Factors associated with short-term survival in neonates with hyperammonemia
Introduction
Serum ammonia is a catabolite of amino acids, which can easily cross the blood-brain barrier and get into the brain. When present in high concentrations, ammonia is toxic to the body, especially the central nervous system (1). When serum ammonia levels increase, hyperammonemia will manifest as vomiting, seizures, disturbance of consciousness, and even coma, potentially resulting in death (2). Surviving infants with hyperammonemia may have severe neurological sequelae, causing heavy burdens on their family and society (3).
There are many factors leading to hyperammonemia, such as liver disease, gastrointestinal blooding, seizures and so on (4). However, hyperammonemia in neonates is mainly attributable to congenital metabolic diseases, including urea cycle defects (UCDs), organic acidemias (OAs), fatty acid oxidation defects and so on (3). These diseases were rare, which leaving the neonatal hyperammonemia in a rare condition (prevalence 5:10,000 live births) (5). As a member of urea cycle, hyperammonemia may leave a metabolic disorder in the body, including metabolic acidosis and electrolyte disturbance. Moreover, these symptoms and other basic diseases may increase the production and decrease the clearness of ammonia in turn. Initial treatments for hyperammonemia include discontinuing protein intake, providing sufficient caloric support, and administering metabolite scavengers (MS) and/or initiating hemodialysis, based on the suggestions provided by the latest edition of Avery’s Diseases of the Newborn (6). However, the mortality rate in neonates with hyperammonemia is still high (25–50%) (7).
We aimed to describe the characteristics of hyperammonemic neonates, and compare the characteristics of neonates who died and who survived. We also analyzed the prognostic factors in neonates with hyperammonemia and explore the potential time point to perform early intervention. Herein, a retrospective and single-center study was conducted to investigate the factors associated with short-term survival of patients with hyperammonemia admitted to the neonatal intensive care unit (NICU). We present the following article in accordance with the STROBE reporting checklist (available at https://tp.amegroups.com/article/view/10.21037/tp-22-70/rc).
Methods
Data collection
This retrospective study was conducted at Children’s Hospital of Fudan University, a national children’s medical center. All neonates with hyperammonemia (age <29 d, ammonia level >100 µmol/L) admitted to NICU between April 2013 and June 2020 were included. The final diagnosis was confirmed by biochemical and molecular investigations, including plasma analysis, hematuria tandem mass spectrometry, and multigene panel testing after admission. In general, patients of the Children’s Hospital of Fudan University were referred to the NICU after the appearance of symptoms. In a minority of cases, patients were transferred to the Children’s Hospital of Fudan University to obtain additional care and treatment after failing to respond to treatment at a local hospital.
Data on general and demographic characteristics were collected at admission. Ammonia was measured according to the standard laboratory methods of Children’s Hospital of Fudan University and was collected at admission and during the NICU stay. Δ (Δ= last ammonia level − first ammonia level) was used to represent the change in ammonia level. Yes represents Δ<0, and No represents Δ≥0. We recorded the days from admission to discharge or death as the length of stay and the days between symptoms appearance and presentation. The MSs administered were intravenous (IV) arginine hydrochloride (250–500 mg/kg/d), IV levocarnitine (250 mg/kg/d) and oral (PO) citrulline (200 mg/kg/d), with the dichotomous variables recorded in the database (yes/no). The dichotomous variables also included acidosis, electrolyte disturbance, seizure, coma, dystonia, abnormal movement, invasive mechanical ventilation, neonatal brain injury, pneumonia, sepsis, respiratory distress syndrome (RDS), protein intake disruption, and continuous venovenous hemodialysis (CVVHD). Acidosis and electrolyte disturbance were diagnosed based on biochemical investigations. The diseases analyzed were diagnosed based on clinical diagnostic criteria by staff neonatologists.
A comparison between the survival group and the non-survival group was made for the total study population and after excluding patients with information loss and age limitation. A flowchart of this study is shown in Figure 1. The survival group was defined as patients with improvement upon discharge, and the non-survival group was defined as patients who died during the hospital stay or required advanced life support equipment upon hospital discharge. The time of survival after discharge in the survival group was followed up by telephone. The study was conducted in accordance with the Declaration of Helsinki (as revised in 2013). The study was approved by Ethics Committee of Children Hospital of Fudan University (No. 2020-515) and individual consent for this retrospective analysis was waived.
