Integrated analysis of immune- and apoptosis-related lncRNA-miRNA-mRNA regulatory network in children with Henoch Schönlein purpura nephritis
Introduction
Henoch-Schönlein purpura (HSP) is the most common IgA-mediated systemic small vessel vasculitis of childhood, characterized by palpable purpuric rash, arthritis, abdominal pain, and renal involvement (1,2). The prognosis of HSP is mostly dependent upon the severity of renal involvement, and chronic kidney disease is detected in up to 20% of children with HSP nephritis (HSPN). Immunosuppressants and multiple-agent therapy have been shown to be beneficial in ameliorating proteinuria and histological severity (3-5).
To date, the precise mechanisms of HSPN have not been elucidated. However, it is worth noting that immune system dysfunction is commonly observed and the serum levels of galactose-deficient IgA1 is drastically elevated in HSPN patients (6,7). A previous study demonstrated that while HSP patients had IgA1-containing circulating immune complexes of small molecular mass, IgA1-IgG-containing circulating immune complexes of large-molecular mass were distinctly present in HSPN patients (6). Besides to IgA1, immune cells also participate in the occurrence and development of HSPN. Infiltrating immune cells are observed in various areas of renal tissue, including the glomerular mesangial and capillary areas, the proximal and distal tubular epithelial cells, and interstitial areas. Involvement of activated cytotoxic T lymphocytes, natural killer cells, interleukin-producing regulatory B cells, macrophage, etc., were discovered in HSPN patients (8-10). Furthermore, existing studies have confirmed that apoptosis also involved in regulating the occurrence and development of HSPN (10-12). Therefore, it is of great importance to explore the underlying immune- and apoptosis-related regulators and molecular mechanisms of HSPN in children.
Long non-coding RNAs (lncRNAs) are non-coding RNAs with a length greater than 200 nucleotides (13,14). Recently, next generation sequencing has identified a great number of lncRNA transcripts and revealed their essential roles in cell differentiation, cell lineage selection, organogenesis, and tissue homeostasis (15-17). The functions of lncRNAs are complex and diverse. Mechanistically, lncRNAs can act as scaffolds, guides, decoys, or enhancers to regulate gene expression and thereby exert their functions (18,19). Recently, there has been much focus on the important roles of lncRNAs in kidney diseases, including acute renal rejection, diabetic nephropathy, membranous nephropathy, chronic kidney disease, and lupus nephritis. For examples, circulating LNC-ephrin type-A receptor 6 (EPHA6) was shown to be a promising marker for vascular injury under acute rejection after kidney transplantation (20). Another study reported that lncRNA NEAT1 serves as a sponge for microRNA (miRNA)-146b to regulate tumor necrosis factor receptor-associated factor 6 (TRAF6) expression and nuclear factor (NF)-κB signaling, thus accelerating renal mesangial cell injury in lupus nephritis (21).
However, the precise lncRNAs involved in the occurrence and development of HSPN and the corresponding molecular mechanisms remain to be elucidated. Few studies have revealed that lncRNAs might play essential roles in the development of HSPN through promoting serum proteins generation and regulating the apoptosis pathway. Pang et al. determined the expression of lncRNAs and messenger RNAs (mRNAs) in the peripheral blood of 6 children with HSPN, and several lncRNAs were associated with the p53 signaling pathway and apoptosis-associated genes, but the study did not explore in depth about the associations between lncRNAs, coding RNAs and proteins (12). Thus, the competing endogenous RNAs (ceRNAs) theory, a novel post-transcriptional regulation mechanism, was applied in our study. It states that lncRNAs and mRNAs can interact with each other via miRNAs by forming a regulatory network, in which LncRNAs can serve as sponges for miRNAs to release the interaction of miRNAs and their target genes, thereby regulating the expression of target genes (22). And the exploring of expression pattern and function of lncRNAs and ceRNAs in children with HSPN is of significant value, providing potential novel strategies for the diagnosis, prognosis, and therapy.
In this study, the lncRNAs and the mRNA expression profiles of high-throughput sequencing dataset GSE102114 were downloaded to screen out the differentially expressed lncRNAs, as well as immune-related genes and apoptosis-related genes in the peripheral blood of children with HSPN. The critical immune- and apoptosis-related genes in children with HSPN were explored through Gene Ontology (GO) and the Kyoto Encyclopedia of Genes and Genomes (KEGG) enrichment analysis, and a protein-protein network (PPI) was constructed. Using Spearman correlation analysis, the lncRNAs that may be involved in the regulation of critical immune- and apoptosis-related genes in children with HSPN were identified. According to the ceRNAs theory, we established a lncRNA-miRNA-mRNA regulatory network, and this may contribute to understand the mechanisms involved in the occurrence and development of HSPN in children. We present the following article in accordance with the STREGA reporting checklist (available at https://tp.amegroups.com/article/view/10.21037/tp-22-437/rc).
