Fourteen cases of cerebral creatine deficiency syndrome in children: a cohort study in China
Highlight box
Key findings
• This study summarized the diagnosis, treatment, and follow-up of multiple cases (14 patients) with creatine deficiency syndrome (CCDS) in China.
• CCDS are rare conditions, our findings may improve understanding of children with developmental delay. The incidence of CCDS in children with developmental retardation in China is ~0.39%.
What is known and what is new?
• Many cases with CCDS have been reported and treated in North America and Europe and many patients were treatable.
• Fewer cases have been reported in children in China. This study was the first to conduct a long-term follow-up and report the outcomes of multiple cases with CCDS.
What is the implication, and what should change now?
• It is necessary to improve the awareness of CCDS, and metabolic/genetic analyses should be performed if CCDS is suspected.
• The long-term treatment side effects of GAMT deficiency require further investigation.
• New treatments of SLC6A8 deficiency need to be explored.
Introduction
Cerebral creatine deficiency syndromes (CCDS) are hereditary neurometabolic diseases and is characterized by reduced brain creatine (Cr) levels. There are 3 types: (I) CCDS1: creatine transporter deficiency (CTD) or SLC6A8 deficiency; and two the biosynthesis disorders; (II) CCDS2: guanidinoacetate methyltransferase (GAMT) deficiency; and (III) CCDS3: L-arginine:glycine amidinotransferase (AGAT) deficiency. Patients have mild-severe global developmental delay, characterized by intellectual impairment, hypotonia, speech delay, behavioral disorders, and epilepsy from early childhood. In 1994 (1), the first case of CCDS2 was described in a 22-month-old infant, who presented with hypotonia and movement disorders. Since then, >300 cases of CCDS have been reported in newborns, children, and adults and the results of treatment. In children, the early development delays in CCDS and other disorders are nonspecific and similar in presentation. But CCDS could be differentiated from other diseases through in vivo metabolites and genetic analysis (2-11).
Few cases of CCDS have been found in China due to the lack of testing equipment in many hospitals. So many clinicians know little about CCDS and have very limited treatment experience, or think that there was no treatment for CCDS, just like other genetic diseases. Several cases had not been diagnosed for a long time until they came to Children’s Hospital of Fudan University, so some patients may be missed. In this study, a cohort of 3,586 patients with developmental delay, evaluated by Gesell Developmental Scale in Department of Child Care and Neurology at the Children’s Hospital of Fudan University (from January 2017–December 2022) was screened for CCDS. Cr and guanidinoacetate (GAA) levels on dried blood spots (DBS), the Cr/creatinine (Cr/Crn) ratio in the urine, and Cr levels in the brain were measured by liquid chromatography-tandem mass spectrometry (LC-MS/MS) and magnetic resonance spectroscopy (MRS). Genetic analysis was done by next-generation sequencing (NGS). We would like to know the incidence of CCDS in Chinese children with developmental delay, and summarize our treatment results and provide some experience for clinicians. We present this article in accordance with the STROBE reporting checklist (available at https://tp.amegroups.com/article/view/10.21037/tp-23-164/rc).
Methods
Children diagnosed with developmental delay, intellectual disability, epilepsy, behavior and movement disorders, and speech and language disorders in the last 5 years at the Genetic Metabolic Diseases Laboratory and Molecular Central Laboratory, Department of Radiology of Children’s Hospital were included in this study. The physicians following these patients were invited to participate in this study. This study was approved by the Medical Research Ethics Committee (No. 2015-130) at Pediatric Research Institution of Children’s Hospital of Fudan University. The parents/guardians of the included patients provided written informed consent. The study was conducted in accordance with the Declaration of Helsinki (as revised in 2013).
Metabolic and genetic screening
Cr and GAA levels on DBS and the Cr/Crn ratio in the urine both were measured by LC-MS/MS (Acquity UHPLC-class Xevo TQD, Waters, USA) (8,9). The peripheral blood of the patients with suspected CCDS and their parents were obtained for next-generation sequencing (NGS). Genomic DNA was obtained from venous (whole) blood of the index patients and their parents and sequenced using an Illumina HiSeq 2500 sequencer (Illumina, San Diego, USA). All detected mutations were confirmed by Sanger sequencing using ABI 3500 Gene Analyzer (Applied Bio-systems, USA).
Gene sequencing, variant interpretation, and reporting were performed according to the high-throughput sequencing and diagnostic protocol established by the Molecular Diagnosis Center at Pediatric Research institution (12). New variants were analyzed by Polyphen-2 (http://genetics.bwh.harvard.edu) and Mutation Taster (www.mutationtaster.org). A comprehensive assessment of each variant’s pathogenicity, including the inheritance pattern, was carried out according to the American College of Medical Genetics and Genomics criteria (13).