Statistical analysis
Data were analyzed using the statistical software Stata 15. Average values with full ranges (minimum–maximum) were calculated for continuous variables, and numbers and/or frequencies (%) were calculated for binary or categorical data. A comparative analysis of data from surviving patients versus non-surviving patients was performed. Normal values were compared using a t test if they passed the normality test (Shapiro–Wilk test). The Wilcoxon rank sum test was used for nonnormally distributed data, and Fisher’s exact test was used to assess qualitative variables. Independent values were first analyzed by univariate analysis and then analyzed by multivariate logistic regression analysis. Time-dependent ROC curves and Kaplan-Meier method were used to validate the survival value of the factors for hyperammonemic patients. Odds ratios (ORs) and 95% confidence intervals (CIs) were calculated for all parameters in both groups. The alpha error was 5%, and a P value of <0.05 was considered to be statistically significant. All P values were two-sided.
Results
Demographics and patient characteristics
A total of 62 patients who were diagnosed with hyperammonemia before or after admission were admitted to the NICU. The male to female sex ratio was 2.1. The average birth weight was 2,861.95 g (range, 630–4,600 g). Eighteen (29%) patients had low birth weight (<2500 g). The average gestational age was 264.1 d (range, 179–295 d). Fifteen (24.2%) patients were born prematurely. Thirty-three (53.2%) patients were born by cesarean delivery (Table 1).
Table 1
Characteristics | Survival group | Non-survival group | Total population | P value | OR (95% CI) |
---|---|---|---|---|---|
Number | 30 | 32 | 62 | – | – |
Type of disease, n | – | – | |||
UCDs | 2 | 1 | 3 | ||
OAs | 4 | 7 | 11 | ||
Age at admission to NICU, days, mean [range] | 8.1 [0–25] | 6.3 [0–25] | 7.2 [0–25] | 0.115 | – |
Time between symptoms appearance and presentation, days, mean [range] | 5.6 [0–22] | 4.1 [0–19] | 4.8 [0–22] | 0.056 | – |
GA, days, mean [range] | 267.2 [202–295] | 261.3 [179–289] | 264.1 [179–295] | 0.287 | – |
Preterm, n (%) | 6 (20.0) | 9 (28.1) | 15 (24.2) | 0.558 | 0.639 (0.196–2.081) |
Sex (male/female), n | 20/10 | 22/10 | 42/20 | 1.000 | 0.9091 (0.313–2.639) |
Cesarean delivery, n (%) | 17 (56.7) | 16 (50.0) | 33 (53.2) | 0.621 | 1.308 (0.481–3.558) |
Birth weight, g, mean [range] | 2,999 [1,025–4,600] | 2,737 [630–4,230] | 2,861.95 [630–4,600] | 0.226 | – |
Low birth weight, n (%) | 8 (26.7) | 10 (31.3) | 18 (29.0) | 0.783 | 0.800 (0.266–2.408) |
OR, odds ratio; CI, confidence interval; NICU, neonatal intensive care unit; GA, gestational age; UCDs, urea cycle defects; OAs, organic acidemias.
Among 62 cases, there were three UCD and eleven OA cases. Among the three UCD cases, there were two cases of citrullinemia and one case of carbamoyl phosphate synthetase 1 deficiency. Among the eleven OA cases, there were five cases of propionic acidemia, five cases of methylmalonic acidemia, and one case of glutaric acidemia. Among the remaining cases, there were two cases of carnitine-acylcarnitine translocate deficiency, while the rest were due to unknown causes. The mean age at admission was 7.2 d (range, 0–25 d). The mean time between symptoms appearance and presentation was 4.8 d (range, 0–22 d). There were no significant differences in baseline characteristics between the two groups. Among these cases, there were some specific cases in family history. One case was in the survival group whose parents were cousins. There were two cases in the non-survival group, who were the test-tube babies. Two cases in the non-survival group who were twins both with the carnitine translocation enzyme deficiency, whose brother died 2-day-old with congenital adipose metabolic disorder. One case in the survival group had a sister died with alaninemia. The mother of one case in the non-survival group had a history of two abnormal neonatal deaths.