Methods
Data collection and processing
The gene expression profiles of dataset GSE102114 were downloaded from the NCBI Gene Expression Omnibus (GEO, https://www.ncbi.nlm.nih.gov/geo/), including the lncRNA and mRNA expression profiles of the peripheral blood of 6 children with HSPN and 4 healthy children (21). A list of 1,811 immune-related genes were downloaded from ImmPort (https://www.immport.org/), of which, 962 immune-related genes were found in the mRNA expression profile of GSE102114 (23). In addition, a total of 306 apoptosis-related genes were identified through merging several classical apoptosis-related gene sets downloaded from the molecular signature database (MSigDB, https://www.gsea-msigdb.org/gsea/msigdb/index.jsp), including KEGG_APOPTOSIS, WP_APOPTOSIS_MODULATION_AND_SIGNALING, WP_APOPTOSIS, REACTOME_SUPPRESSION_OF_APOPTOSIS, REACTOME_ REGULATION_OF_APOPTOSIS,REACTOME_INTRINSIC_PATHWAY_FOR_ APOPTOSIS, REACTOME_APOPTOSIS_INDUCED_DNA_ FRAGMENTATION, and REACTOME_APOPTOSIS (24,25). The expression profile of 279 apoptosis-related genes was extracted from the mRNA expression profile of GSE102114. The list of immune-related genes and apoptosis-related genes is provided in available at https://cdn.amegroups.cn/static/public/tp-22-437-1.xlsx.
Differential expression analysis
Using the “limma” R package, differential expression analysis was conducted to screen out differentially expressed lncRNAs and mRNAs. The differentially expressed immune-related genes and apoptosis-related genes were further identified from the differentially expressed genes. The R packages “pheatmap” and “ggplot2” were used to display the differentially expressed lncRNAs, mRNAs, as well as the immune-related genes and apoptosis-related genes.
GO and KEGG enrichment analysis
GO annotation consists of biological process (BP), cellular component (CC), and molecular function (MF) of genes in different databases using standard expression terms. KEGG (http://www.kegg.jp/) is a bioinformatics resource of genomes and genes by assigning functional and pathway meanings to genomes and genes, which uncover cellular and organism-level functions from genome sequences and other molecular datasets (26-28). Herein, GO and KEGG enrichment analyses were performed using “clusterProfiler” R package for differentially expressed immune-related genes and apoptosis-related genes, with an adjusted P value <0.05 as the threshold. Thereafter, “ggplot2” and “GOplot” R packages were used to show the results of the top 10 terms of BP, CC, and MF in GO and KEGG analyses.
PPI network construction
Venn plots were constructed using the “ggplot2” R package to overlap the differentially expressed genes to identify the critical immune- and apoptosis-related genes in children with HSPN. To explore the interactions among the critical immune- and apoptosis-related genes, these genes were uploaded to STRING (https://string-db.org), a database of functional protein association networks, to obtain their interaction information (29,30). Thereafter, the PPI network was visualized and analyzed by Cytoscape 3.8.2. In addition, the top 2 clusters of the Molecular Complex Detection (MCODE) plugins in Cytoscape were constructed in the complex protein networks.
Correlation analysis
To identify the important immune- and apoptosis-related lncRNAs in children with HSPN, Spearman correlation analyses were conducted to analyze the correlations between differentially expressed lncRNAs and critical immune- and apoptosis-related genes. The absolute value of correlation coefficient |r| >0.9 and P value <0.001 was set as the threshold to filter the lncRNAs that were correlated with immune- and apoptosis-related genes.
LncRNA-miRNA and miRNA-mRNA prediction, lncRNA-miRNA-mRNA regulatory network construction
MiRcode (http://mircode.org/) is a database that predicts miRNA targets based on the comprehensive GENCODE gene annotation consisting of more than 10,000 lncRNAs. The potential lncRNA-miRNA pairs were predicted by miRcode. In addition, the file containing the mRNA-miRNA pairs, released on September 2021, was retrieved from TargetScanHuman 8.0 (https://www.targetscan.org/vert_80/docs/help.html/) (31). Based on the ceRNA mechanism, lncRNAs and mRNAs that were predicted to bind to the same miRNAs and exhibit positive correlations were considered as ceRNAs. Consequently, the immune- and apoptosis-related lncRNA-miRNA-mRNA for HSPN in children was constructed and visualized using Cytoscape 3.8.2.
