Exploring dynamic change and influencing factors of TGF-β1 and MUC1 in human breast milk, and their association with infantile diseases
Highlight box
Key findings
• The high concentration of TGF-β1 in human breast milk was significantly associated with an increased risk of infantile diarrhea and upper respiratory infection (URI).
What is known and what is new?
• Increase TGF-β1 levels in human breast milk was reported to play a role in preventing infants’ allergic disease. However, few studies were reported on the association between TGF-β1 and other infants’ diseases.
• We reported that the high concentration of TGF-β1 in colostrum was associated with a significantly increased risk of diarrhea within the first 3 months after giving birth, and URI within the first 6 months after giving birth.
What is the implication, and what should change now?
• The present study reported the correlation between the TGF-β1 in human breast milk and infantile diarrhea and URI, which might help to optimize the infant formula.
Introduction
Human breast milk is considered to be the optimal food for infants (1). Apart from providing a source of nutrients suitable for infants’ digestion and absorption, human breast milk also contains numerous bioactive components, including immunoglobulins, cytokines, antioxidants, hormones, oligosaccharides, lactoferrin, lysozyme, epidermal growth factors, nucleotides, vitamins, defensin, and bacterial constituents. These bioactive compositions are thought to provide necessary compensation for immature neonatal immune response, and inhibit translocation of pathogenic organism across the gastrointestinal tract (1-3). Identifying components in breast milk, their influencing factors, and their effects on infants in short- and long-term outcomes would help to promote breastfeeding and optimize infant formula.
Transforming growth factor beta (TGF-β), which has three isoforms (β1–β3), is recognized as a pleiotropic cytokine with multiple functions in immunity (4). It could not only regulate immune responses against pathogens, but also play an important role in maintaining immune tolerance against self- and benign antigens at steady state (5,6). Besides, it has been reported to be involved in keeping the integrity and homeostasis of respiratory epithelium and mucosa (7). TGF-β2 is the predominant isoform of TGF-β in human breast milk (8). TGF-β1, which is thought to be mostly implicated in immune regulation, was also reported in human breast milk. Some evidence has shown that TGF-β1 and TGF-β2 concentrations in breast milk were negatively associated with the development of eczema in infants (9,10). In animal models, orally administered TGF-β1 was shown to be biologically active in intestinal mucosa and enhance oral tolerance (11,12). However, few studies have reported on the association between TGF-β1 and other infantile diseases.
Studies in vitro have suggested that mucin 1 (MUC1) acted as a modulator of TGF-β signaling in pancreatic ductal adenocarcinoma (13,14). MUC1, which is derived from the maternal plasma membrane, is one of the major components of the human milk fat globule membrane (MFGM) (15). MUC1 is a multi-functional protein, whose most important function is to provide protection against infections in infants, including human immunodeficiency virus (HIV), rotavirus, and salmonella enterica serovar typhimurium (16-18). It has also been reported to play a role in regulating inflammatory response, T cell response and toll-like receptor signaling (19-21). In recent years, MUC1 is recognized as a potential target in cancer treatment, because it acts as an oncoprotein with a key role in cancer progression due to its abnormal glycosylation (22). In human breast milk, it has been reported that majority of mucins are present in MFGM with a minority in skim milk (23), but few studies have quantified MUC1 in human breast milk (24). More studies are still needed to determine its concentration and its effects on infants.
In the present study, we aimed to determine the concentration of TGF-β1 and MUC1 in human breast milk, identify their influencing factors and their association with infantile diseases, which might help to better understand their significance for infants. We present this article in accordance with the MDAR reporting checklist (available at https://tp.amegroups.com/article/view/10.21037/tp-22-469/rc).
Methods
Subjects and data collection
From September 2018 to March 2020, a total of 130 healthy mother-infant pairs from Shanghai Punan Hospital, Shanghai, China, participated in this study. All subjects were Han Chinese. Ninety mother-infant pairs who met the inclusion criteria were enrolled in the present study: (I) mothers in good health without a history of chronic diseases, acute infections, or pregnancy-related complications during gestation and lactation; (II) healthy infants of the healthy mothers who passed the physical examination. Forty mother-infant pairs were excluded because of incomplete demographic and clinical information or refusal to participate (Figure 1). The study was performed in accordance with the Declaration of Helsinki (as revised in 2013), and approved by the Ethical Committee of Shanghai Children’s Medical Center (No. SCMCIRB-K2017047). Written informed consent was obtained from all participating mothers and the parents or guardians of the infants.