Clinical features and management
A comparative analysis between the survival group and the non-survival group according to clinical features and management is detailed in Table 2. The non-survival group had higher ammonia levels at the first ammonia level measurement (600 vs. 344.9 µmol/L, P=0.008), the peak ammonia level measurement (882.2 vs. 433.3 µmol/L, P=0.002), and the last ammonia level measurement (693.4 vs. 157.9 µmol/L, P=0.000) than the survived group. There were 39 (62.9%) patients with a ∆<0 (∆= last ammonia level − first ammonia level), which meant that there were a reduce of ammonia level at the discharge. The odds of Δ<0 in the survival group was 9.5 times higher than that in the non-survival group (P<0.000). The average length of stay was 13.7 d (range, 1–89 d). The average length of stay in the survival group was 22.3 d (range, 1–89 d), while that in the non-survival group was 5.6 d (range, 1–32 d).
Table 2
Variables | Survival group (N=30) | Non-survival group (N=32) | Total population | P value | OR (95% CI) |
---|---|---|---|---|---|
First ammonia level, µmol/L, mean [range] | 344.9 [62.5–1,123] | 600 [80–1,199] | 476.5 [62.5-1,199] | 0.008* | – |
Peak ammonia level, µmol/L, mean [range] | 433.4 [114–1,247] | 882.2 [101–2,409] | 665.0 [101-2,409] | 0.002* | – |
Last ammonia level, µmol/L, mean [range] | 157.9 [31–1,204] | 693.4 [86–2,409] | 434.3 [31–2,409] | 0.000* | – |
Δ <0, n (%) | 26 (86.7) | 13 (40.6) | 39 (62.9) | 0.000* | 9.500 (2.675–33.74) |
Length of stay, days, mean [range] | 22.3 [1–89] | 5.6 [1–32] | 13.7 [1–89] | <0.000* | – |
Acidosis, n (%) | 10 (33.3) | 19 (59.4) | 29 (46.8) | 0.047* | 0.342 (0.121–0.965) |
Electrolyte disturbance, n (%) | 6 (20.0) | 15 (46.9) | 21 (33.9) | 0.033* | 0.283 (0.091–0.880) |
Seizure, n (%) | 10 (33.3) | 9 (28.1) | 19 (30.6) | 0.785 | 1.278 (0.433–3.770) |
Coma, n (%) | 2 (6.7) | 12 (37.5) | 14 (22.6) | 0.005* | 0.119 (0.024–0.592) |
Dystonia, n (%) | 16 (53.3) | 22 (68.8) | 38 (61.3) | 0.298 | 0.520 (0.184–1.464) |
Abnormal movement, n (%) | 9 (30.0) | 12 (37.5) | 21 (33.9) | 0.598 | 0.714 (0.248–2.061) |
Invasive mechanical ventilation, n (%) | 8 (26.7) | 28 (87.5) | 36 (58.1) | 0.000* | 0.052 (0.014–0.195) |
Neonatal brain injury, n (%) | 16 (53.3) | 12 (37.5) | 28 (45.2) | 0.307 | 1.905 (0.691–5.248) |
Pneumonia, n (%) | 6 (20.0) | 7 (21.9) | 13 (21.0) | 1 | 0.893 (0.262–3.043) |
Sepsis, n (%) | 6 (20.0) | 12 (37.5) | 18 (29.0) | 0.166 | 0.417 (0.133–1.311) |
RDS, n (%) | 4 (13.3) | 4 (12.5) | 8 (12.9) | 1 | 1.077 (0.244–4.757) |
Protein intake disruption, n (%) | 28 (93.3) | 30 (93.8) | 58 (93.5) | 1 | 0.933 (0.244–4.757) |
MS administration, n (%) | 19 (63.3) | 24 (75.0) | 43 (69.4) | 0.411 | 0.576 (0.193–1.716) |
CVVHD, n (%) | 0 (0) | 3 (9.4) | 3 (4.8) | 0.239 | 0.138 (0.007–2.794) |
The asterisk designates statistical significance, with a P value less than 0.05. NICU, neonatal intensive care unit; OR, odds ratio; CI, confidence interval; Δ= last ammonia level − first ammonia level; RDS, respiratory distress syndrome; MS, metabolite scavenger; CVVHD, continuous venovenous hemodialysis. The P value corresponds to the comparison of the two groups.