Validation by quantitative real-time polymerase chain reaction (qRT-PCR)
The peripheral blood samples of 11 HSP/HSPN children and 3 age-matched healthy children were enrolled from the Children’s Hospital, Zhejiang University School of Medicine (Hangzhou, China) between August 2021 and November 2021. HSP was diagnosed according to the criteria outlined by the Society of Pediatrics, Chinese Medical Association in 2013 (32). HSPN was diagnosed as the presence of either hematuria and/or proteinuria during the first 6 months of HSP (33). None of the patients had complications or had taken any immunosuppressants prior to this study. The clinical characteristics of the children are presented in Table S1. The research was approved by the Ethics Committee of the Children’s Hospital, Zhejiang University School of Medicine (No. 2022-IRB-015). Informed consent was taken from all the participants’ guardians, and the study was conducted in accordance with the Declaration of Helsinki (as revised in 2013). The total RNA of these samples was extracted using the RNA Extraction Kit (Omega, Guangzhou, China). Reverse transcription was conducted using PrimeScript RT Master Mix Kit (Takara, Dalian, China). The expression of lncRNAs was assessed using the TB Green Premix Ex Taq Kit (Takara, Dalian, China) in accordance with the manufacturer’s instructions. Primers used in this study are listed in Table S2. The relative expression of lncRNAs was calculated using the 2−ΔΔCt method, with glyceraldehyde-3-phosphate dehydrogenase (GAPDH) as the reference. Two-sided unpaired Student’s t-test was applied to compare the difference in lncRNA expression levels between the peripheral blood samples of 11 HSP/HSPN children and 3 age-matched healthy children. The corresponding results were visualized using R package “ggplot2”.
Statistical analysis
Data were analyzed using SPSS (Version 23.0; SPSS, Inc., Chicago, IL, USA) and GraphPad Prism (Version 8.0; GraphPad Software, CA, USA). For the differential expression analysis, |log2 fold change(log2FC) |>1 and adjusted P value <0.05 were set as the screening criteria. For qRT-PCR analysis, all data collected from three independent replicates were presented as mean ± standard deviation. Differences between groups were analyzed by Student’s t-test. P<0.05 were considered to be statistically significant.
Results
Identification of the differentially expressed lncRNAs and mRNAs in children with HSPN
The differentially expressed lncRNAs and mRNAs were identified from the GSE102114 dataset, and the results are presented in Figure 1. A total of 396 differentially expressed lncRNAs was identified, among which, 184 were upregulated and 212 were downregulated (available at https://cdn.amegroups.cn/static/public/tp-22-437-2.xlsx). The significant expression patterns and distribution of the differentially expressed lncRNAs between healthy controls and the HSPN group are shown in Figure 1A,1C, respectively. A total of 5,417 differentially expressed genes were identified, including 2,737 upregulated genes and 2,680 downregulated genes (available at https://cdn.amegroups.cn/static/public/tp-22-437-3.xlsx). The significant expression patterns and distribution of the differentially expressed mRNAs between healthy controls and the HSPN group are illustrated in Figure 1B,1D, respectively.
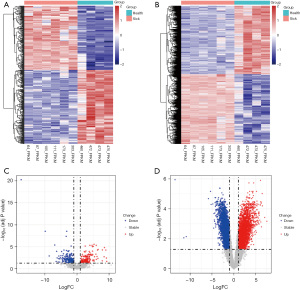
Abnormally expressed immune-related mRNAs in children with HSPN
Since HSPN is characterized as an autoimmune disorder, 314 differentially expressed immune-related genes (including 185 upregulated genes and 129 downregulated genes) were filtered from 5,417 differentially expressed genes. The significant expression patterns and distribution of the differentially expressed immune-related genes between healthy controls and HSPN children are shown in Figure 2A,2B, respectively. GO analysis demonstrated that the 314 genes were significantly enriched in BP including regulation of innate immune response, T cell activation, and positive regulation of cytokine production; CC including external side of plasma membrane, membrane raft, and membrane microdomain; and MF including receptor ligand activity, cytokine binding, and cytokine receptor activity (Figure 2C). Overall, these terms were all closely associated with immune function. Similarly, KEGG analysis revealed that these 314 genes were significantly enriched in multiple immune-related pathways, including T cell receptor signaling pathway, natural killer cell mediated cytotoxicity, and B cell receptor signaling pathway (Figure 2D).
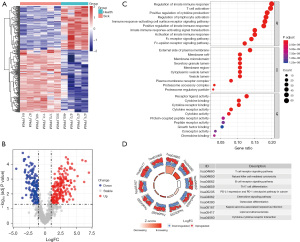
Abnormally expressed apoptosis-related mRNAs in children with HSPN
A total of 124 differentially expressed apoptosis-related genes were identified in children with HSPN, including 71 upregulated and 53 downregulated genes. The significant expression patterns and distribution of the differentially expressed apoptosis-related genes between healthy controls and the HSPN group are shown in Figure 3A,3B, respectively. Moreover, GO analysis demonstrated that these 124 genes were significantly enriched in BP including the inactivation of innate immune response, response to tumor necrosis factor (TNF), and interleukin-1-mediated signaling pathway; CC including proteasome complex, endopeptidase complex, and peptidase complex; and MF including endopeptidase activity and ubiquitin protein ligase binding (Figure 3C). Interestingly, these terms were also correlated with immune functions. In addition, KEGG analysis revealed that these 124 genes were significantly enriched in apoptosis, proteasome, spinocerebellar ataxia, and lipid and atherosclerosis (Figure 3D).
