Ruxolitinib inhibits the proliferation and induces the apoptosis of MLL-r ALL cells through inactivating JAK/STAT signaling pathway
Highlight box
Key findings
• The study provides compelling evidence that ruxolitinib is a promising agent against MLL-r leukemia cell line.
What is known and what is new?
• JAKs play a crucial role in the regulation and homeostasis of hematopoiesis and immunity, and ruxolitinib can be used to intervene in it.
• Our studies demonstrated that ruxolitinib inhibits MLL-r proliferation and induces apoptosis by the JAK/STAT pathway, revealing the effects of ruxolitinib therapy on MLL-r leukemia.
What is the implication, and what should change now?
• This is an early laboratory data showing promise in favour of use of JAK inhibitor in MLL-r ALL. However, it needs going through multiple more steps to confirm before it can be an option in clinical practice.
Introduction
Rearrangements of the histone lysine [K]-MethylTransferase 2A gene (KMT2A) gene on chromosome 11q23, as refer to the mixed-lineage leukemia rearrangement (MLL-r) gene, occurred in 10% of adult, while 5% among pediatric acute lymphoblastic leukemia (ALL) cases (1,2). Compared to non-MLL leukemia, the clinical characteristics of MLL-r-positive ALL children distinguish them from other types of leukemia, mainly due to high white blood cell counts at initial diagnosis, and insensitivity to traditional chemotherapy drugs, accompanied with a low complete remission rates, which showing a 5-year event-free survival (EFS) of 47% and an overall survival (OS) of 55%, and was much lower than the current survival rate of childhood ALL (80–90%) (3-5). Patients with MLL-r are significantly worse off than most other leukemia patients and are therefore often treated with high-risk regimens. Because there is no useful and precise treatment for these leukemias, the current reliance on high-intensity chemotherapy for MLL-r leukemias, while increasing the intensity of chemotherapy may reduce the relapse rate in children with MLL-r leukemia, there is a corresponding increase in the incidence of treatment-related leukemia and morbidity and mortality due to complications such as infection-based diseases (6). In addition, the lack of donor source, the high number of complications and the long-term use of immunosuppressive drugs prevent hematopoietic stem cell transplant (HSCT) from being the treatment of choice for MLL-r-related leukemia in children (7).
Most of the recent domestic and international studies (8-11) have focused on the new functions brought by the new fusion protein (FP) part of MLL, however, studies on the N-terminal protein part of MLL shared by MLL FPs are still lacking. Therefore, it is of more general significance to explore the functional studies on MLL-BP (MLL breakpoint), the N-terminal protein part shared by MLL. Due to some recurrent FPs occurred in tumor cells are critical drivers of carcinogenic signals, and which could be effective candidates for targeted therapy. For example, mutations of RAS pathway members are often mentioned in MLL-r leukemia, so we think it is more promising to find out MLL specific signal pathways and use targeted inhibitors (12).
The Janus kinase (JAK) family includes four intracellular non receptor tyrosine kinases, such as JAK1, JAK2, JAK3 and TYK2. After ligand binding, JAK is activated to phosphorylate and activate downstream signal transducers and transcriptional activators (STATs). Therefore, JAKs plays a crucial role in the regulation and homeostasis of hematopoiesis and immunity (13). These results have led to the development of drugs targeting wild-type and/or mutant JAK2. Ruxolitinib, which has been widely used in myeloproliferative tumors, can competes with ATP at the catalytic sites of JAK1 and JAK2 as an oral reversible class I inhibitor (14).
Based on the information above, we implied that JAK/STAT signaling pathway might play an important role in MLL-r leukemia. Hence, we have used a lot of methods to confirm the dependence of MLL on JAK/STAT mediated inflammatory signals in the development of leukemia, and we have identified clinically feasible approaches to treat these leukemias. Of course, this is an early laboratory data showing promise in favour of use of JAK inhibitor in MLL-r ALL, which needs going through multiple more steps before it can be an option in clinical practice. We present this article in accordance with the MDAR reporting checklist (available at https://tp.amegroups.com/article/view/10.21037/tp-23-16/rc).