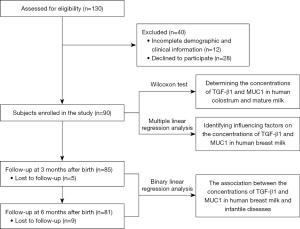
Clinical information of the mother-infant pairs was collected from their medical records. Maternal clinical information included maternal allergic history, pre-pregnancy body mass index (BMI), prenatal BMI, post-partum BMI, blood glucose levels, and serum total protein levels. Infants’ clinical information included gender, gestational age and birth weight. Questionnaires were used to collect the mother’s demographic information, including the mothers’ age, height, mode of delivery, parity.
At 3 and 6 months after giving birth, infants were followed up using telephone interviews. The incidence of eczema, upper respiratory infection (URI), and diarrhea, which were the most common infantile diseases, was recorded to evaluate their association with the concentrations of TGF-β1 and MUC1 in human breast milk.
Sample collection
The colostrum and mature milk were collected from healthy mothers within 5 days and approximately 42 days after delivery, respectively. The breast milk samples were collected by taking all the milk from one breast at a time, and then transferred to the laboratory on ice immediately. After removal of the lipid layer, the aqueous fraction was transferred into a tube. The whole breast milk, lipid layer and aqueous fractions were stored at −80 ℃ before use.
Enzyme linked immunosorbent assay (ELISA)
The concentration of TGF-β1 in aqueous fraction of human breast milk was determined by commercial ELISA kit (R&D systems) according to the manufacturer’s instructions. For MUC1, the whole breast milk was first lysed by a naïve lysis buffer (Abcam, ab156035) according to the previous report (25), then the concentration of MUC1 was determined by a commercial ELISA kit (Invitrogen) according to the manufacturer’s instructions.
Statistical analysis
Differences in the concentrations of TGF-β1 and MUC1 in paired colostrum and mature milk were evaluated by Wilcoxon test. Correlation analysis on the concentration of TGF-β1 and MUC1 in breast milk and the subjects’ characteristics was performed by spearman’s (comparing the relationship between ordinal, or rank-ordered, variables) or point-biserial (comparing the relationship between two variables if one of the variables is dichotomous) correlation analysis. Multiple linear regression analysis was used to identify the influencing factors of subjects’ characteristics on breast milk composition. Binary linear regression analysis was used to determine the association between TGF-β1 and MUC1 in human breast milk and infantile diseases. Data analyses were performed by using SPSS 26.0 software, and a P value less than 0.05 was considered to be statistically significant.
Results
Characteristics of the subjects
Ninety paired mother–infants were enrolled in this study. As shown in Table 1, the mean maternal age was 29.63±4.77 years (range, 20.79–43.25 years), the mean maternal blood glucose was 4.41±0.60 mmol/L (range, 3.1–5.8 mmol/L), and the mean maternal serum total protein level was 59.62±5.24 g/L (range, 49.41–76.49 g/L). The mean gestational age of the infants was 39.32±1.08 weeks (range, 36.57–41 weeks), with 3 cases ≤37 weeks, and the mean birth weight of the infants was 3,345.36±415.03 g (range: 2,435–4,755 g).
Table 1
Factors | Value (n=90) |
---|---|
Maternal characteristics | |
Age (years) | 29.63±4.77 |
Mode of delivery | |
Vaginal | 36 (40.0) |
Caesarean | 54 (60.0) |
Parity | |
1 | 53 (58.9) |
2 | 35 (38.9) |
3 | 2 (2.2) |
History of allergy | |
No | 83 (92.22) |
Yes | 7 (7.78) |
Blood glucose (mmol/L) | 4.41±0.60 |
Serum total protein (g/L) | 59.62±5.24 |
Pre-pregnancy BMI (kg/m2) | 21.22±3.26 |
Prenatal BMI (kg/m2) | 26.89±3.94 |
Post-partum BMI (kg/m2) | 23.72±4.65 |
Infantile characteristics | |
Gender | |
Male | 47 (52.22) |
Female | 43 (47.78) |
Gestational age (weeks) | 39.32±1.08 |
Birth weight (g) | 3,345.36±415.03 |
Data are given as n (%) or mean ± standard deviation. BMI, body mass index.
