Robotic-assisted surgery in pediatrics: what is evidence-based?—a literature review
Introduction
The use of the da Vinci Surgical System (Intuitive Surgical, Sunnyvale, CA, USA) has increased up to 40-fold over the past decade when compared to traditional laparoscopic procedures in general surgery (1). Improved dexterity, precision, 3D visualization, and ergonomics are robotic-assisted surgical features that allow surgeons to overcome the disadvantages of the traditional (2D) laparoscopic surgery, leading to better surgical results (2). In addition, several training curricula, on-site training programs, and robotic surgery virtual reality simulators have been developed and are currently being implemented for surgeons and trainees to gain adequate surgical competence (3,4).
The use of robotic-assisted surgery (RAS) has increased more slowly in pediatrics than in the adult population (5,6). Despite the many advantages of robotic instruments, such as the ability to mimic the movements of the human wrist, highly magnified 3D visualization, and tremor filtration, the da Vinci Surgical System still presents some limitations for use in pediatric surgery. The principal reasons for the low use of this new technology in children are: (I) the difficulty in developing surgical robots and related instruments appropriately sized for smaller children and neonates, and (II) the elevated costs for pediatric hospitals correlated with the smaller volume of patients eligible for robotic procedures (6,7). Even though new platforms are being developed, the da Vinci Surgical System is actually the leading robotic surgical technology approved for pediatric use; instruments are available in sizes of 8 and 5 mm and the recommended distance between the ports is at least 8 cm (7,8).
The aim of this study was to describe through a narrative synthesis the evidence-based indications for RAS in the different fields of pediatric surgery according to the published literature. We present the following article in accordance with the Narrative Review reporting checklist (available at https://tp.amegroups.com/article/view/10.21037/tp-22-338/rc).
Methods
Eligibility criteria and information sources
An electronic search was conducted of MEDLINE, Scopus, and Web of Science. Using Boolean operators AND/OR, all possible combinations of the following search terms were used: robotic surgery, pediatrics, neonatal surgery, thoracic surgery, abdominal surgery, urologic surgery, hepatobiliary surgery, and surgical oncology. The selection criteria were limited to the English language, pediatric patients (under 18 years of age), and articles published after 2010. A flow diagram of the literature search strategy is reported in Figure 1.
Study selection
Titles and abstracts were screened for relevance by two reviewers independently (EG, MRC). All relevant articles meeting inclusion criteria were selected and any disagreement was resolved by a senior reviewer (AB). Commentaries, editorials, short notes, and letters to the editor were excluded. The search strategy summary is reported in Table 1. Oxford score for therapeutic studies was used for evaluating the articles in order to select those with the highest level of evidence (Table 2).
Table 1
Items | Specification |
---|---|
Date of search | 28th February 2022 |
Databases and other sources searched | MEDLINE, Scopus, Web of Science |
Search terms used (Table S1) | MeSH terms: Robotic surgery, pediatrics, neonatal surgery, thoracic surgery, abdominal surgery, urologic surgery, hepatobiliary surgery, surgical oncology; Boolean operators: AND/OR |
Timeframe | 1st January 2010–28th February 2022 |
Inclusion and exclusion criteria | The selection criteria were limited to the English language, pediatric patients (under 18 years of age), and articles published after 2010. Commentaries, editorials, short notes, and letters to the editor were excluded |
Selection process | Titles and abstracts were screened for relevance by two reviewers independently (EG, MRC). All relevant articles meeting inclusion criteria were selected and any disagreement was resolved by a senior reviewer (AB) |
Any additional considerations, if applicable | Primary screening excluded articles consisting of duplicates, off-topic works, and original languages other than English |
Table 2
Level | Evidence |
---|---|
IA | Systematic review (with homogeneity) of randomized controlled trials |
IB | Individual randomized controlled trials (with narrow confidence intervals) |
IC | All or none study (met when all patients died before the intervention became available, but some now survive on it; or when some patients died before the intervention became available, but none now die on it) |
IIA | Systematic review (with homogeneity) of cohort studies |
IIB | Individual Cohort study (including low quality randomized controlled trials) |
IIC | “Outcomes” research or Ecological studies |
IIIA | Systematic review (with homogeneity) of case-control studies |
IIIB | Individual Case-control study |
IV | Case series (and poor quality cohort and case-control studies) |
V | Expert opinion without explicit critical appraisal or based on physiology bench research or “first principles” |
* Table edited and adapted according to Burns PB, Rohrich RJ, Chung KC. The levels of evidence and their role in evidence-based medicine. Plast Reconstr Surg. 2011 Jul;128(1):305-310 and https://www.cebm.ox.ac.uk/resources/levels-of-evidence/oxford-centre-for-evidence-based-medicine-levels-of-evidence-march-2009, produced by Bob Phillips, Chris Ball, Dave Sackett, Doug Badenoch, Sharon Straus, Brian Haynes, Martin Dawes since November 1998. Updated by Jeremy Howick March 2009.