Methods
Cell culture (15)
Nalm-6 is a human B cell precursor leukemia cell line, purchased from Guangzhou Geneo Biotechnology Co., Ltd. The Nalm-6 cells were cultured in vitro in RPMI 1640 90% with fetal bovine serum (FBS) 10% medium at 95% humidity and 5% CO2 in a constant temperature incubator (37 ℃). Cell growth (density and rate) is measured by passaging at a ratio of 1:3, every 2 to 3 days.
Drug concentration exploration (16)
Human ALL Nalm-6 cells are inoculated into 96-well plates at a density of 2,000 cells per well in a volume of 100 µL per well and treated with exogenously added JAK2/STAT3 signaling pathway inhibitor ruxolitinib for 24 h to a final concentration of 0, 10, 50 and 100 nM, respectively. Then add 10 µL of CCK8 solution to each well and continue to incubate for 1 h. Select the wavelength of 450 nm, measure the light absorption value of each well on an enzyme-linked immunosorbent assay (ELISA), record the results and calculate the cell viability.
Plasmid transfection into Nalm-6 cells (17)
- Pass Nalm-6 cells into a 6-well plate, divided into 4 wells, each well 3. 2×106 cells per well, 2 mL culture medium per well.
- Transfection solution configuration, each well corresponding to take out two 1. Add 200 µL medium to one tube to dilute the plasmid DNA, and mix gently. Add 200 µL of serum-free medium dilution and plasmid transfection reagent to the other tube, mix well, and incubate for 5 min at room temperature. Grouping of plasmids is shown in Table 1.
Table 1
Grouping of plasmidsPlasmids Groups 1 2 3 4 Ruxolitinib − − − + Vector (2 μg) − + − − FLAG-MLL-BP (2 μg) − − + + +, means addition; −, means none. MLL-BP, mixed-lineage leukemia breakpoint. - Add the mixed transfection solution to each well of cells and gently shake well.
- After 24 h, collect the cells.
Fluorescence quantitative polymerase chain reaction (PCR) assay (18)
- Total RNA extraction: Add 1 mL of Trizol reagent to the collected Nalm-6 cells, mix well with gun blast, transfer to RNase-free 1. 5 mL EP tube and lyse for 10 min. Add 1/5 volume of chloroform to the cells, cap the centrifuge tube tightly, shake vigorously by hand for 15 s to form an emulsion, and let stand for 5 min. Note that this step must be a low temperature centrifugation, otherwise the product will have a small amount of genomic contamination. A white precipitate is usually visible. Carefully discard the supernatant and add 1 mL of 75% ethanol prepared with diethyl pyrocarbonate (DEPC) water. Wash the cap and wall of the tube well and flick the bottom of the tube to suspend the precipitate and let it stand for 3–5 min. Centrifuge at 12,000 g for 5 min at 4 ℃ and discard supernatant. Open-dry the precipitate for 2–5 min at room temperature in a clean environment, taking care not to over-dry, as this may result in difficult RNA solubilization. Add the appropriate amount of DEPC water to dissolve the precipitate, if necessary, use a pipette to gently blow a few times, and then take a small amount for detection after complete dissolution, and store the rest at −80 ℃.
- Reverse transcription: use the total RNA extracted from Nalm-6 cells as template to obtain cDNA by reverse transcription.
- Design and synthesize the relevant fluorescent quantitative PCR primers, and the primer sequences are shown in Table 2. Make a 5-fold dilution of the obtained cDNA, add it to the fluorescent quantitative PCR tube according to the dosage in Table 3, and mix well. Place the fluorescent quantitative PCR tube in the fluorescent quantitative PCR instrument, and set up the program as shown in Table 4.