There were 29 neonates (46.8%) with acidosis, 21 neonates (33.9%) with electrolyte disturbance, and 19 neonates (30.6%) with seizures. Coma was observed in 14 neonates (22.6%), dystonia in 38 neonates (61.3%), and abnormal movements in 21 neonates (33.9%). Thirty-six patients were intubated (58.1%). Twenty-eight neonates (45.2%) were found to have neonatal brain injury with electroencephalogram (EEG) or neuroimaging abnormalities. Pneumonia, sepsis and RDS were observed in 13 (21.0%), 18 (29.0%) and 8 neonates (12.9%), respectively.
Protein intake was stopped in 58 neonates (93.5%) during their NICU stay, and all neonates received intravenous high-caloric and protein-free intake providing glucose and lipids. A total of 43 neonates (69.4%) received MS administration, which mainly comprised IV arginine hydrochloride. Only three neonates (4.8%) received CVVHD.
Acidosis [odds ratio (OR): 0.342, 95% CI: 0.121–0.965, P=0.047], electrolyte disturbance (OR: 0.283, 95% CI: 0.091–0.880, P=0.033), coma (OR: 0.119; 95% CI: 0.024–0.592, P=0.005), and invasive mechanical ventilation (OR: 0.052; 95% CI: 0.014–0.195, P=0.000) was associated with the increased risk of death. There were no significant differences between the groups with respect to the rates of seizure, dystonia, abnormal movement, neonatal brain injury, pneumonia, sepsis, RDS, protein intake disruption, MS administration and CVVHD.
Time course of ammonia levels in the total population
The time course of average ammonia levels according to the group (survival group vs. non-survival group) is detailed in Figure 2. The first detected ammonia level was considered as the level at Day 0. On Day 0, Day 1, Day 2, and Day 3, the average ammonia levels were 476.5, 577.9, 525.0, and 447.7 µmol/L, respectively. The average ammonia levels were 344.9, 338.9, 367.9, and 184.7 µmol/L in the survival group on Day 0, Day 1, Day 2, and Day3, and 600, 781.1, 693.2, and 710.6 µmol/L in the non-survival group.
Multivariate logistic regression analysis
We selected 6 variables that were significant in the univariate analyses plus MS administration as independent variables for inclusion in the multivariate logistic regression analysis. The results are shown in Table 3. In model 1, we included ∆, the length of stay, coma and MS administration in the multivariate logistic regression analysis. The results show that the variables ∆, length of stay, and MS administration were significant factors in non-surviving neonates with hyperammonemia (P<0.05). In model 2, the above seven factors were entered into the multivariate logistic regression analysis, and only length of stay and invasive mechanical ventilation were found to be significant factors (P<0.05).
Table 3
Variable | Unadjusted OR (95% CI) | Model 1 | Model 2 | |||||
---|---|---|---|---|---|---|---|---|
Adjusted death percentage, mean (range) | Adjusted OR (95% CI) |
P value | Adjusted death percentage, mean (range) | Adjusted OR (95% CI) |
P value | |||
Δ<0 | ||||||||
No | Ref | 81.2% (45.8–95.7%) | Ref | Ref | 64.6% (22.9–91.8%) | Ref | Ref | |
Yes | 0.11 (0.03–0.37) | 21.3% (5.0–58.2%) | 0.06 (0.01–0.65) | 0.02* | 26.0% (5.1–69.5%) | 0.19 (0.02–2.16) | 0.182 | |
Length of stay, days | 0.87 (0.80–0.94) | N/A | 0.90 (0.83–0.98) | 0.011* | N/A | 0.88 (0.80–0.97) | 0.010* | |
Acidosis | ||||||||
No | Ref | N/A | N/A | N/A | 34.7% (10.6–70.5%) | Ref | Ref | |
Yes | 2.92 (1.04–8.24) | N/A | N/A | N/A | 54.6% (16.8–87.8%) | 2.26 (0.38–13.56) | 0.372 | |