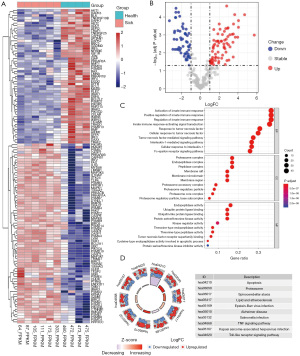
Identification of important immune- and apoptosis-related mRNAs and lncRNAs in children with HSPN
By intersecting the differentially expressed immune-related and apoptosis-related genes, a total of 43 genes which may simultaneously regulate immune function and cell apoptosis in children with HSPN was identified (Figure 4A). The interactions among these immune- and apoptosis-related genes and the PPI network of these genes are displayed in Figure 4B. The top two highly interconnected subclusters were analyzed using the MCODE algorithm of Cytoscape 3.8.2, and scored 9.75 and 9.0, respectively (Figure 4C,4D). We then defined 100 lncRNAs that were significantly correlated with the above 43 genes as immune- and apoptosis-related lncRNAs in children with HSPN. The specific correlations of immune- and apoptosis-related lncRNAs and mRNAs in children with HSPN are displayed in available at https://cdn.amegroups.cn/static/public/tp-22-437-4.xlsx.
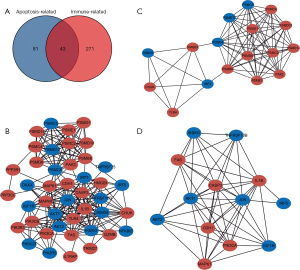
Construction of the immune- and apoptosis-related lncRNA–miRNA–mRNA ceRNA network for children with HSPN
Immune- and apoptosis-related lncRNAs and mRNAs that have positive correlations are considered ceRNAs and used to construct the ceRNA regulatory network. The miRNAs which might bridge the lncRNAs and mRNAs were identified by matching lncRNA-miRNA pairs and miRNA-mRNA pairs (Figure 5A,5B). Correspondingly, the immune- and apoptosis-related lncRNA-miRNA-mRNA regulatory network for children with HSPN was established (Figure 5C). The specific regulatory relationship in the ceRNA network is displayed in Figure 6 and available at https://cdn.amegroups.cn/static/public/tp-22-437-5.xlsx.

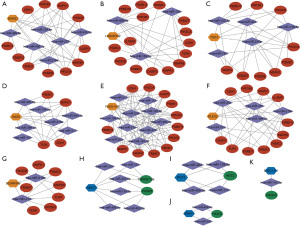
Validation of the expression levels of the lncRNAs in children with HSPN
To further confirm the expression levels of the lncRNAs in the immune- and apoptosis-related lncRNA-miRNA-mRNA regulatory network, qRT-PCR was conducted using clinical samples. Consistent with the results of the GSE102114 dataset, the expression levels of small nucleolar RNA host gene-3 (SNHG3), LINC00152, taurine up-regulated 1 (TUG1), growth-arrest-specific transcript 5 (GAS5), PH domain containing 5 antisense RNA 1 (FGD5-AS1), deleted in lymphocytic leukemia 2 (DLEU2), and small Cajal body-specific RNA 9 (SCARNA9) were significantly upregulated (Figure 7A), while the expression of nuclear-enriched abundant transcript 1 (NEAT1), plasmacytoma variant translocation 1 (PVT1), SNHG1, and disrupted-in-schizophrenia 1-intronic transcript 1 (DISC1-IT1) were dramatically downregulated in the blood samples of HSPN patients compared to normal blood samples (Figure 7B). Therefore, the upregulation of SNHG3, LINC00152, TUG1, GAS5, FGD5-AS1, DLEU2, and SCARNA9 may be potential biomarkers for HSPN. Similarly, the downregulation of NEAT1, PVT1, SNHG1, and DISC1-IT1 may be potential indicators for HSPN.
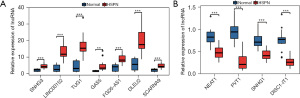
Discussion
HSPN accounts for approximately 80% of children with secondary glomerulopathy, and renal involvement is the most important prognostic factor in determining morbidity and mortality of HSP patients. Extensive clinical and experimental data have shown that disorders of cellular and humoral immune responses are closely related to the pathogenesis of HSPN in children (6,34). However, the key genes responsible for the dysregulation of humoral and cellular immunity during the progression of HSPN in children remain unknown.