Table 2
Primers for fluorescent quantitative PCRPrimer name Primer sequences (5'-3') MLL-BP-QF CCCATCCCTGGAGAAGGAGA MLL-BP-QR TGGAAGCTTGTCTGCCTGAG JAK2-QF CCAGATGGAAACTGTTCGCTCAG JAK2-QR GAGGTTGGTACATCAGAAACACC STAT3-QF CTTTGAGACCGAGGTGTATCACC STAT3-QR GGTCAGCATGTTGTACCACAGG 18S-QF CCCGCAAATTACCCAATTT 18S-QR GCCCTCCAATTGTTCCTCGTTAAG PCR, polymerase chain reaction; MLL-BP, mixed-lineage leukemia breakpoint; JAK, Janus kinase; STAT, signal transducers and activators of transcription.Table 3
Reaction system for fluorescent quantitative PCRIngredients Dosage 2×ChamQ SYBR qPCR Master Mix 10.0 μL Primer 1 (10 μM) 0.4 μL Primer 2 (10 μM) 0.4 μL 50×ROX Reference Dye1 0.4 μL cDNA 1 μg ddH2O To 20.0 μL PCR, polymerase chain reaction.Table 4
Reaction procedure for fluorescent quantitative PCRStage Procedure Reps Temperature (℃) Time (s) 1 Pre-mutability*1 1 95 30 2 Circular reaction*2 40 95 10 60 30 95 15 3 Dissolution curve*3 1 60 60 95 15 PCR, polymerase chain reaction; Reps, repeat sequence.
Western blot assay (19)
- Cells from each of the 6-well plates are transferred to 2 mL EP tubes, centrifuged at 3,000 rpm for 5 min in a 4 ℃ centrifuge, and the supernatant is discarded. Protease inhibitor phenylmethanesulfonyl fluoride (PMSF) and cocktail are added before use, lyse on ice for 30 min, and resuspend by flicking the bottom of the tube for 10 min each lysis.
- After lysis, centrifuge at 12,000 rpm for 20 min at 4 ℃, transfer the supernatant to a new EP tube, remove 30 µL of supernatant and add 7. The supernatant is transferred to a new EP tube, 30 µL of supernatant is added to 7.5 µL of 5× sodium dodecyl sulfate-polyacrylamide gel electrohoresis (SDS-PAGE) loading buffer, then boiled at 100 ℃ for 10 min, and then centrifuged at 12,000 rpm for 2 min.
- Electrophoresis: fix the prepared gel onto the electrophoresis tank and pour the electrophoresis solution into the reservoir.
- Electrotransfer: the polyvinylidene fluoride (PVDF) membrane is soaked in methanol and then soaked in electrotransfer solution together with the filter paper. Transfer condition: 300 mA current for 30 min.
- Immunoblot color development: after the end of membrane transfer, remove the PVDF membrane and soak it in Tris Buffered Saline with Tween (TBST) skim for closure. Phosphorylated proteins are closed with 1–3% BSA. The primary antibody is diluted with the closure solution, and the dilution is shown in Table 5. After preparing the primary antibody, the PVDF membrane is immersed in the primary antibody dilution and left overnight at 4 ℃ for 4–6 h. TBST is washed fully 5–6 times for 5 min each time. During the washing process, note that the membranes should not adhere to the dish wall nor overlap to each other. After washing, dilute the corresponding secondary antibodies with the blocking solution, immerse the PVDF membrane in the secondary antibody dilution and incubate for 2 h at room temperature in a shaker. Wash again TBST fully 5–6 times for 5 min each time. Configure the enhanced chemiluminescence (ECL) developing solution proportionally, add it dropwise on PVDF film, react for several minutes, blot dry with filter paper, cover with cling film, fix in the cassette, close the cassette and expose according to the actual situation. Remove the film and place it in the developing solution, rinse with water and fix the film. Rinse the film with clean water, dry and scan.
Table 5
Primary antibody dilution ratioName of primary antibody Dilution ratio GAPDH 1:1,000 Flag 1:1,000 p-STAT3 1:1,000 p-JAK2 1:1,000 STAT3 1:1,000 JAK2 1:1,000 GAPDH, glyceraldehyde-3-phosphate dehydrogenase; STAT, signal transducers and activators of transcription; JAK, Janus kinase.
CCK8 assay (20)
The collected cells are inoculated into 96-well plates at a density of 2,000 cells per well in a volume of 100 µL per well, and incubated for 1 h after 1, 2 and 3 d. CCK8 solution is added to each well for 10 µL. The wavelength of 450 nm is selected and the light absorption value of each well was measured on an ELISA, and the results are recorded to calculate the cell viability.