Combined brain magnetic resonance imaging (MRI)/MRS examinations
Combined MRI/MRS examinations were performed with a 3.0T clinical scanner (Discovery TM MR 750 3.0T, GE Healthcare, USA). All patients sedated with chloral hydrate. Routine MRI scans to rule out unknown brain lesions. MRS Collection: T2WI was used to identify the basal ganglia, thalamus, and white matter as the area of interest. MRS Scanning uses a two-dimensional point resolution spectrum (2D-PRESS) sequence with horizontal position and the scanning time was 4.30 min. The original detection results of MRS were imported into the GEAW4.2 workstation and the Functool software was used for post-processing. The peak area of N-acetyl aspartate (NAA), choline (Cho) and Cr were measured by integral method.
Treatment and follow-up
The treatment of children with CCDS was based on previous reports (14-18). Six patients with the GAMT deficiency all received a low-protein diet (<20–25 g/day) with Cr (400 mg/kg/day), and ornithine (400–500 mg/kg/day) supplementation. And we added Addition nutritional (Cyclinex-2 and Pro-Phree, Abbott, USA) for patients <4 years old. Six patients with the SLC6A8 deficiency received Cr monotherapy, but this was discontinued after 3–6 months. Additionally, 1 patient received combined Cr (400 mg/kg/day), arginine (200 mg/kg/day), and glycine (100 mg/kg/day) therapy for 7 months, and 1 patient received combined Cr gluconate (300 mg/kg/day), arginine (200 mg/kg/day), and glycine (100 mg/kg/day) therapy for 2 months. Metabolites in the blood and urine were monitored after 1–2 months of treatment, and brain Cr levels were measured after 6–9 months of treatment.
Statistical analysis
For this study, Cr levels in the blood and the Cr/Crn ratio in the urine are expressed as the mean ± standard error of the mean, and differences in the clinical treatment outcomes and Cr levels in the brain were analyzed using the analysis of variance test for the continuous variables. All the statistical analyses were performed with GraphPad Prism 8 (GraphPad Software, San Diego, CA, USA). A P value <0.05 was considered statistically significant.
Results
In the last 6 years, 3,586 children were diagnosed with developmental delay, intellectual disability, epilepsy, behavior and movement disorders, and speech and language disorders by the Genetic Metabolic Diseases Laboratory and Molecular Central Laboratory, Department of Radiology at Children’s Hospital. Of these patients, 148 (of whom 98 were male and 50 were female) had suspected CCDS by LC-MS/MS or genetic testing at first. Ultimately, 14 patients were confirmed with CCDS by MRS examinations, of whom 6 were diagnosed with GAMT deficiency (CCDS2) and 8 were diagnosed with SLC6A8 deficiency (CCDS1). The clinical characteristics of the 14 patients diagnosed with CCDS are summarized in Table 1.
Table 1
Patients No./sex/age at diagnosis/current age | Clinical features | Biochemical indications; Ref: (Cr 80–1,000 μM, GAA 0.40–3.82 μM, Cr/Crn 0.02–0.43) | Gene | Genomic variants | Brain MRI/MRS | Diagnosis |
---|---|---|---|---|---|---|
1/F/4 y/14 y | Moderate GDD, speaks 2–3 words, occasional epilepsy, hypotonia | Cr 33.26↓↓; GAA 17.25↑ | GAMT | Known c.564G>T (p.M188I), known c.491dupG (p.Gly164Alafs*14) | Abnormal signals in the white matter/brain Cr↓↓ | CCDS2 |
2/F/20 m/4 y | Severe GDD, mild epileptic, no speech | Cr 39.45↓↓; GAA 2.84 | GAMT | Known c.289delC (p.Gln97Serfs*17), known c.392-1G>C | Extracerebral space enlargement/brain Cr↓↓ | CCDS2 |
3/F/4 y/6 y | Severe GDD, epilepsy, no speech | Cr 48.32↓↓; GAA 5.46↑ | GAMT | Novel c.328-1G>A, known c.403G>A (p.D135N) | Thin corpus callosum/ brain Cr↓↓ |
CCDS2 |
4/F/6 y/9 y | Moderate GDD, refractory epilepsy, speaks 2–3 words | Cr 52.67↓; GAA 6.04↑ | GAMT | Novel c.328-1G>A, novel c.114C>T (p.G38G) | Abnormal signals in the white matter/brain Cr↓↓ | CCDS2 |
5/M/3 y/4 y | Moderate GDD, speaks 1–2 words | Cr 42.96↓↓; GAA 7.01↑ | GAMT | Novel c.328-2A>G, novel c.115 A>G (p.K39E) | N/brain Cr↓↓ | CCDS2 |
6/M/1 y/2 y | Mild GDD, speaks 1–2 words | Cr 62.62↓; GAA 6.01↑ | GAMT | N/brain Cr↓↓ | CCDS2 | |
7/M/8 y/11 y | Severe ID, epilepsy, few teeth, speaks 2–3 words | Cr 61.23↓; Cr/Crn 1.21↑ | SLC6A8 | Known c. 626_627delCT (Pro209Argfs*87) | N/brain Cr↓↓ | CCDS1 |
8/M/2 y/4 y | Severe ID, ADHD, laryngeal cartilage dysplasia, no language, severe epilepsy. Mother: mild ID, epileptic | Cr 67.53↓; Cr/Crn 1.38↑ | SLC6A8 | Known c.200G>A (p.G67D) | Extracerebral space enlargement, thin corpus callosum/brain Cr↓↓ | CCDS1 |