Results
The search retrieved 824 abstracts on pediatric robotic-assisted surgery from electronic databases. Of these, 75 (9%) focused on thoracic RAS, 80 (10%) on the use of RAS in neonates, 171 (21%) on RAS for pediatric abdominal procedures, 439 (53%) on RAS in urology, 41 (5%) on the use of RAS in hepatobiliary, and 18 (2%) on oncological diseases. Primary screening excluded 585 articles consisting of duplicates, off-topic works, and original languages other than English. Subsequently, 239 abstracts were reviewed. The selected abstracts included 23 (10%) on pediatric thoracic diseases, 192 (80%) on pediatric urologic anomalies, and 14 (6%), 8 (3%), and 2 (1%), respectively, on the treatment of pediatric tumors, abdominal surgical pathologies, and hepatobiliary diseases. Interestingly, none of the articles addressed specific indications for the use of the da Vinci surgical system in newborns. Figure 2 summarizes the distribution of RAS publications among the current pediatric literature. Ultimately, 10 published articles with the highest level of evidence according to the guidelines for therapeutics studies and Oxford Score (9,10) met the purpose of our work and were examined. Most of the articles included in this review reported evidence-based indications in pediatric urological surgery. An overview on the 10 articles included in the final analysis is reported in Table 3.
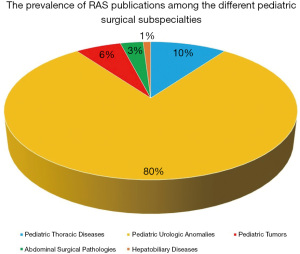
Table 3
No. | Year | Authors | Title | Article type | Key contents | Level of evidence* |
---|---|---|---|---|---|---|
1 | 2020 | Denning NL, et al. (6) | Pediatric Robotic Surgery | Review | ? The majority of studies about robotic-assisted pyeloplasty reported success rates in excess of 90%. Robotic procedures demonstrated to have shorter hospital length of stay and require less pain medication, but longer operative times. Pyeloplasty in infants less than 10 kg seems to be safe with no significant differences in outcomes or complications | 2A |
? Robotic-assisted ureteral reimplantation demonstrated to have longer operative times, higher 90-day complication rate (13% vs. 4.5%), and higher median hospital cost | ||||||
? No difference between operating time, duration of hospital stay, rate of conversion, and rates of intraoperative or postoperative complications were found between laparoscopic and robotic fundoplication of the stomach | ||||||
? Conversion and complication rates for robotic hepatobiliary procedures are still too high | ||||||
? Contraindications for minimally invasive surgery in children include a high risk of tumor fracture and spillage, significant adhesions from previous operations, or significant impairment in respiratory or cardiovascular physiology | ||||||
2 | 2013 | Cundy TP, et al. (11) | The first decade of robotic surgery in children | Systematic Review | ? The most prevalent genitourinary, gastrointestinal and thoracic procedures were pyeloplasty, fundoplication of the stomach, and lobectomy, respectively | 2A |
? The overall reported conversion rate was 2.5% | ||||||
3 | 2014 | Cundy TP, et al. (12) | Meta analysis of robot-assisted versus conventional laparoscopic fundoplication in children | Systematic Review & Meta-analysis | ? No statistically significant differences between robotic and laparoscopic fundoplication for conversions, operating time, length of hospital stay, and post-operative complication were found | 2A |
? Cost analysis revealed a 29% higher per case cost for robotic procedures | ||||||
4 | 2015 | Mahida JB, et al. (13) | Utilization and costs associated with robotic surgery in children | Comparative Study | ? The most common robotic surgeries performed were pyeloplasty and ureteral reimplantation; followed by nephrectomy, partial nephrectomy, fundoplication, and cholecystectomy | 2B |
? Postoperative length of stay was shorter after robotic urologic surgeries, but overall hospitalization costs were higher | ||||||
5 | 2017 | Madadi-Sanjani O, et al. (14) | Minimally Invasive Hepatobiliary Surgery | Review | ? Minimally invasive techniques can be recommended for the resection of choledochal cysts and for cholecystectomy | 2A |