The present study identified 314 differentially expressed immune-related mRNAs in the peripheral blood of children with HSPN. GO and KEGG enrichment analysis revealed that these mRNAs were involved in multiple immune-related biological processes, including regulation of innate immune response, cytokine production, lymphocyte activation, T cell and B cell receptor signaling pathway, which indicating that activation of T cells and B cells, as well as elevated cytokine and chemokine production, are closely associated with the dysregulation of immune-related mRNAs in children with HSPN. These results also corroborated previous studies that multiple immune cells participate in the increased secretion of inflammatory mediators and the deposition of IgA-containing immune complexes, thereby inducing the development of HSPN (6-10). Thus, we speculated that the dysregulation of these differentially expressed immune-related mRNAs may contribute to the immune dysfunction in children with HSPN.
Apoptosis is another critical factor in children with HSPN (11). Herein, we identified 124 differentially expressed apoptosis-related mRNAs in the peripheral blood of children with HSPN. Interestingly, GO enrichment analysis revealed that these apoptosis-related mRNAs also participate in the regulation of immune-related processes, and KEGG enrichment analysis demonstrated that these mRNAs are significantly enriched in apoptosis, proteasome, lipid and atherosclerosis, TNF signaling pathways, and toll-like receptor signaling pathways. Previous studies have confirmed that the deposition of IgA-containing immune complexes is responsible for the renal damage observed in HSPN patients. During the progression of HSPN, IgA deposition may induce apoptosis of human umbilical vein endothelial cells, thereby causing HSP vascular endothelial damage (35). On the other hand, apoptosis may promote the removal of inflammatory cells, contributing to the control of early inflammatory response and repair self-limiting vasculitis (36). Hence, there is a complex regulatory link between immune and apoptosis-associated signaling pathways in children with HSPN. By overlapping the differentially expressed immune- and apoptosis-related mRNAs, a total of 43 mRNAs were identified as critical genes, and these may play essential regulatory roles in the pathogenesis of HSPN in children.
Recently, accumulating studies have demonstrated that lncRNAs play important roles in the regulation of immune and apoptosis-related pathways (37-39). However, the critical lncRNAs responsible for the dysregulation of immune response and apoptosis in children with HSPN remain largely unknown. In this study, a total of 100 lncRNAs that correlated with the above 43 mRNAs were identified as immune- and apoptosis-related lncRNA, and the immune- and apoptosis-related lncRNAs-miRNA-mRNAs regulatory network was first constructed based on ceRNAs mechanism in children with HSPN. In this regulatory network, elevated expression of SNHG3, LINC00152, TUG1, GAS5, FGD5-AS1, DLEU2, and SCARNA9 might promote the expression of TLR4, IL1RAP, CDH1, PSMC2, PSMD1, PSMD6, PSMD10, PSMD14, PIK3CA, PIK3CB, CASP3, PPP3R1, TRIM27, HMGB1, MAPK1, and MAPK8; while decreased expression of SNHG1, NEAT1, DISC1-IT1, and PVT1 might induce the downregulation of AKT2, TNFRSF10B, PIK3R5, and PIK3CD.
The dysregulation of these 11 lncRNAs were validated using the peripheral blood of children with HSPN, and the results suggested that these lncRNAs may act as potential biomarkers for the diagnosis of HSPN in children. Studies thus far have mainly focused on the roles of these lncRNAs in regulating the occurrence and development of various cancers (40,41). SNHG3, TUG1, GAS5, and SCARNA9 have been identified as immune-related lncRNAs in different cancers (42-45), and the anti-apoptotic effects of SNHG3, LINC00152, TUG1, and FGD5-AS1 have been validated in different cancer cell models (41,46-48). Nevertheless, to date, their immunological and apoptotic roles in the progression of HSPN in children have not been explored. Based on the results of the present study, the dysregulation of TUG1, DLEU2, PVT1 was significant in HSPN group compared to healthy controls, and interestingly, the reliably correlation between these lncRNAs and progression of several kidney diseases (such as diabetic nephropathy, membranous nephropathy, and renal clear cell carcinoma) had been verified in previous studies (49-51). Thus, the above three lncRNAs could be selected as candidate key lncRNAs in further explorative studies to identify a novel ceRNA axis and to explore potential therapeutic drugs in HSPN. In addition to traditional treatment strategies such as steroidal steroid and immunosuppressants, innovative tissue-specific RNA delivery by selective organ targeting nanoparticles should be expected (52).
There were several limitations in this study. First, the sample size used for analysis and validation were relatively small, and the results presented herein should be further validated using larger cohorts. Second, miRNAs in the immune- and apoptosis-related lncRNA-miRNA-mRNA regulatory network were determined by miRNA-target prediction, and updating computer technology is needed to explore the complexities of this network and provide more possibilities for further clinical translation. Third, the specific functions of the key lncRNAs in HSPN need further experimental verification by in vivo and in vitro studies, through lentiviral interference vector, transcriptional repression/inhibition, RNA overexpression/interference, etc. Meanwhile, we sampled peripheral whole blood lncRNAs but did not collect the samples from the clinical renal tissues and peripheral lymphocytes, and the relevant studies is under way. Finally, several drawbacks in the clinical application of lncRNAs should be noticed. Determining the subcellular localization is important for understanding mechanism and designing strategies for manipulating lncRNA expression and function. Engineering animal models to express the lncRNAs may be necessary, to overcome the lack of conservation between lncRNA sequences in humans and animal models (53). In addition, for the relatively poor predictive ability of single-lncRNA, multivariable prediction models with a combination of multi-lncRNAs and disease characteristics, are warranted.