Electrolyte disturbance | ||||||||
No | Ref | N/A | N/A | N/A | 35.1% (11.1–70.1%) | Ref | Ref | |
Yes | 3.53 (1.14–10.95) | N/A | N/A | N/A | 54.1% (15.6–88.2%) | 2.18 (0.34–13.97) | 0.411 | |
Coma | ||||||||
No | Ref | 37.0% (19.2–59.2%) | Ref | Ref | 46.4% (19.6–75.5%) | Ref | Ref | |
Yes | 8.40 (1.69–41.74) | 66.5% (22.2–93.3%) | 3.39 (0.47–24.57) | 0.228 | 42.5% (7.6–87.0%) | 0.85 (0.09–8.33) | 0.893 | |
Invasive mechanical ventilation | ||||||||
No | Ref | N/A | N/A | N/A | 14.6% (2.5–53.2%) | Ref | Ref | |
Yes | 19.25 (5.12–72.33) | N/A | N/A | N/A | 78.9% (43.8–94.7%) | 21.86 (2.70–177.17) | 0.004* | |
MS administration | ||||||||
No | Ref | 23.4% (4.4–67.2%) | Ref | Ref | 34.5% (4.6%, 85.1%) | Ref | Ref | |
Yes | 1.79 (0.58–5.53) | 79.2% (47.5–94.1%) | 12.48 (1.05–148.15) | 0.046* | 54.9% (21.9%, 84.0%) | 2.31 (0.14–38.73) | 0.561 |
The asterisk designates statistical significance, with a P value less than 0.05. OR, odds ratio; CI, confidence interval; Ref, reference; N/A, not applicable; Δ= last ammonia level − first ammonia level; MS, metabolite scavenger.
Survival outcomes
We followed the children up to 1 year of age by telephone, and four patients were lost. Kaplan-Meier survival analysis was conducted to assess the impact of the 6 variables on overall survival in the study cohort. For the same endpoints, ∆<0 was associated with better survival (P<0.0001) (Figure 3A). Patients with coma have a worse survival (P=0.0008) (Figure 3B). With the use of invasive mechanical ventilation, there was worse survival (P<0.0001) (Figure 3C). Patients with electrolyte disturbance have a worse survival (P=0.03) (Figure 3D). There was no difference of survival about acidosis and MS administration (Figure 3E,3F).
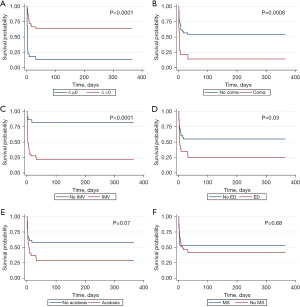
Receiver operating characteristic curve
To explore the relationship of peak ammonia level with the survival, the receiver operating characteristic (ROC) curve was used and based on the peak ammonia levels. Area under curve (AUC) was 0.737 (95% CI: 0.603–0.870) (Figure 4). The ROC curve also highlights potential sensitivity/specificity combinations at various cutpoints. For the best cut-off value, using a cut-point of ≥406.5 (peak ammonia levels >406.5 µmol/L) to define a negative screen gave sensitivity of 71.88% and specificity of 76.92%.
Discussion
Historically, survival among patients with hyperammonemia due to inborn errors of metabolism has been poor (8). Because of severe enzyme deficiency or a high level of ammonia, most neonates die; however, some survive to infancy but experience neurological defects (8,9). Since 1979, scientists have hypothesized that hyperammonemia caused by an inborn error of urea synthesis could be treated with non-urea metabolites to activate alternative ammonia synthesis and excretion pathways (10,11). Over the past several decades, technical advances and a combination of MS administration and dialysis in infants with hyperammonemia have led to prolonged survival and improved clinical outcomes (12). Although there have been many advances in neonatal care and treatment, hyperammonemic neonates are still at risk of death (13). As a result, there is a pressing need to identify potential factors that affect short-term survival. Based on clinical data, we conducted this retrospective study, with the aim of identifying factors that will help improve survival and offer benefits to neonates and clinicians.