9/M/7 y/9 y | Moderate ID, only speaks a few words, few teeth, deficit in fine motor skills | Cr 62.96↓; Cr/Crn 1.30↑ | SLC6A8 | Known c.778-2A>G | N/brain Cr↓↓ | CCDS1 |
10/M/2 y/4 y | Severe ID, unsteady walking, inability to speak, epilepsy, constipation, ADD. Uncle: same symptoms | Cr 45.59↓↓; Cr/Crn 1.18↑ | SLC6A8 | Known c.1222_1224delTTC (p.Phe408del) | Extracerebral space enlargement/brain Cr↓↓ | CCDSI |
11/M/1 y/2 y | Severe ID, repeated convulsions, ADHD, epilepsy | Cr 49.59↓↓; Cr/Crn 1.24↑ | SLC6A8 | Novel c.1767+1_1767+2insA | Cerebral atrophy/brain Cr↓↓ | CCDSI |
12/M/3 y/5 y | Severe GDD, hypotonia, ADD, intractable epilepsy, | Cr 55.20↓; Cr/Crn 1.13↑ | SLC6A8 | Known c.321_323 del (p.F107del) | N/brain Cr↓↓ | CCDS1 |
13/M/2 y/4 y | Severe ID, open mouth, drooling, difficulty falling asleep, crying at night, sweating, white hair | Cr 55.20↓; Cr/Crn 3.45↑ | SLC6A8 | Novel c.1496G>A (p.G499V) | Thin corpus callosum, Abnormal signals in the white matter/brain Cr↓↓ | CCDS1 |
14/M/9 m/4 y | Moderate ID, little eye contact | Cr 47.53↓↓; Cr/Crn 2.62↑ | SLC6A8 | Novel c. 967G>C (p.A323P) | N/brain Cr ↓↓ | CCDS1 |
↑, increased; ↓, reduced; ↓↓, significantly reduced; Patients 5 and 6 are brothers. CCDS, cerebral creatine deficiency syndrome; F, female; M, male; y, years; m, months; GDD, global developmental delay; ID, intellectual disability; ADHD, attention deficit hyperactivity disorder; ADD, attention deficit disorder; Cr, creatine; GAA, guanidinoacetic acid; Crn, creatinine; MRI, magnetic resonance imaging; MRS, magnetic resonance spectroscopy; N, normal.
Metabolic screening and genetic testing
All the patients with suspected CCDS had a Cr level from DBS below <85 µM/L on DBS. During the follow-up, the Cr level decreased in 17 patients, the GAA from DBS level increased in 6 patients, and the Cr/Crn ratio from urine increased in 8 patients by LC-MS/MS. Seven patients with suspected CCDS had compound heterozygous variants in the GAMT gene, suggesting the possibility of GATM deficiency. In total, 10 patients had hemizygous variants of the SLC6A8 gene, suggesting the possibility of SLC6A8 deficiency, among whom, 6 had inherited the mutations from their mother, and 4 had spontaneous mutations.
Brain MRI/MRS examinations
The brain MRI showed that 3 patients (Patients 3, 8, 13) with suspected CCDS had a thin corpus callosum, 3 (Patients 1, 4, 13) had abnormalities in the white matter, 3 (Patients 2, 8, 10) extracerebral space enlargement, and 1 had cerebral atrophy (Patient 11). While 6 patients showed no abnormalities (Table 1). The brain MRS confirmed that the Cr peaks in the basal ganglia and thalamus were below the normative values in 13 patients and moderately reduced in 1 patient (Figure 1). One patient with variants in GAMT gene and 2 patients with variants in SLC6A8 gene were excluded from CCDS, as brain Cr levels were normal by MRS. All cranial MRI/MRS results from Department of Radiology in Children’s Hospital of Fudan University.
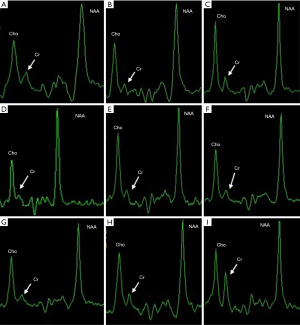
Treatment outcomes
The DBS results showed that the Cr and GAA levels of the patients diagnosed with CCDS2 returned to the normal range after 2–3 weeks of treatment. Brain Cr levels increased significantly 6–9 months after treatment, returning to 50–80% of normal. The clinical manifestations of a majority of the patients improved. In 3 patients (Patients 1, 3, and 4), epilepsy gradually resolved after 3–6 months of treatment, and the patients no longer required anti-epileptic drugs. Patient 1, now aged 14 years, was treated for 10 years and went to school normally, and she performed as well intellectually normal children. Patient 2 made progress in communication and simple conversations but was not able to speak long sentences after 3 years of treatment. This patient had occasional absent-mindedness and some abnormalities on electroencephalogram, which showed significant improvement with the use of anti-epileptic drugs (sodium valproate). The other 4 patients with CCDS2 were treated for 4–8 months, and their motor and cognitive skills improved (could walk, run, and climb stairs, gross motor and fine motor progress. significant progress in understanding and communication, like read children’s books, start to speak), but their speech was relatively slow.