In conclusion, the present study constructed an immune- and apoptosis-related lncRNA-miRNA-mRNA regulatory network of HSPN in children, which provided a basis and direction for future studies involving the molecular mechanisms of the pathogenesis of HSPN in children. These lncRNAs and mRNAs in the immune- and apoptosis-related lncRNA-miRNA-mRNA regulatory network have potential to be novel diagnostic and therapeutic biomarkers for children with HSPN.
Acknowledgments
Funding: This project support was provided by the Natural Science Foundation of Zhejiang Province (Grant No. LQY20H300002 to Jing Miao) and the Hospital Pharmacy Research Funding Project of Zhejiang Pharmaceutical Association (Grant No. 2014ZYY05 to Lingfei Huang).
Footnote
Reporting Checklist: The authors have completed the STREGA reporting checklist. Available at https://tp.amegroups.com/article/view/10.21037/tp-22-437/rc
Data Sharing Statement: Available at https://tp.amegroups.com/article/view/10.21037/tp-22-437/dss
Conflicts of Interest: All authors have completed the ICMJE uniform disclosure form (available at https://tp.amegroups.com/article/view/10.21037/tp-22-437/coif). LH reports funding support from the Hospital Pharmacy Research Funding Project of Zhejiang Pharmaceutical Association (Grant No. 2014ZYY05 to L Huang). J Miao reports funding support from the Natural Science Foundation of Zhejiang Province (Grant No. LQY20H300002 to J Miao). The other authors have no conflicts of interest to declare.
Ethical Statement: The authors are accountable for all aspects of the work in ensuring that questions related to the accuracy or integrity of any part of the work are appropriately investigated and resolved. The research was approved by the Ethics Committee of the Children’s Hospital, Zhejiang University School of Medicine (No. 2022-IRB-015). Informed consent was taken from all the participants’ guardians, and the study was conducted in accordance with the Declaration of Helsinki (as revised in 2013).
Open Access Statement: This is an Open Access article distributed in accordance with the Creative Commons Attribution-NonCommercial-NoDerivs 4.0 International License (CC BY-NC-ND 4.0), which permits the non-commercial replication and distribution of the article with the strict proviso that no changes or edits are made and the original work is properly cited (including links to both the formal publication through the relevant DOI and the license). See: https://creativecommons.org/licenses/by-nc-nd/4.0/.
References
- McCarthy HJ, Tizard EJ. Clinical practice: Diagnosis and management of Henoch-Schönlein purpura. Eur J Pediatr 2010;169:643-50. [Crossref] [PubMed]
- Yang YH, Yu HH, Chiang BL. The diagnosis and classification of Henoch-Schönlein purpura: an updated review. Autoimmun Rev 2014;13:355-8. [Crossref] [PubMed]
- Leung AKC, Barankin B, Leong KF. Henoch-Schönlein Purpura in Children: An Updated Review. Curr Pediatr Rev 2020;16:265-76. [Crossref] [PubMed]
- Lau KK, Suzuki H, Novak J, et al. Pathogenesis of Henoch-Schönlein purpura nephritis. Pediatr Nephrol 2010;25:19-26. [Crossref] [PubMed]
- Chen JY, Mao JH. Henoch-Schönlein purpura nephritis in children: incidence, pathogenesis and management. World J Pediatr 2015;11:29-34. [Crossref] [PubMed]
- Su Q, Jiang L, Chai J, et al. Changes of Peripheral Blood Lymphocyte Subsets and Immune Function in Children with Henoch-Schonlein Purpura Nephritis. Iran J Immunol 2021;18:259-67. [PubMed]
- Duan X, Yu D, Yu C, et al. Henoch-Schönlein purpura following high-voltage electric burn injury: A case report and review of the literature. Exp Ther Med 2016;11:540-2. [Crossref] [PubMed]
- Imai T, Nishiyama K, Ueki K, et al. Involvement of activated cytotoxic T lymphocytes and natural killer cells in Henoch-Schönlein purpura nephritis. Clin Transl Immunology 2020;24:e1212. [Crossref] [PubMed]
- Yang B, Tan X, Xiong X, et al. Effect of CD40/CD40L signaling on IL-10-producing regulatory B cells in Chinese children with Henoch-Schönlein purpura nephritis. Immunol Res 2017;65:592-604. [Crossref] [PubMed]