Flow cytometry (FCM)
The collected cells are washed twice with pre-cooled phosphate belanced solution (PBS), each time by centrifugation at 1,000 rpm for 5 min at 4 ℃, and the supernatant was discarded. Refer to Souza et al.’s research for more details (21).
Statistical analysis
Both nominal and numerical data are used χ2 test/Fisher precision test and ANOVA/Student t-test. Kappa coefficients are used to estimate the consistency between qualitative data sets, and Pearson product moment correlation coefficients are used to estimate the linear relationship between quantitative data sets. GraphPad Prism (version 8.2.1 Windows version, GraphPad Software, San Diego) is used for graphical analysis. Unless otherwise stated, all experiments were conducted in triplicate.
Results
Ruxolitinib reduced the viability of B-ALL cell lines
In order to explore the effect for the growth of ALL cells of ruxolitinib, CCK8 assays were used for testing the proliferation of Nalm-6. We dealed with Nalm-6 with different concentrations of ruxolitinib, and then measured the viability by CCK8 assay. As shown in Figure 1A, ruxolitinib could obviously reduce Nalm-6 cell viability in a dose-dependent manner. Culturing the Nalm-6 cell lines in the presence of ruxolitinib showed an IC50 of 47.7 µM. Totally, the findings indicated that ruxolitinib reduced Nalm-6’s viability.
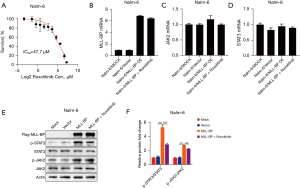
MLL-BP FPs drive potent activation of inflammatory signaling
To biochemically test that STAT3 was activated in MLL-BP ALL, we monitored STAT3 phosphorylation using PCR and Western blotting for key pathway components. An analysis of genes, the mRNA of MLL-BP expression was close between Nalm-6/MLL-BP over-express (OE) cells and Nalm-6 cells treated with ruxolitinib (6.8 vs. 6.4, P>0.05, Figure 1B). When refer to JAK2, which was associated with Nalm-6 expression by PCR assay, revealed that it was not significantly differentially expressed in Nalm-6/MLL-BP OE cells with or without the addition of ruxolitinib (1.1 vs. 0.9, P>0.05, Figure 1C). Also, similar to JAK2, compared with Nalm-6/MLL-BP OE cells, STAT3 was not significantly in Nalm-6 cells treated with ruxolitinib (0.9 vs. 0.8, P>0.05, Figure 1D).
However, by Western blotting assay, we found that among JAK/STAT signal way, STAT3 was a critical downstream mediator. This reduction in STAT3 phosphorylation, which occurred after Nalm-6/MLL-BP OE plus ruxolitinib, suggests that STAT3 activation is a direct consequence of Nalm-6/MLL-BP OE protein expression in ALL cells (p-STAT3/STAT3: 5.3 vs. 2.8, P<0.05, Figure 1E,1F). Furthermore, noticeable decrease in phospho-JAK2 were also observed in the Nalm-6/MLL-BP OE fusion compared to Nalm-6/MLL-BP OE plus ruxolitinib (p-JAK2/JAK2: 2.7 vs. 2.2, P<0.05, Figure 1E,1F). Taken together, these studies indicate that Nalm-6/MLL-BP OE protein but not MLL-BP gene activate the transcriptional circuitry of inflammatory signaling networks.
Ruxolitinib suppressed the proliferation of Nalm-6 cells by arresting cell cycle at G0/G1 phase
When we used CCK8 to detect cell proliferation, we found that when compared with the Nalm-6/MLL-BP OE transfected Nalm-6 cell group, the survival of Nalm-6 cell was significantly decreased from 113.6%±2.68% to 31.45%±2.68% when cells were treated with ruxolitinib (P<0.001) (Figure 2A). To confirm if the viability decrease among Nalm-6 treated by ruxolitinib was because the decreased proliferation in cell, subsequently we study the effects of ruxolitinib on cell cycle distribution in Nalm-6. As shown in Figure 2B,2C, compared with the Nalm-6/MLL-BP OE transfected Nalm-6 cell group, the ratio of Nalm-6 in the G0/G1 phase was significantly increased from 46.71%±1.95% to 63.85%±1.95% when cells were treated with ruxolitinib (P<0.001). These results suggest that ruxolitinib suppressed the proliferation of Nalm-6 cells by arresting cell cycle at G0/G1 phase.