Treatment was not effective for patients diagnosed with CCDS1. Among these patients, 6 received Cr monotherapy for 6 months, and their clinical manifestations did not improve, 1 patient received Cr monotherapy for 6 months, followed by combined Cr, arginine and glycine therapy for 2 months and showed some improvements, including the ability to walk more steadily, a greater willingness to learn, and less crying, and 1 patient received combined Cr gluconate, arginine and glycine therapy for 2 months, and showed modest improvements in behavior, social interactions and strength. The treatment and outcomes of the 14 CCDS patients are summarized in Table 2.
Table 2
Cases No./sex/age at treatment | Treatment [dose mg/kg/d] | Treatment duration | Outcome/improvement on treatment | Metabolites on treatment | Brain Cr level on treatment |
---|---|---|---|---|---|
1/F/4 y | Low protein diet, Cr [400], Orn [300–400], Cyclinex-2 and Pro-Phreea | 10 y 4 m | Significant progress in movement, language, and social interactions. Epilepsy disappeared after 2 years. Normal neurodevelopmental outcome | Normal Cr and GAA levels | 70–80% of normal |
2/F/20 m | Low protein diet, Cr [400], Orn [300–400], Cyclinex-2 and Pro-Phreea | 2 y 2 m | Significant progress in movement and social interactions. Ability to speak 3–4 words. Mild epilepsy that could be controlled | Normal Cr and GAA levels | 70–80% of normal |
3/F/4 y | Low protein diet, Cr [400], Orn [300–400] | 2 y 1 m | Progress in movement and social interaction. Ability to speak 3–4 words. Epilepsy disappeared after 1 year | Normal Cr and GAA levels | 50–60% of normal |
4/F/6 y | Low protein diet, Cr [400], Orn [300–400] | 3 y 3 m | Significant progress in movement and social interactions, Ability to speak 7–8 words. Epilepsy disappeared after 2 years | Normal Cr and GAA levels | 50–60% of normal |
5/M/3 y | Low protein diet, Cr [400], Orn [300–400] | 7 m | Progress in movement, language, and social interactions | Normal Cr and GAA levels | 50–60% of normal |
6/M/1 y | Low protein diet, Cr [400], Orn [300–400] | 8 m | Progress in movement, language, and social interactions | Normal Cr and GAA levels | 50–60% of normal |
7/M/8 y | Cr [400] | 6 m | None | Cr/Crn 0.40 | Not performed |
8/M/2 y | Cr [400] | 6 m | None | Cr/Crn 0.35 | Not performed |
9/M/7 y | Cr [400] | 6 m | None | Cr/Crn 0.43 | No change |
10/M/2 y | Cr [400] | 6 m | None | Cr/Crn 0.46 | No change |
11/M/1 y | Cr [400] | 4 m | None | Cr/Crn 032 | No change |
12/M/3 y | Cr [400] | 5 m | None | Cr/Crn 0.41 | Not performed |
13/M/2 y | Cr [400], Gly [150], Arg [300], creatine gluconate [400] | 2 m | Improvement in some motor skills, cognition and hand behavior | Cr/Crn 0.23 | Slightly increased but still very low |
14/M/9 m | Cr [400], Gly [150], Arg [300], creatine gluconate [400] | 3 m | Improvement in motor skills and cognition | Cr/Crn 0.37 | Slightly increased but still very low |
a, when <5 years old. CCDS, cerebral creatine deficiency syndrome; F, female; M, male; y, years; m, months; d, day; Cr, creatine; Orn, ornithine; Gly, glycine; Arg, arginine; Crn, creatinine; Cr/Crn in urine, (normal 0.02–0.43); GAA, guanidinoacetate.
Discussion
Cr/creatine-phosphate is an electrical buffer system of chemical energy in brain and muscle tissues/cells, which plays an important role in energy storage and conversion. In vivo Cr is synthesized mainly in the liver, pancreas and kidneys by AGAT and GAMT in two steps. The former enzymes catalyzed the formation of GAA from arginine and glycine, and the latter catalyzed the conversion of GAA into Cr. Cr is transported to the brain and muscle tissue by Cr transporters in the blood. Studies show that Cr synthesis or transporter deficiency causes severe Cr deficiency in brain and leads to a series of severe neurological disorders (1,14,17).