? Further data are needed before recommending the use of minimally invasive techniques for biliary atresia and hepatic tumors | ||||||
6 | 2018 | Cave J, et al. (15) | Paediatric robotic surgery | Review | ? Robotic-assisted pyeloplasty demonstrated a high success rate, and resulted in a significantly shorter hospital stay and lower rate of complication when compared with laparoscopic procedure | 2A |
? No significant difference in postoperative complications, and reduced length of stay and postoperative pain were found after robotic-assisted ureteral reimplantation | ||||||
? A meta-analysis on 297 children found that despite a tendency towards conversion to open surgery in the laparoscopic fundoplication group (6.1% vs. 3%), there was no significant difference in postoperative complications between robotic (8.9%) and laparoscopic fundoplication (8%) | ||||||
7 | 2021 | Esposito C, et al. (16) | Robotics and future technical developments in pediatric urology | Review | ? The main indications of robotic surgery in pediatric urology are pyeloplasty for ureteropelvic junction obstruction and ureteral reimplantation according to Lich Gregoire technique | 2A |
? The main limitation of robotic surgery remains the excessive costs and the limited lifespan of robotic instruments | ||||||
8 | 2021 | Chandrasekharam VVS, et al. (17) | A systematic review and meta-analysis of conventional laparoscopic versus robot-assisted laparoscopic pyeloplasty in infants | Systematic Review & Meta-analysis | ? No significant difference between the success rates of laparoscopic or robotic-assisted pyeloplasty was found (97.5% vs. 94.8%) | 2A |
? The mean age at operation was significantly lower for laparoscopic (5.6±1.8 months) than robotic-assisted pyeloplasty (7.2±1.2 months) | ||||||
? The duration of surgery was significantly higher for robotic-assisted pyeloplasty (179±49 vs. 137±45 min) | ||||||
? The mean time to discharge was 2 days for laparoscopic pyeloplasty while 1.3 days for robotic-assisted pyeloplasty | ||||||
? The overall complication rate was significantly higher for robotic-assisted procedure | ||||||
9 | 2022 | Ferrero PA, et al. (18) | The potential and the Limitations of Esophageal Robotic Surgery in Children | Multicenter Cohort Study & Review | ? The main limitation is the low body weight of children results in incompatibility between the size of the trocars and the size of the intercostal space | 2B |
? Esophageal robotic-assisted thoracoscopic surgery appears to be a feasible procedure for pediatric cases with body weights more than 15 kg | ||||||
10 | 2022 | Blanc T, et al. (19) | Robotic Surgery in Pediatric Oncology: Lessons learned from the First 100 Tumors – A Nationwide Experience | Prospective Cohort Study | ? Robotic surgery for pediatric tumors is a safe option in highly selected cases. Indications should be discussed by tumor boards to avoid widespread and uncontrolled application | 2C |
*, According to Oxford Score and guidelines for therapeutic studies.
Discussion
Since the 1980s, minimally invasive surgery (MIS) has demonstrated its feasibility and safety in the adult population compared to open surgery. However, this evidence has not been confirmed in pediatric surgery, and MIS is considered feasible and safe in childhood only when performed by experienced surgeons (11,20). RAS is largely adopted in the adult population for urological, gynecologic, and colorectal surgery due to better outcomes in operative time, conversion rate, and length of hospital stay (LOS) (21,22). Conversely, the use of RAS in pediatrics is still controversial and evidence-based indications in the current literature are lacking.
This review aimed to describe in a narrative manner the different applications of RAS in pediatrics and to identify evidence-based indications according to the current literature. In the absence of specific literature, the current state of MIS in a subspecialty has been reported in brief.