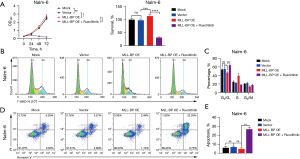
Ruxolitinib promoted the apoptosis of Nalm-6 cells
Whether ruxolitinib have effect on the cell survival of Nalm-6, we conducted the FCM assays. Nalm-6 cells were treated with ruxolitinib for 24 h, and then cells were stained with Annexin-V and propidium iodide (PI) and analyzed by FCM assays. As shown in Figure 2D,2E, when compared to the Nalm-6/MLL-BP OE transfected Nalm-6 cell group, the proportion of Nalm-6 cell was significantly increased from 4.8% to 26.8% when cells were treated with ruxolitinib (P<0.001). In total, these results indicated that ruxolitinib promoted the apoptosis of Nalm-6/MLL-BP OE transfected Nalm-6 cells.
Discussion
MLL-r is typically associated with activation of overlapping molecular pathways among ALL with poor outcomes (22-25). Generally, infants ALL with MLL-r have a 5-year EFS of 20–40%, while those with wild-type MLL have a percent of 60% or higher (26,27). Childhood ALL patients with MLL-r over than 1 year are better than infants, but remained not as good as non-MLL-r cases (28). The latest data estimates that the 5-year EFS for children with ALL is about 60%, while overall it is about 92% (29). Therefore, these patients are considered to be at high risk for pediatric ALL, further reinforcing the urgent and unmet need to identify effective and highly targeted therapies for this malignant tumor in childhood population.
More and more data indicate that proteasome inhibitors may be a promising drug for supplementing MLL-r leukemia treatment. Kamens et al. noted that the expression level of MLL FP in leukemia cells is not too high, and hypothesized that strict regulation of FP expression may be achieved through proteasome mechanisms. In fact, they demonstrated that proteasome inhibitor treatment increased the protein level of wild-type MLL and, to a greater extent, increased the protein level of the MLL FP (30). Although other targeted therapies, such as hypomethylating agents, histone deacetylase (HDAC) inhibitors and FMS-like tyrosine kinase-3 (FLT-3) inhibitors, are being explored as treatment for MLL-r ALL, However, the translation potential of these targets remains to be determined by clinical research (31-33). Patients with MLL-r continue to receive extreme cytotoxic therapy associated with induction failure and severe debilitating effects, even if lasting therapeutic effects are achieved.
Although there is a strong theoretical basis for targeting tumor promoting fusion oncoproteins in cancer, most of these oncoproteins are still difficult to target as drugs. One way to circumvent this issue is to identify downstream transcriptional or signaling networks activated by these FPs and target them. It is worth noting that this study in our cell line has shown that ALL cells carrying MLL-BP fusion products are highly sensitive to ruxolitinib, indicating that these fusion products are attractive candidates for targeted treatment of MLL leukemia. In addition to these studies, our extensive characterization of the JAK/STAT signaling pathway helps to expand the molecular network of MLL-r leukemia, which will help to understand the hitherto unknown mechanism of MLL-r leukemia. More importantly, these datasets on MLL-r leukemia will help identify and prioritize targeted therapeutic candidates for the disease. We found that MLL-BP FP directly recruits JAK2 and effectively activates inflammatory signaling, which provides an attractive pathway for therapeutic intervention.