CCDS are hereditary metabolic diseases in which Cr synthesis or Cr transport disorders lead to low Cr levels in brain. Patients with CCDS2 have also elevated GAA levels, and patients with CCDS1 have also an elevated urinary Cr/Crn ratio. Cr is an energy-rich amino acid derivative that is produced endogenously in several human tissues. Glycine and arginine combine to form GAA, which is methylated to Cr under the catalysis of GAMT. Cr may also be obtained from the dietary consumption of meat and fish. Cr is transported through the blood-brain barrier via a Cr transporter protein. Cr maintains adenosine triphosphate (ATP) levels in the body through reversible phosphorylation. Cr and Cr-phosphate play an important role in energy homeostasis, especially in the brain. Cr deficiency causes global developmental delay, resulting in language impairment, motor and mental disorders, epilepsy, and autism (19-21). Secondary GAA increases inhibit mitochondrial respiratory chain enzymes and Cr kinase, reduces antioxidant and ATP levels, increases inflammatory factors, and results in the brain abnormalities (22,23).
Brain MRS can detect several important metabolites in the brain, including lactate, Cr, NAA, and Cho. A significant decrease in the Cr peak alone in the brain is highly suggestive of CCDS (1,10,11). MRS also provides a non-invasive tool to evaluate the degree of cerebral Cr recovery in the follow-up of children. LC-MS/MS could be used to screen blood/urine for metabolite deficiencies to identify patients with suspected CCDS (8,9). Genetic testing should be used to make a definitive diagnosis and MRS can confirm that a variant is pathogenic as a functional test. CCDS1 could also be confirmed by an in vitro fibrocyte Cr uptake assay (24), but this is costly, time consuming, and only available in specialized laboratories.
The outcomes of patients with CCDS2 appear to improve if they receive early treatment with Cr and ornithine supplementation and a low-protein diet. Ornithine has been shown to reduce GAA synthesis and prevents brain damage, especially in infants and young children (15,16). In 2021, CCDS2 was identified among the 116 treatable inherited metabolic disorders that cause intellectual disability (25,26). More than 150 cases of CCDS2 have been reported in the literature, mostly in infants and young children. CCDS2 in an adult patient was first described in 2003, and an estimated 25 cases have been reported since then. Children with CCDS2 should receive timely treatment to reduce the risk of developing severe mental and intellectual disabilities in adulthood (5,15,16,27). In the present study, Patient 1 was diagnosed at the age of 4 years and had a normal developmental outcome after >10 years of treatment. Patients 2, 3, and 4 were treated for >1 year, and their epilepsy disappeared after 3–6 months, and they showed significant improvements in motor skills and cognition, and moderate progress in language. Patients 5 and 6 were treated for 4 months and showed noticeable progress in their global development. Patients treated displayed variable improvement, maybe it depends on the age they were treated, how long they’ve been treated, and how well they’ve followed the advice.
Approximately 20 cases of CCDS3 have been reported in the literature, but none have been reported in China. CCDS3 was first described in 2 sisters who suffered mental retardation and severe language delay (28). Early intervention with Cr supplementation has been shown to be effective in patients with CCDS3. The brain Cr level may return to 60–98% of normal if treatment begins in the neonatal period, but improvements in adult patients are limited (6,7).
CCDS1 is an X-linked Cr deficiency syndrome that was first described in a male patient with severe language delay and mild retardation (29,30). An estimated 1% of males with mental retardation of unknown etiology may have a SLC6A8 mutation. Mutations in the SLC6A8 gene occur de novo in 30% of males, and in the general population 0.024% of females are carriers. Cr supplementation may improve the phenotype in female patients with CCDS1, but treatment responses are limited in males (31-33). In the present study, Patients 13 and 14 made some progress after combined therapy. About one-third of patients could benefit by oral high-dose Cr. Studies in animals have shown that the uptake of Cr by the brain or the transport of Cr across the blood-brain barrier may be increased by pharmacochaperones, nanotechnology, changing the Cr structure, or gene therapy which has led to the identification of potential novel treatment approaches for patients with CCDS1 (34-39).
To date, 30 CCDS patients and 46 mutations of GAMT and SCL6A8 have been reported in China, including the 14 cases in this study. The number of patients with CCDS1 and CCDS2 has been reported to be 50% and 50%, respectively, but the number of patients with CCDS3 has not been reported (3,4,40-43). In this study, the frequencies of GAMT c.403G>A (5 patients) and c.491dupG (4 patients) were relatively high in Chinese patients.
It has been reported that autism spectrum disorder is associated with inborn errors of metabolism, such as CCDS (44-46). The early stages of CCDS may be difficult to detect in childhood, and diagnosis is often missed. CCDS should be suspected in patients with developmental delay, feeding difficulties/weight gain, growth retardation, and epilepsy, especially in males (47-49). Neuroimaging plays an increasingly important role in inherited metabolic diseases and other diseases, and sometimes plays a key role in differential diagnosis, such as the diagnosis of CCDS by MRS (10,11).