MIS in neonates and infants is a relatively new field, evolving over the last two decades and requiring the development of new techniques and instruments. The attention of surgeons and industries is focused on developing robotic instruments (3 mm or less) able to work in small body cavities and requiring shorter distance between the ports or a single-port access, which could lead to wider use of RAS in newborns and small infants (23,24). Furthermore, the use of RAS in some subspecialties, such as for hepatobiliary diseases, is still limited by the lack of comparative studies and large-scale case series that confirm the advantages of minimally invasive surgery in these patients (14). Interestingly, recent studies including systematic reviews, meta-analyses, comparative studies, and prospective case-series showed the safety and feasibility of laparoscopic surgery for choledochal cyst excision and cholecystectomy (14,25-28); however, it cannot be actually recommended for infants with biliary atresia and pediatric hepatobiliary tumors (14,29,30). Overall, the literature provides evidence supporting the use of MIS; in particular, the indications for RAS in pediatric patients presenting hepatobiliary diseases remain very scarce (13,14,31). Additionally, although a panel of experts recently stated that for pediatric living donor liver transplantation MIS should be the standard approach for left lateral section donor hepatectomies, RAS for living donor hepatectomy could be considered an alternative for skilled surgeons but requires further investigation (32,33).
The use of the da Vinci surgical system has been reported in the general thoracic field since the beginning of this century. The first report of RAS for the treatment of thoracic pathologies in adults was published in 2002. However, controversy remains about the application of RAS, with a lack of evidence in the adult population (34). In the pediatric population, video-assisted thoracoscopic surgery (VATS) seems to have many advantages when compared with traditional thoracic surgery. Nonetheless, the level of evidence is not high enough (35). Further, robotic-assisted thoracoscopic surgery (RATS) in children presents several technical difficulties for neonatal surgeries in particular. In two retrospective multicentric studies recently published, the authors concluded that RATS could be suitable for older children with a body weight of at least 15–20 kg, and currently there is a persistent lack of evidence that lower weight children and neonates are candidates for RATS due to an incompatibility between the size of the robotic instruments, the intercostal space, and the small thoracic working space (18,36).
To date, the most common procedures described using the da Vinci Surgical System are pyeloplasty and ureteral reimplantation, followed by fundoplication of the stomach (5,37). Interestingly, a review of the literature and a meta-analysis reporting outcomes of children undergoing mini-invasive fundoplication found no significant difference in terms of conversions, operating time, length of hospital stay, and postoperative complications. Nevertheless, the results are significantly limited by the absence of long-term follow-up. Notably, the cost analysis revealed a 29% higher per case cost for robotic procedures (12,38). Moreover, numerous case reports and series in the current pediatric literature document diverse successful applications of RAS for abdominal surgical procedures such as splenectomy, Heller’s myotomy, intestinal anastomosis, anorectal pull-through (e.g., for Hirschsprung disease), ovarian cystectomy, and salpingo-oophorectomy (15,39-42). However, further studies are needed to evaluate whether RAS in these procedures gives a real advantage over MIS. Conversely, an analysis of the literature shows that the main indication of RAS in pediatrics is represented by pediatric urology indications. Specifically, the main indications for RAS in pediatric urology are: (I) pyeloplasty for ureteropelvic junction obstruction, and (II) ureteral reimplantation according to the Lich-Gregoire technique, principally in case of bilateral reflux or high degree reflux with megaureter (15,16,43,44). Notably, even though several studies have documented advantageous outcomes in older children, the application of RAS in infants with urological diseases weighing less than 10 kg is still being investigated and a cut-off weight has yet to be determined (6,45). However, a recent systematic review and meta-analysis found no significant difference between the success rates of laparoscopic or robotic-assisted pyeloplasty; while the operating time and overall complication rate were significantly higher for robotic-assisted procedure (17).
Lastly, oncological pediatric surgery deserves a special mention about RAS. Undoubtedly, RAS is rare in pediatric oncology due to the rarity of pediatric tumors, and the principal matter of debate remains whether the fundamental oncological principles of no spillage and total resection of the margins can be fulfilled with the use of RAS (6,15). Consequently, robotic surgery for pediatric tumors is considered a safe option only in highly selected cases (19).
