from sepsis (SAILS) study. Intensive Care Med 2018;44:1859-69. [Crossref] [PubMed]
- Kurdowska A, Noble JM, Grant IS, et al. Anti-interleukin-8 autoantibodies in patients at risk for acute respiratory distress syndrome. Crit Care Med 2002;30:2335-7. [Crossref] [PubMed]
- Oh SB, Aguilan A, Tan HL, et al. The Association Between Alveolar Dead Space Fraction and Mortality in Pediatric Acute Respiratory Distress Syndrome: A Prospective Cohort Study. Front Pediatr 2022;10:814484. [Crossref] [PubMed]
- Ushakumari CJ, Zhou QL, Wang YH, et al. Neutrophil Elastase Increases Vascular Permeability and Leukocyte Transmigration in Cultured Endothelial Cells and Obese Mice. Cells 2022;11:2288. [Crossref] [PubMed]
- Tokuhiro T, Ishikawa A, Sato H, et al. Oxidized Phospholipids and Neutrophil Elastase Coordinately Play Critical Roles in NET Formation. Front Cell Dev Biol 2021;9:718586. [Crossref] [PubMed]
- Cui Y, Zhang M, Xu H, et al. Elastase Inhibitor Cyclotheonellazole A: Total Synthesis and In Vivo Biological Evaluation for Acute Lung Injury. J Med Chem 2022;65:2971-87. [Crossref] [PubMed]
- Maki C, Inoue Y, Ishihara T, et al. Evaluation of appropriate indications for the use of sivelestat sodium in acute respiratory distress syndrome: a retrospective cohort study. Acute Med Surg 2020;7:e471. [Crossref] [PubMed]
- Gao X, Zhang R, Lei Z, et al. Efficacy, safety, and pharmacoeconomics of sivelestat sodium in the treatment of septic acute respiratory distress syndrome: a retrospective cohort study. Ann Palliat Med 2021;10:11910-7. [Crossref] [PubMed]
- Ito H, Nakayama H, Yokose T, et al. A prophylaxis study of acute exacerbation of interstitial pneumonia after lung cancer surgery. Jpn J Clin Oncol 2020;50:198-205. [Crossref] [PubMed]
- Fu J, Kong J, Wang W, et al. The clinical implication of dynamic neutrophil to lymphocyte ratio and D-dimer in COVID-19: A retrospective study in Suzhou China. Thromb Res 2020;192:3-8. [Crossref] [PubMed]
- Laforge M, Elbim C, Frère C, et al. Tissue damage from neutrophil-induced oxidative stress in COVID-19. Nat Rev Immunol 2020;20:515-6. Erratum in: Nat Rev Immunol 2020 Aug 10. [Crossref] [PubMed]
- Yamada K, Kobayashi H, Bo R, et al. Elevation of pivaloylcarnitine by sivelestat sodium in two children. Mol Genet Metab 2015;116:192-4. [Crossref] [PubMed]
- Toyama S, Hatori F, Shimizu A, et al. A neutrophil elastase inhibitor, sivelestat, improved respiratory and cardiac function in pediatric cardiovascular surgery with cardiopulmonary bypass. J Anesth 2008;22:341-6. [Crossref] [PubMed]
- Kikuchi T, Kugimiya T, Katashima S. Usefulness of sivelestat sodium administration in patients with aspiration pneumonia. J Anesth 2006;20:355. [Crossref] [PubMed]
- Matics TJ, Sanchez-Pinto LN. Adaptation and Validation of a Pediatric Sequential Organ Failure Assessment Score and Evaluation of the Sepsis-3 Definitions in Critically Ill Children. JAMA Pediatr 2017;171:e172352. [Crossref] [PubMed]
- Barbateskovic M, Schjørring OL, Russo Krauss S, et al. Higher versus lower fraction of inspired oxygen or targets of arterial oxygenation for adults admitted to the intensive care unit. Cochrane Database Syst Rev 2019;2019:CD012631. [Crossref] [PubMed]
- Terragni PP, Del Sorbo L, Mascia L, et al. Tidal volume lower than 6 ml/kg enhances lung protection: role of extracorporeal carbon dioxide removal. Anesthesiology 2009;111:826-35. [Crossref] [PubMed]
- Amato MB, Meade MO, Slutsky AS, et al. Driving pressure and survival in the acute respiratory distress syndrome. N Engl J Med 2015;372:747-55. [Crossref] [PubMed]
- Ranieri VM, Giunta F, Suter PM, et al. Mechanical ventilation as a mediator of multisystem organ failure in acute respiratory distress syndrome. JAMA 2000;284:43-4. [Crossref] [PubMed]
- Yoshida T, Uchiyama A, Matsuura N, et al. Spontaneous breathing during lung-protective ventilation in an experimental acute lung injury model: high transpulmonary pressure associated with strong spontaneous breathing effort may worsen lung injury. Crit Care Med 2012;40:1578-85. [Crossref] [PubMed]
- Cressoni M, Chiumello D, Chiurazzi C, et al. Lung inhomogeneities, inflation and [18F]2-fluoro-2-deoxy-D-glucose uptake rate in acute respiratory distress syndrome. Eur Respir J 2016;47:233-42. [Crossref] [PubMed]
- Hochberg CH, Semler MW, Brower RG. Oxygen Toxicity in Critically Ill Adults. Am J Respir Crit Care Med 2021;204:632-41. [Crossref] [PubMed]
- Xiao XG, Zu HG, Li QG, et al. Sivelestat sodium hydrate attenuates acute lung injury by decreasing systemic inflammation in a rat model of severe burns. Eur Rev Med Pharmacol Sci 2016;20:528-36. [PubMed]
- Lee JM, Yeo CD, Lee HY, et al. Inhibition of neutrophil elastase contributes to attenuation of lipopolysaccharide-induced acute lung injury during neutropenia recovery in mice. J Anesth 2017;31:397-404. [Crossref] [PubMed]
- Aikawa N, Ishizaka A, Hirasawa H, et al. Reevaluation of the efficacy and safety of the neutrophil elastase inhibitor, Sivelestat, for the treatment of acute lung injury associated with systemic inflammatory response syndrome; a phase IV study. Pulm Pharmacol Ther 2011;24:549-54. [Crossref] [PubMed]
- Kido T, Muramatsu K, Yatera K, et al. Efficacy of early sivelestat administration on acute lung injury and acute respiratory distress syndrome. Respirology 2017;22:708-13. [Crossref] [PubMed]
(English Language Editor: L. Huleatt)