Thus, in addition to clinical and biochemical profiling, neuroimaging plays a pivotal role in differentiating between metabolic disorders and other diseases, in providing a differential diagnosis, in suggesting a metabolic pathway derangement, and, on occasion, in helping to make a specific diagnosis.
Conclusions
In conclusion, we described the clinical characteristics and treatment outcomes of 14 patients with CCDS. The prevalence of CCDS is about 0.39% in Chinese children with developmental delay. Children with suspected CCDS may be early diagnosed using LC-MS/MS, genetic testing and MRS. We discovered 7 novel genetic variants, and thus expanded the spectrum of CCDS. Among the 14 patients, 6 with CCDS2 made significant progress, Patient 1 had normal neurodevelopment. Combined Cr and ornithine therapy and a low-protein diet improved seizure control, cognition, and movement disorders. The clinical characteristics of the 6 patients with CCDS1 treated with Cr monotherapy did not improve, which provides further evidence that this approach is not effective in males. Two patients with CCDS1 received combined Cr/Cr gluconate, arginine, glycine therapy and showed some improvement in motor skills, hand behaviors, and cognition. Treatment responses should be monitored by MRS, and the outcomes of combined therapy should be continually observed during the long-term follow-up.
Acknowledgments
The authors thank Dr. William E. O’Brien and Dr. Qin Sun of Department of Molecular and Human Genetics, Baylor College of Medicine for their help with the mass spectrometry analysis. The authors thank Dr. V. Reid Sutton of Texas Children’s Hospital for his advice for treatment.
Funding: None.
Footnote
Reporting Checklist: The authors have completed the STROBE reporting checklist Available at https://tp.amegroups.com/article/view/10.21037/tp-23-164/rc
Data Sharing Statement: Available at https://tp.amegroups.com/article/view/10.21037/tp-23-164/dss
Peer Review File: Available at https://tp.amegroups.com/article/view/10.21037/tp-23-164/prf
Conflicts of Interest: All authors have completed the ICMJE uniform disclosure form (available at https://tp.amegroups.com/article/view/10.21037/tp-23-164/coif). The authors have no conflicts of interest to declare.
Ethical Statement: The authors are accountable for all aspects of the work in ensuring that questions related to the accuracy or integrity of any part of the work are appropriately investigated and resolved. This study was approved by the Medical Research Ethics Committee (No. 2015-130) at Pediatric Research Institution of Children’s Hospital of Fudan University. The parents/guardians of the included patients provided written informed consent.
Open Access Statement: This is an Open Access article distributed in accordance with the Creative Commons Attribution-NonCommercial-NoDerivs 4.0 International License (CC BY-NC-ND 4.0), which permits the non-commercial replication and distribution of the article with the strict proviso that no changes or edits are made and the original work is properly cited (including links to both the formal publication through the relevant DOI and the license). See: https://creativecommons.org/licenses/by-nc-nd/4.0/.
References
- Stöckler S, Holzbach U, Hanefeld F, et al. Creatine deficiency in the brain: a new, treatable inborn error of metabolism. Pediatr Res 1994;36:409-13. [Crossref] [PubMed]
- Schulze A, Hess T, Wevers R, et al. Creatine deficiency syndrome caused by guanidinoacetate methyltransferase deficiency: diagnostic tools for a new inborn error of metabolism. J Pediatr 1997;131:626-31. [Crossref] [PubMed]
- Sun W, Wang Y, Zu Z, et al. First reported Chinese case of guanidinoacetate methyltransferase deficiency in a 4-year-old child. Clin Chim Acta 2017;470:42-5. [Crossref] [PubMed]
- Shen M, Yang G, Chen Z, et al. Identification of novel variations in SLC6A8 and GAMT genes causing cerebral creatine deficiency syndrome. Clin Chim Acta 2022;532:29-36. [Crossref] [PubMed]
- Modi BP, Khan HN, van der Lee R, et al. Adult GAMT deficiency: A literature review and report of two siblings. Mol Genet Metab Rep 2021;27:100761. [Crossref] [PubMed]
- Battini R, Alessandrì MG, Leuzzi V, et al. Arginine:glycine amidinotransferase (AGAT) deficiency in a newborn: early treatment can prevent phenotypic expression of the disease. J Pediatr 2006;148:828-30. [Crossref] [PubMed]
- Battini R, Alessandrì MG, Casalini C, et al. Fifteen-year follow-up of Italian families affected by arginine glycine amidinotransferase deficiency. Orphanet J Rare Dis 2017;12:21. [Crossref] [PubMed]
- Bodamer OA, Bloesch SM, Gregg AR, et al. Analysis of guanidinoacetate and creatine by isotope dilution electrospray tandem mass spectrometry. Clin Chim Acta 2001;308:173-8. [Crossref] [PubMed]
- Benoit R, Samir M, Boutin J, et al. LC-MS/MS measurements of urinary guanidinoacetic acid and creatine: Method optimization by deleting derivatization step. Clin Chim Acta 2019;493:148-55. [Crossref] [PubMed]
- Liserre R, Pinelli L, Gasparotti R. MR spectroscopy in pediatric neuroradiology. Transl Pediatr 2021;10:1169-200. [Crossref] [PubMed]
- Bianchi MC, Tosetti M, Battini R, et al. Treatment monitoring of brain creatine deficiency syndromes: a 1H- and 31P-MR spectroscopy study. AJNR Am J Neuroradiol 2007;28:548-54. [PubMed]
- Yang L, Dong X, Peng X, et al. Evaluation of turn around time and diagnostic accuracy of the next generation sequencing data analysis pipeline version 2 of Children’s Hospital of Fudan University. Chin J Evid Based Pediatr 2018;13:118-23.