However, the current review was judged to be at low or moderate risk of bias for all domains according to the ROBINS-I assessment tool (46). Further studies with a high level of evidence are needed in the future to better define evidence-based indications for RAS in the pediatric population.
In conclusion, the future of RAS in pediatrics depends on advancing technologies and the demand for smaller robotic devices. This will probably result in a reduction of instrument size, an improvement in haptic feedback, and development of a more cost-effective surgery, in turn leading to the adoption of new robotic platforms able to work in small cavities and so extending this promising technology to infants and neonates (7,8,24,47).
Conclusions
To date the indications for RAS in the pediatric population are limited to pyeloplasty for ureteropelvic junction obstruction in older children and ureteral reimplantation according to the Lich-Gregoire technique in selected cases for the need to access the pelvis with a narrow anatomical and working space. All other indications for RAS in pediatric surgery are still under discussion to date, and cannot be supported by papers with a high level of evidence. However, RAS is a promising technology and further evidence is strongly encouraged in the future.
Acknowledgments
This work has been accepted as oral presentation at the 23rd European Paediatric Surgeons’ Association (EUPSA) Congress, 29th June–2nd July 2022, Tel Aviv-Israel.
Funding: This work was supported by the Ministry of Health, Rome-Italy, in collaboration with the Institute for Maternal and Child Health IRCCS Burlo Garofolo, Trieste-Italy.
Footnote
Reporting Checklist: The authors have completed the Narrative Review reporting checklist. Available at https://tp.amegroups.com/article/view/10.21037/tp-22-338/rc
Peer Review File: Available at https://tp.amegroups.com/article/view/10.21037/tp-22-338/prf
Conflicts of Interest: All authors have completed the ICMJE uniform disclosure form (available at https://tp.amegroups.com/article/view/10.21037/tp-22-338/coif). The authors have no conflicts of interest to declare.
Ethical Statement: The authors are accountable for all aspects of the work in ensuring that questions related to the accuracy or integrity of any part of the work are appropriately investigated and resolved.
Open Access Statement: This is an Open Access article distributed in accordance with the Creative Commons Attribution-NonCommercial-NoDerivs 4.0 International License (CC BY-NC-ND 4.0), which permits the non-commercial replication and distribution of the article with the strict proviso that no changes or edits are made and the original work is properly cited (including links to both the formal publication through the relevant DOI and the license). See: https://creativecommons.org/licenses/by-nc-nd/4.0/.
References
- Armijo PR, Pagkratis S, Boilesen E, et al. Growth in robotic-assisted procedures is from conversion of laparoscopic procedures and not from open surgeons' conversion: a study of trends and costs. Surg Endosc 2018;32:2106-13. [Crossref] [PubMed]
- Peters BS, Armijo PR, Krause C, et al. Review of emerging surgical robotic technology. Surg Endosc 2018;32:1636-55. [Crossref] [PubMed]
- Chen R, Rodrigues Armijo P, Krause C, et al. A comprehensive review of robotic surgery curriculum and training for residents, fellows, and postgraduate surgical education. Surg Endosc 2020;34:361-7. [Crossref] [PubMed]
- Azadi S, Green IC, Arnold A, et al. Robotic Surgery: The Impact of Simulation and Other Innovative Platforms on Performance and Training. J Minim Invasive Gynecol 2021;28:490-5. [Crossref] [PubMed]
- Fernandez N, Farhat WA. A Comprehensive Analysis of Robot-Assisted Surgery Uptake in the Pediatric Surgical Discipline. Front Surg 2019;6:9. [Crossref] [PubMed]
- Denning NL, Kallis MP, Prince JM. Pediatric Robotic Surgery. Surg Clin North Am 2020;100:431-43. [Crossref] [PubMed]
- Bergholz R, Botden S, Verweij J, et al. Evaluation of a new robotic-assisted laparoscopic surgical system for procedures in small cavities. J Robot Surg 2020;14:191-7. [Crossref] [PubMed]
- Asensus Surgical. Senhance®. Available online: https://www.senhance.com/us/home (accessed on 8 February 2022).
- Oxford Centre for Evidence-based Medicine - Levels of Evidence (March 2009). CEBM. 2009. Available online: http://www.cebm.net/oxford-centre-evidence-basedmedicine-levels-evidence-march-2009/. Accessed April 7, 2017.