On the basis of our study of 62 hyperammonemic neonates, length of stay and invasive mechanical ventilation were the most significant factors, followed by a ∆<0 and MS administration. Although there were no significant differences in the rates of coma and acidosis after adjustment for all selected variables, the rates of coma and acidosis were higher in the non-survival group than in the survival group; coma and acidosis may lead to a higher usage rate of invasive mechanical ventilation. In our study, when the length of stay was increased by 1 d, the mortality rate decreased by 0.88 times, mainly due to long-term neonatal care and effective treatment during the hospital stay. A prospective study on survival after treatment with MSs for UCDs showed that slower reductions in ammonia levels may result in worse outcomes (12). Similarly, the decrease in the ammonia level in the non-survival group was slower than that in the survival group in the first three days after the first ammonia level measurement.
Additionally, we analyzed the factors affecting the short-term survival. We have found that the condition of ∆<0, invasive mechanical ventilation, electrolyte disturbance, and coma may result in a high risk of death. And we found that when peak ammonia levels were more than 406.5 µmol/L, the further intervention should be carried out to save the lives of patients, such as dialysis.
MS administration is a classical treatment for hyperammonemia in neonates and has been studied for many years. When administered MSs in the clinic, ammonia may decrease to normal or near-normal levels; clinical improvement and prolonged survival have been observed (11,12,14,15). Currently, renal replacement therapy is considered a beneficial treatment option for patients with hyperammonemia (13). Dialysis, including hemofiltration, intermittent hemodialysis, and continuous hemodiafiltration, can decrease ammonia levels more quickly than MS administration alone (6,16). The rapid decrease in ammonia levels can improve short-term survival and even neurological sequelae (17,18). However, dialysis in neonates for hyperammonemia treatment may cause some side effects, such as hemodynamic instability, peritonitis, and tunnel infection, and couldn’t prevent poor neurodevelopmental outcomes (6,19,20). A retrospective study showed that final ammonia levels were similar between the dialyzed group and non-dialyzed group (6). MS administration is less invasive and has a lower risk of complications than CVVHD. Local hospitals should administer MS in hyperammonemic neonates to decrease the ammonia level as soon as possible. Moreover, a combination therapy of MS administration and dialysis may improve short-term survival and neurological sequelae.
Our study has several limitations due to its retrospective nature. First, our sample size was small, which likely reduced the significance of the logistic regression model. Second, the study provided little data on dialysis. Due to the basic physical conditions and economic status of the patients as well as the poor prognosis of hyperammonemia, dialysis was rarely applied in neonates with hyperammonemia. Last, we did not report the side effects of MS administration.
Conclusions
MS administration is a beneficial treatment for hyperammonemia in neonates. Increasing the length of stay in the NICU could help improve short-term survival. Further intervention should be administered when peak ammonia levels >406.5 µmol/L.
Acknowledgments
The authors would like to thank Yanchen Wang (Children’s hospital of Fudan University) for the support in providing helpful suggestions of statistics in this article.
Funding: None.
Footnote
Reporting Checklist: The authors have completed the STROBE reporting checklist. Available at https://tp.amegroups.com/article/view/10.21037/tp-22-70/rc
Data Sharing Statement: Available at https://tp.amegroups.com/article/view/10.21037/tp-22-70/dss
Peer Review File: Available at https://tp.amegroups.com/article/view/10.21037/tp-22-70/prf
Conflicts of Interest: All authors have completed the ICMJE uniform disclosure form (available at https://tp.amegroups.com/article/view/10.21037/tp-22-70/coif). The authors have no conflicts of interest to declare.
Ethical Statement: The authors are accountable for all aspects of the work in ensuring that questions related to the accuracy or integrity of any part of the work are appropriately investigated and resolved. The study was conducted in accordance with the Declaration of Helsinki (as revised in 2013). The study was approved by Ethical Committee of Children Hospital of Fudan University (No. 2020-515) and individual consent for this retrospective analysis was waived.
Open Access Statement: This is an Open Access article distributed in accordance with the Creative Commons Attribution-NonCommercial-NoDerivs 4.0 International License (CC BY-NC-ND 4.0), which permits the non-commercial replication and distribution of the article with the strict proviso that no changes or edits are made and the original work is properly cited (including links to both the formal publication through the relevant DOI and the license). See: https://creativecommons.org/licenses/by-nc-nd/4.0/.