The concentrations of TGF-β1 and MUC1 in human breast milk
The median concentration of TGF-β1 was 2,706 pg/mL [interquartile range (IQR): 1,569–4,191 pg/mL] in colostrum, and 316 pg/mL (IQR: 236–880 pg/mL) in mature milk (Figure 2A). The median concentration of MUC1 was 2,103 U/mL (IQR: 884–3,720 U/mL) in colostrum, and 550 U/mL (IQR: 381–834 U/mL) in mature milk (Figure 2B). The concentration of TGF-β1 and MUC1 were both significantly higher in colostrum than in mature milk (Z=−6.941, P<0.0001, and Z=−6.602, P<0.0001, respectively). Therefore, these results showed a dynamic change of TGF-β1 and MUC1 in human breast milk over the course of lactation.
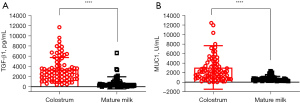
Influencing factors on TGF-β1 and MUC1 concentrations in human milk
In order to determine the influence of subjects’ characteristics on the concentrations of TGF-β1 and MUC1 in human milk, we evaluated the correlation between the subjects’ characteristics and the concentrations of TGF-β1 and MUC1. As shown in Table S1, the maternal age and caesarean delivery were significantly positively correlated with TGF-β1 concentration in colostrum (rs=0.393, P=0.0002, and r=0.230, P=0.0329, respectively). However, neither the concentration of MUC1 in colostrum, nor the concentrations of TGF-β1 and MUC1 in mature milk, was observed to correlate with the subjects’ characteristics.
Thereafter, factors in the above correlation analysis with P<0.2 were selected, and a multiple linear regression analysis with backward selection (threshold, P<0.05) was further applied to evaluate the influential variables of subjects’ characteristics on the concentrations of TGF-β1 and MUC1 in human milk. The results showed that, an advanced maternal age was associated with a significantly increased TGF-β1 concentration in colostrum, and caesarean delivery was associated with a significantly increased MUC1 concentration in colostrum (Table 2). However, no subjects’ characteristics was identified to have significant association with the concentrations of TGF-β1 and MUC1 in mature milk.
Table 2
Variables | Factors | β | P value | 95% CI for β | |
---|---|---|---|---|---|
Lower bound | Upper bound | ||||
Log (TGF-β1) | Maternal age | 0.3559 | 0.0007 | 0.0100 | 0.0363 |
Log (MUC1) | Delivery mode | 0.2538 | 0.0224 | 0.0436 | 0.5518 |
TGF-β1, transforming growth factor beta 1; MUC1, mucin 1; β, standardized coefficients; CI, confidence interval.
The correlation between the concentrations of TGF-β1 and MUC1 in human breast milk and the infantile disease within the first 6 months after giving birth
In the follow-up study during the first 3 months after giving birth, 5 (5.56%) mother-infant pairs were lost to follow-up. Among the 85 infants whose follow-up data were available, 63 (74.12%) were exclusively breastfed, 18 (21.18%) were mixed-fed, and 4 (4.71%) were formula-fed. Twenty-five (29.41%) infants were reported to suffer from eczema, 9 (10.59%) from URI, and 7 (8.24%) from diarrhea. In the follow-up study during the first 6 months after giving birth, 9 (10%) mother-infant pairs were lost to follow-up. Among the 81 infants whose follow-up data were available, 49 (60.49%) were exclusively breastfed, 12 (14.81%) were mixed-fed, and 20 (24.69%) were formula-fed. Twenty-one (25.93%) infants were reported to suffer from eczema, 23 (28.40%) from URI, and 7 (8.64%) from diarrhea.