- Burns PB, Rohrich RJ, Chung KC. The levels of evidence and their role in evidence-based medicine. Plast Reconstr Surg 2011;128:305-10. [Crossref] [PubMed]
- Cundy TP, Shetty K, Clark J, et al. The first decade of robotic surgery in children. J Pediatr Surg 2013;48:858-65. [Crossref] [PubMed]
- Cundy TP, Harling L, Marcus HJ, et al. Meta analysis of robot-assisted versus conventional laparoscopic fundoplication in children. J Pediatr Surg 2014;49:646-52. [Crossref] [PubMed]
- Mahida JB, Cooper JN, Herz D, et al. Utilization and costs associated with robotic surgery in children. J Surg Res 2015;199:169-76. [Crossref] [PubMed]
- Madadi-Sanjani O, Petersen C, Ure B. Minimally Invasive Hepatobiliary Surgery. Clin Perinatol 2017;44:805-18. [Crossref] [PubMed]
- Cave J, Clarke S. Paediatric robotic surgery. Ann R Coll Surg Engl 2018;100:18-21. [Crossref] [PubMed]
- Esposito C, Autorino G, Castagnetti M, et al. Robotics and future technical developments in pediatric urology. Semin Pediatr Surg 2021;30:151082. [Crossref] [PubMed]
- Chandrasekharam VVS, Babu R. A systematic review and meta-analysis of conventional laparoscopic versus robot-assisted laparoscopic pyeloplasty in infants. J Pediatr Urol 2021;17:502-10. [Crossref] [PubMed]
- Ferrero PA, Blanc T, Binet A, et al. The Potential and the Limitations of Esophageal Robotic Surgery in Children. Eur J Pediatr Surg 2022;32:170-6. [Crossref] [PubMed]
- Blanc T, Meignan P, Vinit N, et al. Robotic Surgery in Pediatric Oncology: Lessons Learned from the First 100 Tumors-A Nationwide Experience. Ann Surg Oncol 2022;29:1315-26. [Crossref] [PubMed]
- van Dalen EC, de Lijster MS, Leijssen LG, et al. Minimally invasive surgery versus open surgery for the treatment of solid abdominal and thoracic neoplasms in children. Cochrane Database Syst Rev 2015;1:CD008403. [Crossref] [PubMed]
- Broholm M, Onsberg Hansen I, Rosenberg J. Limited Evidence for Robot-assisted Surgery: A Systematic Review and Meta-Analysis of Randomized Controlled Trials. Surg Laparosc Endosc Percutan Tech 2016;26:117-23. [Crossref] [PubMed]
- Steyaert H, Van Der Veken E, Joyeux L. Implementation of Robotic Surgery in a Pediatric Hospital: Lessons Learned. J Laparoendosc Adv Surg Tech A 2019;29:136-40. [Crossref] [PubMed]
- Rothenberg SS. Developing neonatal minimally invasive surgery: Innovation, techniques, and helping an industry to change. J Pediatr Surg 2015;50:232-5. [Crossref] [PubMed]
- Kayser M, Krebs TF, Alkatout I, et al. Evaluation of the Versius Robotic Surgical System for Procedures in Small Cavities. Children (Basel) 2022;9:199. [Crossref] [PubMed]
- Zhen C, Xia Z, Long L, et al. Laparoscopic excision versus open excision for the treatment of choledochal cysts: a systematic review and meta-analysis. Int Surg 2015;100:115-22. [Crossref] [PubMed]
- Shen HJ, Xu M, Zhu HY, et al. Laparoscopic versus open surgery in children with choledochal cysts: a meta-analysis. Pediatr Surg Int 2015;31:529-34. [Crossref] [PubMed]
- Dagash H, Chowdhury M, Pierro A. When can I be proficient in laparoscopic surgery? A systematic review of the evidence. J Pediatr Surg 2003;38:720-4. [Crossref] [PubMed]
- Garey CL, Laituri CA, Ostlie DJ, et al. Single-incision laparoscopic surgery in children: initial single-center experience. J Pediatr Surg 2011;46:904-7. [Crossref] [PubMed]