Recently, there have been some reports about the use of ruxolitinib in children with acute lymphocytic leukemia. It is well known that Philadelphia chromosome like acute lymphoblastic leukemia (Ph-like ALL) is a high-risk subtype of ALL with a high recurrence rate and poor prognosis. Functional acquisition mutations in JAK2 have been found in high-risk Ph-like ALL subtypes, occurring only in conjunction with rearrangement of CRLF2 (CRLF2r), which leads to overexpression of CRLF2. Approximately 50% of Ph-like ALL patients harbor CRLF2r, and roughly half of these patients also harbor activating point mutations in JAK1 or JAK2 (34). A previous study conducted preclinical in vivo drug testing and L-asparaginase in 2/3 CRLF2 rearranged Ph-like ALL xenografts, which supported evaluation of the addition of ruxolitinib to a conventional induction regimen for the treatment of CRLF2 recombinant Ph-like ALL (35). Lately, another clinical study showed the outcome concerning administration of ruxolitinib in twelve Ph-like ALL pediatric patients. Finally, treatment with ruxolitinib resulted in complete (n=7) and partial (n=2) remission in three patients, but no information was found, so they concluded that ruxolitinib could be used as an additional compound to activate the JAK-STAT pathway in Ph-like ALL patients (36). Consistent with above study, our study also demonstrated that aberrations activating JAK-STAT pathway existed in MLL-r leukemia, and the effect could be reversed by ruxolitinib. Our findings indicate that there are specific requirements for JAK2 in high-risk ALL, indicating that in the clinical context of the need for new treatment methods, such methods may have important therapeutic indicators.
Interestingly, the anti-leukemia effects of ruxolitinib were observed in MLL-r ALL cells line in our study for the first time, our study showed that ruxolitinib inhibited JAK/STAT activation and significantly impaired the proliferation of MLL-BP FP ALL cells and blocked the cell cycle in G0/G1 phase, leading to an increase in apoptosis. We acknowledge that our study also has limitations. Firstly, we only explored this effect in vitro, thus, the next step for us is to study animal models in vivo, in order to further confirm the conclusion. Secondly, we explored the common part of MLL, but it remains unclear which partner gene of MLL-r leukemia patients might benefit most from these therapies.
Conclusions
Our studies demonstrated that ruxolitinib inhibits MLL-r proliferation and induces apoptosis by the JAK/STAT pathway, revealing the effects of ruxolitinib therapy on MLL-r leukemia. This is an early laboratory data showing promise in favour of use of JAK inhibitor in MLL-r ALL. However, it needs going through multiple more steps to confirm before it can be an option in clinical practice.
Acknowledgments
Funding: This work was supported by the Guangzhou Science and Technology Program key projects (No. 201803010032), Beijing New Sunshine Charity Foundation, Sun Yat-sen Scientific Research Project (No. SYSKY-2022-103-01), Bethune Medical Scientific Research Fund Project (No. SCE111DS), Guangdong Basic and Applied Basic Research Fund (No. 2021A1515011809), Basic Research Project (Dengfeng Hospital) jointly funded by Universities (Institutes) in Guangzhou (No. 202201020310) and Sun Yat-sen Scientific Research Sailing Project (No. YXQH202205).
Footnote
Reporting Checklist: The authors have completed the MDAR reporting checklist. Available at https://tp.amegroups.com/article/view/10.21037/tp-23-16/rc
Data Sharing Statement: Available at https://tp.amegroups.com/article/view/10.21037/tp-23-16/dss
Peer Review File: Available at https://tp.amegroups.com/article/view/10.21037/tp-23-16/prf
Conflicts of Interest: All authors have completed the ICMJE uniform disclosure form (available at https://tp.amegroups.com/article/view/10.21037/tp-23-16/coif). The authors have no conflicts of interest to declare.
Ethical Statement: The authors are accountable for all aspects of the work in ensuring that questions related to the accuracy or integrity of any part of the work are appropriately investigated and resolved.
Open Access Statement: This is an Open Access article distributed in accordance with the Creative Commons Attribution-NonCommercial-NoDerivs 4.0 International License (CC BY-NC-ND 4.0), which permits the non-commercial replication and distribution of the article with the strict proviso that no changes or edits are made and the original work is properly cited (including links to both the formal publication through the relevant DOI and the license). See: https://creativecommons.org/licenses/by-nc-nd/4.0/.