- Irabu H, Shimizu M, Kaneko S, et al. Apoptosis inhibitor of macrophage as a biomarker for disease activity in Japanese children with IgA nephropathy and Henoch-Schönlein purpura nephritis. Pediatr Res 2021;89:667-72. [Crossref] [PubMed]
- Yuan P, Bo Y, Ming G, et al. Apoptosis of human umbilical vein endothelial cells (HUVEC) induced by IgA1 isolated from Henoch-Schonlein purpura children. Asian Pac J Allergy Immunol 2014;32:34-8. [PubMed]
- Pang S, Lv J, Wang S, et al. Differential expression of long non-coding RNA and mRNA in children with Henoch-Schönlein purpura nephritis. Exp Ther Med 2019;17:621-32. [PubMed]
- Jarroux J, Morillon A, Pinskaya M. History, Discovery, and Classification of lncRNAs. Adv Exp Med Biol 2017;1008:1-46. [Crossref] [PubMed]
- St Laurent G, Wahlestedt C, Kapranov P. The Landscape of long noncoding RNA classification. Trends Genet 2015;31:239-51. [Crossref] [PubMed]
- Kazimierczyk M, Kasprowicz MK, Kasprzyk ME, et al. Human Long Noncoding RNA Interactome: Detection, Characterization and Function. Int J Mol Sci 2020;21:1027. [Crossref] [PubMed]
- Melissari MT, Grote P. Roles for long non-coding RNAs in physiology and disease. Pflugers Arch 2016;468:945-58. [Crossref] [PubMed]
- Yip CW, Sivaraman DM, Prabhu AV, et al. Functional annotation of lncRNA in high-throughput screening. Essays Biochem 2021;65:761-73. [Crossref] [PubMed]
- Chen LL. Linking Long Noncoding RNA Localization and Function. Trends Biochem Sci 2016;41:761-72. [Crossref] [PubMed]
- Qian X, Zhao J, Yeung PY, et al. Revealing lncRNA Structures and Interactions by Sequencing-Based Approaches. Trends Biochem Sci 2019;44:33-52. [Crossref] [PubMed]
- Groeneweg KE, Duijs JMGJ, Florijn BW, et al. Circulating Long Noncoding RNA LNC-EPHA6 Associates with Acute Rejection after Kidney Transplantation. Int J Mol Sci 2020;21:5616. [Crossref] [PubMed]
- Zhang LH, Xiao B, Zhong M, et al. LncRNA NEAT1 accelerates renal mesangial cell injury via modulating the miR-146b/TRAF6/NF-κB axis in lupus nephritis. Cell Tissue Res 2020;382:627-38. [Crossref] [PubMed]
- Wang Y, Hou J, He D, et al. The Emerging Function and Mechanism of ceRNAs in Cancer. Trends Genet 2016;32:211-24. [Crossref] [PubMed]
- Bhattacharya S, Dunn P, Thomas CG, et al. ImmPort, toward repurposing of open access immunological assay data for translational and clinical research. Sci Data 2018;5:180015. [Crossref] [PubMed]
- Liberzon A, Birger C, Thorvaldsdóttir H, et al. The Molecular Signatures Database (MSigDB) hallmark gene set collection. Cell Syst 2015;1:417-25. [Crossref] [PubMed]
- Subramanian A, Tamayo P, Mootha VK, et al. Gene set enrichment analysis: a knowledge-based approach for interpreting genome-wide expression profiles. Proc Natl Acad Sci U S A 2005;102:15545-50. [Crossref] [PubMed]
- Kanehisa M, Goto S. KEGG: kyoto encyclopedia of genes and genomes. Nucleic Acids Res 2000;28:27-30. [Crossref] [PubMed]
- Kanehisa M. Toward understanding the origin and evolution of cellular organisms. Protein Sci 2019;28:1947-51. [Crossref] [PubMed]
- Kanehisa M, Furumichi M, Sato Y, et al. KEGG: integrating viruses and cellular organisms. Nucleic Acids Res 2021;49:D545-51. [Crossref] [PubMed]
- Szklarczyk D, Gable AL, Lyon D, et al. STRING v11: protein-protein association networks with increased coverage, supporting functional discovery in genome-wide experimental datasets. Nucleic Acids Res 2019;47:D607-13. [Crossref] [PubMed]
- Szklarczyk D, Gable AL, Nastou KC, et al. The STRING database in 2021: customizable protein-protein networks, and functional characterization of user-uploaded gene/measurement sets. Nucleic Acids Res 2021;49:D605-12. [Crossref] [PubMed]
- McGeary SE, Lin KS, Shi CY, et al. The biochemical basis of microRNA targeting efficacy. Science 2019;366:eaav1741.
- Subspecialty Group of Renal Diseases. the Society of Pediatrics, Chinese Medical Association. Evidence-based guideline for diagnosis and treatment of Henoch-Schonlein purpura nephritis (2016). Zhonghua Er Ke Za Zhi 2017;55:647-51.