- Lishuang M, Zhen C, Guoliang Q, et al. Laparoscopic portoenterostomy versus open portoenterostomy for the treatment of biliary atresia: a systematic review and meta-analysis of comparative studies. Pediatr Surg Int 2015;31:261-9. [Crossref] [PubMed]
- Hussain MH, Alizai N, Patel B. Outcomes of laparoscopic Kasai portoenterostomy for biliary atresia: A systematic review. J Pediatr Surg 2017;52:264-7. [Crossref] [PubMed]
- Alizai NK, Dawrant MJ, Najmaldin AS. Robot-assisted resection of choledochal cysts and hepaticojejunostomy in children. Pediatr Surg Int 2014;30:291-4. [Crossref] [PubMed]
- Cho HD, Samstein B, Chaundry S, et al. Minimally invasive donor hepatectomy, systemic review. Int J Surg 2020;82S:187-91. [Crossref] [PubMed]
- Liu R, Wakabayashi G, Kim HJ, et al. International consensus statement on robotic hepatectomy surgery in 2018. World J Gastroenterol 2019;25:1432-44. [Crossref] [PubMed]
- Yamashita S, Yoshida Y, Iwasaki A. Robotic Surgery for Thoracic Disease. Ann Thorac Cardiovasc Surg 2016;22:1-5. [Crossref] [PubMed]
- Kiblawi R, Zoeller C, Zanini A, et al. Video-Assisted Thoracoscopic or Conventional Thoracic Surgery in Infants and Children: Current Evidence. Eur J Pediatr Surg 2021;31:54-64. [Crossref] [PubMed]
- Ballouhey Q, Villemagne T, Cros J, et al. Assessment of paediatric thoracic robotic surgery. Interact Cardiovasc Thorac Surg 2015;20:300-3. [Crossref] [PubMed]
- Cundy TP, Harley SJD, Marcus HJ, et al. Global trends in paediatric robot-assisted urological surgery: a bibliometric and Progressive Scholarly Acceptance analysis. J Robot Surg 2018;12:109-15. [Crossref] [PubMed]
- Hambraeus M, Arnbjörnsson E, Anderberg M. A literature review of the outcomes after robot-assisted laparoscopic and conventional laparoscopic Nissen fundoplication for gastro-esophageal reflux disease in children. Int J Med Robot 2013;9:428-32. [Crossref] [PubMed]
- Altokhais T, Mandora H, Al-Qahtani A, et al. Robot-assisted Heller's myotomy for achalasia in children. Comput Assist Surg (Abingdon) 2016;21:127-31. [Crossref] [PubMed]
- Al-Bassam A. Robotic-assisted surgery in children: advantages and limitations. J Robot Surg 2010;4:19-22. [Crossref] [PubMed]
- Pini Prato A, Arnoldi R, Dusio MP, et al. Totally robotic soave pull-through procedure for Hirschsprung's disease: lessons learned from 11 consecutive pediatric patients. Pediatr Surg Int 2020;36:209-18. [Crossref] [PubMed]
- Nakib G, Calcaterra V, Scorletti F, et al. Robotic assisted surgery in pediatric gynecology: promising innovation in mini invasive surgical procedures. J Pediatr Adolesc Gynecol 2013;26:e5-7. [Crossref] [PubMed]
- Villanueva J, Killian M, Chaudhry R. Robotic Urologic Surgery in the Infant: a Review. Curr Urol Rep 2019;20:35. [Crossref] [PubMed]
- Mittal S, Srinivasan A. Robotics in Pediatric Urology: Evolution and the Future. Urol Clin North Am 2021;48:113-25. [Crossref] [PubMed]
- Rague JT, Shannon R, Rosoklija I, et al. Robot-assisted laparoscopic urologic surgery in infants weighing ≤10 kg: A weight stratified analysis. J Pediatr Urol 2021;17:857.e1-7. [Crossref] [PubMed]
- Sterne JA, Hernán MA, Reeves BC, et al. ROBINS-I: a tool for assessing risk of bias in non-randomised studies of interventions. BMJ 2016;355:i4919. [Crossref] [PubMed]
- Wilson M, Badani K. Competing Robotic Systems: A Preview. Urol Clin North Am 2021;48:147-50. [Crossref] [PubMed]