To investigate the association between the concentrations of TGF-β1 and MUC1 in human breast milk and infantile disease, we first compared their concentrations in breast milk fed to infants with and without infantile diseases. It was shown that the concentration of TGF-β1 in colostrum was significantly higher in the infants with diarrhea within the first 3 months after giving birth (P<0.023), as well as in the infants with URI and diarrhea within the first 6 months after giving birth (P<0.035 and P<0.037, respectively).
Subsequently, a further binary logistic regression analysis with backward selection was applied to evaluate the association between the concentrations of TGF-β1 and MUC1 in human breast milk and infantile diseases within the first 6 months after giving birth. TGF-β1 and MUC1 in colostrum and mature milk were divided into high- and low-groups based on their median concentrations. Maternal allergy history and infant feeding patterns were also included in the binary logistic regression analysis, because both of them were reported to play a vital role in the development of infantile diseases. The results showed that, the high concentration of TGF-β1 in colostrum was associated with a significantly higher risk of diarrhea within the first 3 months after giving birth (Table 3), and URI within the first 6 months after giving birth (Table 4).
Table 3
Variables | OR (95% CI) | P value |
---|---|---|
TGF-β1 | 0.046 | |
Low group | 1 | |
High group | 9.964 (1.039–95.576) | |
MUC1 | 0.056 | |
Low group | 1 | |
High group | 0.110 (0.011–1.0612) |
TGF-β1, transforming growth factor beta 1; MUC1, mucin 1; OR, odd ratio; CI, confidence interval.
Table 4
Variables | OR (95% CI) | P value |
---|---|---|
TGF-β1 | 0.016 | |
Low group | 1 | |
High group | 3.888 (1.287–11.751) |
TGF-β1, transforming growth factor beta 1; OR, odd ratio; CI, confidence interval.
Discussion
Compared with formula-feeding, breast-feeding has been well-demonstrated to be beneficial to infants in both short- and long-term outcomes, and many bioactive components were reported to contribute to these favorable outcomes (2,3,26). In this study, we aimed to determine the concentrations of TGF-β1 and MUC1 in human colostrum and mature milk, their influencing factors, and their association with infantile diseases. Three major results were observed: (I) the concentrations of TGF-β1 and MUC1 in human colostrum and mature milk were different, and their concentration were significantly higher in colostrum than in mature milk; (II) an advanced maternal age was associated with a significantly increased TGF-β1 concentration in colostrum, and caesarean delivery was associated with a significantly increased MUC1 concentration in colostrum; (III) high concentration of TGF-β1 in colostrum was significantly associated with a higher risk of diarrhea within the first 3 months after giving birth, and URI within the first 6 months after giving birth.
The concentrations of bioactive components in human breast milk are dynamically changed over the course of lactation, and maternal and infants’ characteristics were shown to have influence on them (1,2). Evidence showed that concentration of TGF-β1 in human breast milk was significantly different among different countries (27,28). A previous cohort study on Chinese population showed that the median concentration of TGF-β1 was 1,305 pg/mL in colostrum within 5–11 days after giving birth, but sharply decreased to 356 pg/mL in mature milk within 1–2 months after giving birth (29). Our results showed a similar concentration of TGF-β1 in mature milk (median: 316 pg/mL), but a higher concentration (median: 2,706 pg/mL) in colostrum within 5 days after giving birth compared with that within 5–11 days (29). This result suggests that the concentration of TGF-β1 might be decreased rapidly with prolonged lactation, especially in the early stage of lactation.
MUC1 is a membrane-bound protein, whose extracellular glycosylated structure is linked to a short cytoplasmic tail by a disulfide bond. The extracellular domain contains a variable number of 20 tandem amino acid repeats with high threonine and serine content, which is named as the variable number tandem repeat (VNTR) domain. The VNTR domain of MUC1 are highly glycosylated, further contributing to its adherent and mucinous properties (30-32). The CA15-3 assay, which could detect a non-glycosylated 8-amino acid sequence (DTRPAPGS) in the VNTR domain, is an available commercial assay that determine the concentrations of MUC1 through DF3 antibody (33). To date, however, there was no studies using this approach to quantify MUC1 in breast milk. Of note, it was reported that more than half of MUC1 is present in the MFGM (cream fractions) and the rest in the skimmed milk (23). Due to its association with the MFGM, it is difficult to release MUC1 from the MFGM unless the membrane is lysed. Therefore, to make MUC1 released from the MFGM, the whole human breast milk was lysed by using a naive lysis buffer before the CA15-3 ELISA assay, according to a previous report (25). The results showed that the concentrations of MUC1 was also different in human colostrum and mature milk. As far as we know, this is the first study to measure the concentration of MUC1 in human breast milk by using CA15-3 ELISA assay, and more studies are needed to determine the concentrations of MUC1 in human breast milk from different countries and ethnicities.