- Davin JC, Coppo R. Henoch-Schönlein purpura nephritis in children. Nat Rev Nephrol 2014;10:563-73. [Crossref] [PubMed]
- Wang Q, Shi YY, Cao M, et al. Role of Imbalance between Th17 Cells and Treg Cells in the Pathogenesis of Children with Henoch-Schonlein Purpura. Zhongguo Shi Yan Xue Ye Xue Za Zhi 2015;23:1391-6. [PubMed]
- Del Vecchio GC, Penza R, Altomare M, et al. Cytokine pattern and endothelium damage markers in Henoch-Schönlein purpura. Immunopharmacol Immunotoxicol 2008;30:623-9. [Crossref] [PubMed]
- Wang J, Zheng Y, Chen G, et al. The changes in pyroptosis-related inflammatory factors in the peripheral blood of patients with Henoch-Schonlein purpura. Ann Palliat Med 2021;10:6687-93. [Crossref] [PubMed]
- Chen YG, Satpathy AT, Chang HY. Gene regulation in the immune system by long noncoding RNAs. Nat Immunol 2017;18:962-72. [Crossref] [PubMed]
- Denaro N, Merlano MC, Lo Nigro C. Long noncoding RNAs as regulators of cancer immunity. Mol Oncol 2019;13:61-73. [Crossref] [PubMed]
- Zhang Y, Mao Q, Xia Q, et al. Noncoding RNAs link metabolic reprogramming to immune microenvironment in cancers. J Hematol Oncol 2021;14:169. [Crossref] [PubMed]
- Zheng S, Jiang F, Ge D, et al. LncRNA SNHG3/miRNA-151a-3p/RAB22A axis regulates invasion and migration of osteosarcoma. Biomed Pharmacother 2019;112:108695. [Crossref] [PubMed]
- Yuan ZJ, Yu C, Hu XF, et al. LINC00152 promotes pancreatic cancer cell proliferation, migration and invasion via targeting miR-150. Am J Transl Res 2020;12:2241-56. [PubMed]
- Xu B, Mei J, Ji W, et al. LncRNA SNHG3, a potential oncogene in human cancers. Cancer Cell Int 2020;20:536. [Crossref] [PubMed]
- Zhan T, Gao X, Wang G, et al. Construction of Novel lncRNA-miRNA-mRNA Network Associated With Recurrence and Identification of Immune-Related Potential Regulatory Axis in Hepatocellular Carcinoma. Front Oncol 2021;11:626663. [Crossref] [PubMed]
- Wang W, Zhao Z, Yang F, et al. An immune-related lncRNA signature for patients with anaplastic gliomas. J Neurooncol 2018;136:263-71. [Crossref] [PubMed]
- Chen Q, Hu L, Huang D, et al. Six-lncRNA Immune Prognostic Signature for Cervical Cancer. Front Genet 2020;11:533628. [Crossref] [PubMed]
- Wang Z, Liu Y, Zhang J, et al. An Immune-Related Long Noncoding RNA Signature as a Prognostic Biomarker for Human Endometrial Cancer. J Oncol 2021;2021:9972454. [Crossref] [PubMed]
- Li T, Chen Y, Zhang J, et al. LncRNA TUG1 promotes cells proliferation and inhibits cells apoptosis through regulating AURKA in epithelial ovarian cancer cells. Medicine (Baltimore) 2018;97:e12131. [Crossref] [PubMed]
- Hao L, Wang J, Bi SJ, et al. Upregulation of Long Noncoding RNA FGD5-AS1 Ameliorates Myocardial Ischemia/Reperfusion Injury via MicroRNA-106a-5p and MicroRNA-106b-5p. J Cardiovasc Pharmacol 2021;78:e45-54. [Crossref] [PubMed]
- Li L, Long J, Mise K, et al. PGC1α is required for the renoprotective effect of lncRNA Tug1 in vivo and links Tug1 with urea cycle metabolites. Cell Rep 2021;36:109510. [Crossref] [PubMed]
- Li J, Zhao Q, Jin X, et al. Silencing of LncRNA PVT1 inhibits the proliferation, migration and fibrosis of high glucose-induced mouse mesangial cells via targeting microRNA-93-5p. Biosci Rep 2020;40:BSR20194427. [Crossref] [PubMed]
- Qi-Dong X, Yang X, Lu JL, et al. Development and Validation of a Nine-Redox-Related Long Noncoding RNA Signature in Renal Clear Cell Carcinoma. Oxid Med Cell Longev 2020;2020:6634247. [Crossref] [PubMed]
- Cheng Q, Wei T, Farbiak L, et al. Selective organ targeting (SORT) nanoparticles for tissue-specific mRNA delivery and CRISPR-Cas gene editing. Nat Nanotechnol 2020;15:313-20. [Crossref] [PubMed]
- Matsui M, Corey DR. Non-coding RNAs as drug targets. Nat Rev Drug Discov 2017;16:167-79. [Crossref] [PubMed]