References
- Valentine MC, Linabery AM, Chasnoff S, et al. Excess congenital non-synonymous variation in leukemia-associated genes in MLL- infant leukemia: a Children's Oncology Group report. Leukemia 2014;28:1235-41. [Crossref] [PubMed]
- Meyer C, Hofmann J, Burmeister T, et al. The MLL recombinome of acute leukemias in 2013. Leukemia 2013;27:2165-76. [Crossref] [PubMed]
- Qiu KY, Zhou DH, Liao XY, et al. Prognostic value and outcome for acute lymphocytic leukemia in children with MLL rearrangement: a case-control study. BMC Cancer 2022;22:1257. [Crossref] [PubMed]
- Guo C, Zhang Y, Shuai S, et al. The super elongation complex (SEC) mediates phase transition of SPT5 during transcriptional pause release. EMBO Rep 2023;24:e55699. [Crossref] [PubMed]
- Sun YN, Hu YX, Gao L, et al. The therapeutic efficacy of pediatric ALL patients with MLL gene rearrangement treated with CCLG-ALL2008 protocol. Eur Rev Med Pharmacol Sci 2018;22:6020-9. [PubMed]
- Qiu KY, Xu HG, Luo XQ, et al. Prognostic Value and Outcome for ETV6/RUNX1-Positive Pediatric Acute Lymphoblastic Leukemia: A Report From the South China Children's Leukemia Group. Front Oncol 2021;11:797194. [Crossref] [PubMed]
- Dreyer ZE, Dinndorf PA, Camitta B, et al. Analysis of the role of hematopoietic stem-cell transplantation in infants with acute lymphoblastic leukemia in first remission and MLL gene rearrangements: a report from the Children's Oncology Group. J Clin Oncol 2011;29:214-22. [Crossref] [PubMed]
- Qiu KY, Liao XY, Liu Y, et al. Poor outcome of pediatric patients with acute myeloid leukemia harboring high FLT3/ITD allelic ratios. Nat Commun 2022;13:3679. [Crossref] [PubMed]
- Qiu KY, Wang JY, Huang LB, et al. Vincristine and dexamethasone pulses in addition to maintenance therapy among pediatric acute lymphoblastic leukemia (GD-ALL-2008): An open-label, multicentre, randomized, phase III clinical trial. Am J Hematol 2023;98:869-80. [Crossref] [PubMed]
- Lee HG, Baek HJ, Kim HS, et al. Biphenotypic acute leukemia or acute leukemia of ambiguous lineage in childhood: clinical characteristics and outcome. Blood Res 2019;54:63-73. [Crossref] [PubMed]
- Cui L, Li ZG, Chai YH, et al. Outcome of children with newly diagnosed acute lymphoblastic leukemia treated with CCLG-ALL 2008: The first nation-wide prospective multicenter study in China. Am J Hematol 2018;93:913-20. [Crossref] [PubMed]
- Winters AC, Bernt KM. MLL-Rearranged Leukemias-An Update on Science and Clinical Approaches. Front Pediatr 2017;5:4. [Crossref] [PubMed]
- Becker H, Engelhardt M, von Bubnoff N, et al. Ruxolitinib. Recent Results Cancer Res 2014;201:249-57. [Crossref] [PubMed]
- Ajayi S, Becker H, Reinhardt H, et al. Ruxolitinib. Recent Results Cancer Res 2018;212:119-32. [Crossref] [PubMed]
- Li Y, Zuo C, Gu L. Characterization of a novel glucocorticoid-resistant human B-cell acute lymphoblastic leukemia cell line, with AMPK, mTOR and fatty acid synthesis pathway inhibition. Cancer Cell Int 2021;21:623. [Crossref] [PubMed]
- Chimalakonda A, Burke J, Cheng L, et al. Selectivity Profile of the Tyrosine Kinase 2 Inhibitor Deucravacitinib Compared with Janus Kinase 1/2/3 Inhibitors. Dermatol Ther (Heidelb) 2021;11:1763-76. [Crossref] [PubMed]
- Kiani M, Salehi M, Mogheiseh A, et al. The Effect of Increased miR-16-1 Levels in Mouse Embryos on Epigenetic Modification, Target Gene Expression, and Developmental Processes. Reprod Sci 2020;27:2197-210. [Crossref] [PubMed]
- Guo P, Wang P, Liu L, et al. Naringin Alleviates Glucose-Induced Aging by Reducing Fat Accumulation and Promoting Autophagy in Caenorhabditis elegans. Nutrients 2023;15:907. [Crossref] [PubMed]