Many components in human breast milk, such as immunoglobulins, cytokines, lactoferrin, and defensin, have been reported to be affected by various factors, including the country of origin, maternal diet, living environment, mode of delivery, etc. (27,34,35). These constituents in human milk could also change in response to active infection in nursing infants. Affolter et al. reported that the mode of delivery was an influencing factor on the TGF-β1 concentration in breast milk (29). Alsharnoubi et al. showed that maternal diet, infants’ age and weight could influence the TGF-β1 concentration in breast milk (36). In this study, we showed that advanced maternal age was associated with a significantly higher TGF-β1 concentration in colostrum. In addition, we showed that the concentration of MUC1 was influenced by the mode of delivery, and caesarean delivery was significantly associated with an increased MUC1 concentration in colostrum. Peterson et al. showed that there was a significant difference in the concentration of MUC1 in breast milk of full-term and preterm birth (24). These data suggested that the concentration of TGF-β1 and MUC1 in human breast milk might be influenced by a combination of physiological and environmental factors.
As an anti-inflammatory cytokine, evidence shows that the TGF-β1 concentration in breast milk is associated with the development of eczema in infants (9). Previous studies have shown that TGF-β is abundant in the mammalian intestine, among which TGF-β1 is the most abundant subtype, and its function in intestinal immunity has been well demonstrated. TGF-β1 plays an important role in inflammatory bowel disease, which acts a pivotal regulator of immune cells, epithelium, and intestinal microbiota (7,37). Elevated serum TGF-β levels were observed in patients with active inflammatory bowel disease (IBD) (7). These results were consistent with our observation that TGF-β1 concentration in human colostrum was positively associated with infantile diarrhea within 3 months after giving birth. More interestingly, we also found that TGF-β1 concentration in human colostrum was also positively associated with infantile URI within 6 months after giving birth. It might be due to the immunosuppressive effects of TGF-β1, which weakens the resistance or immunity to many infectious diseases (38).
Conclusions
In conclusion, we determined the concentrations of TGF-β1 and MUC1 in human colostrum and mature milk, identified their influencing factors, as well as their association with three infantile diseases. We for the first time showed that the high concentration of TGF-β1 in human breast milk was associated with a significantly higher risk of infantile diarrhea and URI, which helps to give a better understanding of relationship between the TGF-β1 in human breast milk and infantile diseases.
Acknowledgments
We thank all the study participants.
Funding: The study was funded by Mead Johnson & Company, LLC.
Footnote
Reporting Checklist: The authors have completed the MDAR reporting checklist. Available at https://tp.amegroups.com/article/view/10.21037/tp-22-469/rc
Data Sharing Statement: Available at https://tp.amegroups.com/article/view/10.21037/tp-22-469/dss
Peer Review File: Available at https://tp.amegroups.com/article/view/10.21037/tp-22-469/prf
Conflicts of Interest: All authors have completed the ICMJE uniform disclosure form (available at https://tp.amegroups.com/article/view/10.21037/tp-22-469/coif). The authors report the funding from Mead Johnson & Company, LLC. The authors have no other conflicts of interest to declare.
Ethical Statement: The authors are accountable for all aspects of the work and in ensuring that questions related to the accuracy or integrity of any part of the work are appropriately investigated and resolved. The study was conducted in accordance with the Declaration of Helsinki (as revised in 2013). The study was approved by the Ethical Committee of Shanghai Children’s Medical Center (No. SCMCIRB-K2017047). Written informed consent was obtained from all participating mothers and the parents or legal guardians of the infants.