- Richards S, Aziz N, Bale S, et al. Standards and guidelines for the interpretation of sequence variants: a joint consensus recommendation of the American College of Medical Genetics and Genomics and the Association for Molecular Pathology. Genet Med 2015;17:405-24. [Crossref] [PubMed]
- Adam MPMirzaa GMPagon RA, et al. Creatine Deficiency Disorders. 1993.
- Khaikin Y, Sidky S, Abdenur J, et al. Treatment outcome of twenty-two patients with guanidinoacetate methyltransferase deficiency: An international retrospective cohort study. Eur J Paediatr Neurol 2018;22:369-79. [Crossref] [PubMed]
- Stockler-Ipsiroglu S, van Karnebeek C, Longo N, et al. Guanidinoacetate methyltransferase (GAMT) deficiency: outcomes in 48 individuals and recommendations for diagnosis, treatment and monitoring. Mol Genet Metab 2014;111:16-25. [Crossref] [PubMed]
- Dunbar M, Jaggumantri S, Sargent M, et al. Treatment of X-linked creatine transporter (SLC6A8) deficiency: systematic review of the literature and three new cases. Mol Genet Metab 2014;112:259-74. [Crossref] [PubMed]
- Bruun TUJ, Sidky S, Bandeira AO, et al. Treatment outcome of creatine transporter deficiency: international retrospective cohort study. Metab Brain Dis 2018;33:875-84. [Crossref] [PubMed]
- Stockler S, Schutz PW, Salomons GS. Cerebral creatine deficiency syndromes: clinical aspects, treatment and pathophysiology. Subcell Biochem 2007;46:149-66. [Crossref] [PubMed]
- Clark JF, Cecil KM. Diagnostic methods and recommendations for the cerebral creatine deficiency syndromes. Pediatr Res 2015;77:398-405. [Crossref] [PubMed]
- Sharer JD, Bodamer O, Longo N, et al. Laboratory diagnosis of creatine deficiency syndromes: a technical standard and guideline of the American College of Medical Genetics and Genomics. Genet Med 2017;19:256-63. [Crossref] [PubMed]
- Schulze A, Tran C, Levandovskiy V, et al. Systemic availability of guanidinoacetate affects GABAA receptor function and seizure threshold in GAMT deficient mice. Amino Acids 2016;48:2041-7. [Crossref] [PubMed]
- Marques EP, Ferreira FS, Santos TM, et al. Cross-talk between guanidinoacetate neurotoxicity, memory and possible neuroprotective role of creatine. Biochim Biophys Acta Mol Basis Dis 2019;1865:165529. [Crossref] [PubMed]
- Joncquel-Chevalier Curt M, Bout MA, Fontaine M, et al. Functional assessment of creatine transporter in control and X-linked SLC6A8-deficient fibroblasts. Mol Genet Metab 2018;123:463-71. [Crossref] [PubMed]
- Assi L, Saklawi Y, Karam PE, et al. Treatable Genetic Metabolic Epilepsies. Curr Treat Options Neurol 2017;19:30. [Crossref] [PubMed]
- Hoytema van Konijnenburg EMM, Wortmann SB, Koelewijn MJ, et al. Treatable inherited metabolic disorders causing intellectual disability: 2021 review and digital app. Orphanet J Rare Dis 2021;16:170. [Crossref] [PubMed]
- Schulze A, Bachert P, Schlemmer H, et al. Lack of creatine in muscle and brain in an adult with GAMT deficiency. Ann Neurol 2003;53:248-51. [Crossref] [PubMed]
- Item CB, Stöckler-Ipsiroglu S, Stromberger C, et al. Arginine:glycine amidinotransferase deficiency: the third inborn error of creatine metabolism in humans. Am J Hum Genet 2001;69:1127-33. [Crossref] [PubMed]
- Salomons GS, van Dooren SJ, Verhoeven NM, et al. X-linked creatine-transporter gene (SLC6A8) defect: a new creatine-deficiency syndrome. Am J Hum Genet 2001;68:1497-500. [Crossref] [PubMed]
- Cecil KM, Salomons GS, Ball WS Jr, et al. Irreversible brain creatine deficiency with elevated serum and urine creatine: a creatine transporter defect? Ann Neurol 2001;49:401-4. [Crossref] [PubMed]
- Clark AJ, Rosenberg EH, Almeida LS, et al. X-linked creatine transporter (SLC6A8) mutations in about 1% of males with mental retardation of unknown etiology. Hum Genet 2006;119:604-10. [Crossref] [PubMed]