References
- Soria LR, Brunetti-Pierri N. Ammonia and autophagy: An emerging relationship with implications for disorders with hyperammonemia. J Inherit Metab Dis 2019;42:1097-104. [Crossref] [PubMed]
- Häberle J, Chakrapani A, Ah Mew N, et al. Hyperammonaemia in classic organic acidaemias: a review of the literature and two case histories. Orphanet J Rare Dis 2018;13:219. [Crossref] [PubMed]
- Ribas GS, Lopes FF, Deon M, et al. Hyperammonemia in Inherited Metabolic Diseases. Cell Mol Neurobiol 2022;42:2593-610. [Crossref] [PubMed]
- Yao ZP, Li Y, Liu Y, et al. Relationship between the incidence of non-hepatic hyperammonemia and the prognosis of patients in the intensive care unit. World J Gastroenterol 2020;26:7222-31. [Crossref] [PubMed]
- Waters D, Adeloye D, Woolham D, et al. Global birth prevalence and mortality from inborn errors of metabolism: a systematic analysis of the evidence. J Glob Health 2018;8:021102. [Crossref] [PubMed]
- Abily-Donval L, Dupic L, Joffre C, et al. Management of 35 critically ill hyperammonemic neonates: Role of early administration of metabolite scavengers and continuous hemodialysis. Arch Pediatr 2020;27:250-6. [Crossref] [PubMed]
- Häberle J, Burlina A, Chakrapani A, et al. Suggested guidelines for the diagnosis and management of urea cycle disorders: First revision. J Inherit Metab Dis 2019;42:1192-230. [Crossref] [PubMed]
- Batshaw ML. Inborn errors of urea synthesis. Ann Neurol 1994;35:133-41. [Crossref] [PubMed]
- Summar ML, Mew NA. Inborn Errors of Metabolism with Hyperammonemia: Urea Cycle Defects and Related Disorders. Pediatr Clin North Am 2018;65:231-46. [Crossref] [PubMed]
- Brusilow SW, Valle DL, Batshaw M. New pathways of nitrogen excretion in inborn errors of urea synthesis. Lancet 1979;2:452-4. [Crossref]
- Batshaw ML, Brusilow S, Waber L, et al. Treatment of inborn errors of urea synthesis: activation of alternative pathways of waste nitrogen synthesis and excretion. N Engl J Med 1982;306:1387-92. [Crossref] [PubMed]
- Enns GM, Berry SA, Berry GT, et al. Survival after treatment with phenylacetate and benzoate for urea-cycle disorders. N Engl J Med 2007;356:2282-92. [Crossref] [PubMed]
- Demirkol D, Aktuğlu Zeybek Ç, Karacabey BN, et al. The Role of Supportive Treatment in the Management of Hyperammonemia in Neonates and Infants. Blood Purif 2019;48:150-7. [Crossref] [PubMed]
- Brusilow S, Tinker J, Batshaw ML. Amino acid acylation: a mechanism of nitrogen excretion in inborn errors of urea synthesis. Science 1980;207:659-61. [Crossref] [PubMed]
- Brusilow SW, Danney M, Waber LJ, et al. Treatment of episodic hyperammonemia in children with inborn errors of urea synthesis. N Engl J Med 1984;310:1630-4. [Crossref] [PubMed]
- Arbeiter AK, Kranz B, Wingen AM, et al. Continuous venovenous haemodialysis (CVVHD) and continuous peritoneal dialysis (CPD) in the acute management of 21 children with inborn errors of metabolism. Nephrol Dial Transplant 2010;25:1257-65. [Crossref] [PubMed]
- Uchino T, Endo F, Matsuda I. Neurodevelopmental outcome of long-term therapy of urea cycle disorders in Japan. J Inherit Metab Dis 1998;21:151-9. [Crossref] [PubMed]
- Picca S, Dionisi-Vici C, Bartuli A, et al. Short-term survival of hyperammonemic neonates treated with dialysis. Pediatr Nephrol 2015;30:839-47. [Crossref] [PubMed]
- Imani PD, Carpenter JL, Bell CS, et al. Peritoneal dialysis catheter outcomes in infants initiating peritoneal dialysis for end-stage renal disease. BMC Nephrol 2018;19:231. [Crossref] [PubMed]
- Kido J, Matsumoto S, Häberle J, et al. Long-term outcome of urea cycle disorders: Report from a nationwide study in Japan. J Inherit Metab Dis 2021;44:826-37. [Crossref] [PubMed]