Open Access Statement: This is an Open Access article distributed in accordance with the Creative Commons Attribution-NonCommercial-NoDerivs 4.0 International License (CC BY-NC-ND 4.0), which permits the non-commercial replication and distribution of the article with the strict proviso that no changes or edits are made and the original work is properly cited (including links to both the formal publication through the relevant DOI and the license). See: https://creativecommons.org/licenses/by-nc-nd/4.0/.
References
- Ballard O, Morrow AL. Human milk composition: nutrients and bioactive factors. Pediatr Clin North Am 2013;60:49-74. [Crossref] [PubMed]
- Andreas NJ, Kampmann B, Mehring Le-Doare K. Human breast milk: A review on its composition and bioactivity. Early Hum Dev 2015;91:629-35. [Crossref] [PubMed]
- Lyons KE, Ryan CA, Dempsey EM, et al. Breast Milk, a Source of Beneficial Microbes and Associated Benefits for Infant Health. Nutrients 2020;12:1039. [Crossref] [PubMed]
- Larson C, Oronsky B, Carter CA, et al. TGF-beta: a master immune regulator. Expert Opin Ther Targets 2020;24:427-38. [Crossref] [PubMed]
- Sanjabi S, Oh SA, Li MO. Regulation of the Immune Response by TGF-β: From Conception to Autoimmunity and Infection. Cold Spring Harb Perspect Biol 2017;9:a022236. [Crossref] [PubMed]
- Travis MA, Sheppard D. TGF-β activation and function in immunity. Annu Rev Immunol 2014;32:51-82. [Crossref] [PubMed]
- Ihara S, Hirata Y, Koike K. TGF-β in inflammatory bowel disease: a key regulator of immune cells, epithelium, and the intestinal microbiota. J Gastroenterol 2017;52:777-87. [Crossref] [PubMed]
- Penttila IA. Milk-derived transforming growth factor-beta and the infant immune response. J Pediatr 2010;156:S21-5. [Crossref] [PubMed]
- Morita Y, Campos-Alberto E, Yamaide F, et al. TGF-β Concentration in Breast Milk is Associated With the Development of Eczema in Infants. Front Pediatr 2018;6:162. [Crossref] [PubMed]
- Oddy WH, Rosales F. A systematic review of the importance of milk TGF-beta on immunological outcomes in the infant and young child. Pediatr Allergy Immunol 2010;21:47-59. [Crossref] [PubMed]
- Saito A, Horie M, Nagase T. TGF-β Signaling in Lung Health and Disease. Int J Mol Sci 2018;19:2460. [Crossref] [PubMed]
- Ando T, Hatsushika K, Wako M, et al. Orally administered TGF-beta is biologically active in the intestinal mucosa and enhances oral tolerance. J Allergy Clin Immunol 2007;120:916-23. [Crossref] [PubMed]
- Bose M, Grover P, Sanders AJ, et al. Overexpression of MUC1 Induces Non-Canonical TGF-β Signaling in Pancreatic Ductal Adenocarcinoma. Front Cell Dev Biol 2022;10:821875. [Crossref] [PubMed]
- Grover P, Nath S, Nye MD, et al. SMAD4-independent activation of TGF-β signaling by MUC1 in a human pancreatic cancer cell line. Oncotarget 2018;9:6897-910. [Crossref] [PubMed]
- Patton S. MUC1 and MUC-X, epithelial mucins of breast and milk. Adv Exp Med Biol 2001;501:35-45. [Crossref] [PubMed]
- Mthembu Y, Lotz Z, Tyler M, et al. Purified human breast milk MUC1 and MUC4 inhibit human immunodeficiency virus. Neonatology 2014;105:211-7. [Crossref] [PubMed]
- Yolken RH, Peterson JA, Vonderfecht SL, et al. Human milk mucin inhibits rotavirus replication and prevents experimental gastroenteritis. J Clin Invest 1992;90:1984-91. [Crossref] [PubMed]
- Liu B, Yu Z, Chen C, et al. Human milk mucin 1 and mucin 4 inhibit Salmonella enterica serovar Typhimurium invasion of human intestinal epithelial cells in vitro. J Nutr 2012;142:1504-9. [Crossref] [PubMed]