- Rosenberg EH, Almeida LS, Kleefstra T, et al. High prevalence of SLC6A8 deficiency in X-linked mental retardation. Am J Hum Genet 2004;75:97-105. [Crossref] [PubMed]
- DesRoches CL, Patel J, Wang P, et al. Estimated carrier frequency of creatine transporter deficiency in females in the general population using functional characterization of novel missense variants in the SLC6A8 gene. Gene 2015;565:187-91. [Crossref] [PubMed]
- Jaggumantri S, Dunbar M, Edgar V, et al. Treatment of Creatine Transporter (SLC6A8) Deficiency With Oral S-Adenosyl Methionine as Adjunct to L-arginine, Glycine, and Creatine Supplements. Pediatr Neurol 2015;53:360-363.e2. [Crossref] [PubMed]
- Schjelderup J, Hope S, Vatshelle C, et al. Treatment experience in two adults with creatinfe transporter deficiency. Mol Genet Metab Rep 2021;27:100731. [Crossref] [PubMed]
- El-Kasaby A, Kasture A, Koban F, et al. Rescue by 4-phenylbutyrate of several misfolded creatine transporter-1 variants linked to the creatine transporter deficiency syndrome. Neuropharmacology 2019;161:107572. [Crossref] [PubMed]
- Ullio-Gamboa G, Udobi KC, Dezard S, et al. Dodecyl creatine ester-loaded nanoemulsion as a promising therapy for creatine transporter deficiency. Nanomedicine (Lond) 2019;14:1579-93. [Crossref] [PubMed]
- Cacciante F, Gennaro M, Sagona G, et al. Cyclocreatine treatment ameliorates the cognitive, autistic and epileptic phenotype in a mouse model of Creatine Transporter Deficiency. Sci Rep 2020;10:18361. [Crossref] [PubMed]
- Fernandes-Pires G, Braissant O. Current and potential new treatment strategies for creatine deficiency syndromes. Mol Genet Metab 2022;135:15-26. [Crossref] [PubMed]
- Wang Q, Yang J, Liu Y, et al. A novel SLC6A8 mutation associated with intellectual disabilities in a Chinese family exhibiting creatine transporter deficiency: case report. BMC Med Genet 2018;19:193. [Crossref] [PubMed]
- Sun WH, Zhuang DY, Wang Y, et al. Clinical features and SLC6A8 gene mutations of cerebral creatine deficiency syndrome I: an analysis of two families. Zhongguo Dang Dai Er Ke Za Zhi 2020;22:482-7. [PubMed]
- Sun W, Wu B, Wu M, et al. Genetic analysis and treatment for an infant with cerebral creatine deficiency syndrome type 2. Zhonghua Yi Xue Yi Chuan Xue Za Zhi 2020;37:1001-4. [PubMed]
- Shi K, Zhao H, Xu S, et al. Treatment efficacy of high-dose creatine supplementation in a child with creatine transporter (SLC6A8) deficiency. Mol Genet Genomic Med 2021;9:e1640. [Crossref] [PubMed]
- Aydin HI. Creatine Transporter Deficiency in Two Brothers with Autism Spectrum Disorder. Indian Pediatr 2018;55:67-8. [Crossref] [PubMed]
- Aydın Hİ, Sönmez FM. A novel mutation in two cousins with guanidinoacetate methyltransferase (GAMT) deficiency presented with autism. Turk J Pediatr 2019;61:92-6. [Crossref] [PubMed]
- Yıldız Y, Göçmen R, Yaramış A, et al. Creatine Transporter Deficiency Presenting as Autism Spectrum Disorder. Pediatrics 2020;146:e20193460. [Crossref] [PubMed]
- Miller JS, Thomas RP, Bennett A, et al. Early Indicators of Creatine Transporter Deficiency. J Pediatr 2019;206:283-5. [Crossref] [PubMed]
- Rostami P, Hosseinpour S, Ashrafi MR, et al. Primary creatine deficiency syndrome as a potential missed diagnosis in children with psychomotor delay and seizure: case presentation with two novel variants and literature review. Acta Neurol Belg 2020;120:511-6. [Crossref] [PubMed]
- Biswas A, Malhotra M, Mankad K, et al. Clinico-radiological phenotyping and diagnostic pathways in childhood neurometabolic disorders-a practical introductory guide. Transl Pediatr 2021;10:1201-30. [Crossref] [PubMed]
(English Language Editor: L. Huleatt)