- Agrawal B, Gupta N, Konowalchuk JD. MUC1 Mucin: A Putative Regulatory (Checkpoint) Molecule of T Cells. Front Immunol 2018;9:2391. [Crossref] [PubMed]
- Ueno K, Koga T, Kato K, et al. MUC1 mucin is a negative regulator of toll-like receptor signaling. Am J Respir Cell Mol Biol 2008;38:263-8. [Crossref] [PubMed]
- Ballester B, Milara J, Cortijo J. The role of mucin 1 in respiratory diseases. Eur Respir Rev 2021;30:200149. [Crossref] [PubMed]
- Nath S, Mukherjee P. MUC1: a multifaceted oncoprotein with a key role in cancer progression. Trends Mol Med 2014;20:332-42. [Crossref] [PubMed]
- Habte HH, Kotwal GJ, Lotz ZE, et al. Antiviral activity of purified human breast milk mucin. Neonatology 2007;92:96-104. [Crossref] [PubMed]
- Peterson JA, Patton S, Hamosh M. Glycoproteins of the human milk fat globule in the protection of the breast-fed infant against infections. Biol Neonate 1998;74:143-62. [Crossref] [PubMed]
- Li Y, Wu J, Chen TX. An improved ELISA method for the determination of lactadherin in human breast milk. Current Immunology 2020;40:506-9.
- Binns C, Lee M, Low WY. The Long-Term Public Health Benefits of Breastfeeding. Asia Pac J Public Health 2016;28:7-14. [Crossref] [PubMed]
- Munblit D, Treneva M, Peroni DG, et al. Colostrum and Mature Human Milk of Women from London, Moscow, and Verona: Determinants of Immune Composition. Nutrients 2016;8:695. [Crossref] [PubMed]
- Holmlund U, Amoudruz P, Johansson MA, et al. Maternal country of origin, breast milk characteristics and potential influences on immunity in offspring. Clin Exp Immunol 2010;162:500-9. [Crossref] [PubMed]
- Affolter M, Garcia-Rodenas CL, Vinyes-Pares G, et al. Temporal Changes of Protein Composition in Breast Milk of Chinese Urban Mothers and Impact of Caesarean Section Delivery. Nutrients 2016;8:504. [Crossref] [PubMed]
- Kimura T, Finn OJ. MUC1 immunotherapy is here to stay. Expert Opin Biol Ther 2013;13:35-49. [Crossref] [PubMed]
- Stroopinsky D, Kufe D, Avigan D. MUC1 in hematological malignancies. Leuk Lymphoma 2016;57:2489-98. [Crossref] [PubMed]
- Apostolopoulos V, Stojanovska L, Gargosky SE. MUC1 (CD227): a multi-tasked molecule. Cell Mol Life Sci 2015;72:4475-500. [Crossref] [PubMed]
- Vylet'al P, Kidd K, Ainsworth HC, et al. Plasma Mucin-1 (CA15-3) Levels in Autosomal Dominant Tubulointerstitial Kidney Disease due to MUC1 Mutations. Am J Nephrol 2021;52:378-87. [Crossref] [PubMed]
- Wang XF, Cao RM, Li J, et al. Identification of sociodemographic and clinical factors associated with the levels of human β-defensin-1 and human β-defensin-2 in the human milk of Han Chinese. Br J Nutr 2014;111:867-74. [Crossref] [PubMed]
- Wu J, Jin YY, Li Y, et al. Dynamic change, influencing factors, and clinical impact of cellular components in human breast milk. Pediatr Res 2023;93:1765-71. [Crossref] [PubMed]
- Alsharnoubi J, Ishaak M, Elsheikh S, et al. Transforming Growth Factor Beta-1 in Human Breast Milk and Its Correlation with Infants' Parameters. Breastfeed Med 2019;14:404-7. [Crossref] [PubMed]
- Xiao K, Cao S, Jiao L, et al. TGF-β1 protects intestinal integrity and influences Smads and MAPK signal pathways in IPEC-J2 after TNF-α challenge. Innate Immun 2017;23:276-84. [Crossref] [PubMed]
- Maizels RM. The multi-faceted roles of TGF-β in regulation of immunity to infection. Adv Immunol 2021;150:1-42. [Crossref] [PubMed]