- Pillai-Kastoori L, Schutz-Geschwender AR, Harford JA. A systematic approach to quantitative Western blot analysis. Anal Biochem 2020;593:113608. [Crossref] [PubMed]
- Zhou J, Jiang YY, Chen H, et al. Tanshinone I attenuates the malignant biological properties of ovarian cancer by inducing apoptosis and autophagy via the inactivation of PI3K/AKT/mTOR pathway. Cell Prolif 2020;53:e12739. [Crossref] [PubMed]
- Souza IMFNB, Siqueira VDS, Ribeiro IDC, et al. Molecular and serological diagnosis of toxoplasmosis: a systematic review and meta-analysis. Rev Inst Med Trop Sao Paulo 2023;65:e19. [Crossref] [PubMed]
- Wyss P, Song C, Bina M. Along the Bos taurus genome, uncover candidate imprinting control regions. BMC Genomics 2022;23:478. [Crossref] [PubMed]
- Li Y, Han J, Zhang Y, et al. Structural basis for activity regulation of MLL family methyltransferases. Nature 2016;530:447-52. [Crossref] [PubMed]
- Kalmode HP, Podsiadly I, Kabra A, et al. Small-Molecule Inhibitors of the MLL1 CXXC Domain, an Epigenetic Reader of DNA Methylation. ACS Med Chem Lett 2022;13:1363-9. [Crossref] [PubMed]
- Grey W, Atkinson S, Rix B, et al. The CKS1/CKS2 Proteostasis Axis Is Crucial to Maintain Hematopoietic Stem Cell Function. Hemasphere 2023;7:e853. [Crossref] [PubMed]
- Tomizawa D, Koh K, Sato T, et al. Outcome of risk-based therapy for infant acute lymphoblastic leukemia with or without an MLL gene rearrangement, with emphasis on late effects: a final report of two consecutive studies, MLL96 and MLL98, of the Japan Infant Leukemia Study Group. Leukemia 2007;21:2258-63. [Crossref] [PubMed]
- Kotecha RS. Updates in infant acute lymphoblastic leukemia and the potential for targeted therapy. Hematology Am Soc Hematol Educ Program 2022;2022:611-7. [Crossref] [PubMed]
- Hunger SP, Mullighan CG. Acute Lymphoblastic Leukemia in Children. N Engl J Med 2015;373:1541-52. [Crossref] [PubMed]
- Attarbaschi A, Möricke A, Harrison CJ, et al. Outcomes of Childhood Noninfant Acute Lymphoblastic Leukemia With 11q23/KMT2A Rearrangements in a Modern Therapy Era: A Retrospective International Study. J Clin Oncol 2023;41:1404-22. [Crossref] [PubMed]
- Kamens JL, Nance S, Koss C, et al. Proteasome inhibition targets the KMT2A transcriptional complex in acute lymphoblastic leukemia. Nat Commun 2023;14:809. [Crossref] [PubMed]
- Xue K, Song J, Yang Y, et al. PAX5 promotes pre-B cell proliferation by regulating the expression of pre-B cell receptor and its downstream signaling. Mol Immunol 2016;73:1-9. [Crossref] [PubMed]
- Cruickshank MN, Ford J, Cheung LC, et al. Systematic chemical and molecular profiling of MLL-rearranged infant acute lymphoblastic leukemia reveals efficacy of romidepsin. Leukemia 2017;31:40-50. [Crossref] [PubMed]
- Stumpel DJ, Schneider P, van Roon EH, et al. Specific promoter methylation identifies different subgroups of MLL-rearranged infant acute lymphoblastic leukemia, influences clinical outcome, and provides therapeutic options. Blood 2009;114:5490-8. [Crossref] [PubMed]
- Downes CE, McClure BJ, McDougal DP, et al. JAK2 Alterations in Acute Lymphoblastic Leukemia: Molecular Insights for Superior Precision Medicine Strategies. Front Cell Dev Biol 2022;10:942053. [Crossref] [PubMed]
- Bӧhm JW, Sia KCS, Jones C, et al. Combination efficacy of ruxolitinib with standard-of-care drugs in CRLF2-rearranged Ph-like acute lymphoblastic leukemia. Leukemia 2021;35:3101-12. [Crossref] [PubMed]
- Kołodrubiec J, Kozłowska M, Irga-Jaworska N, et al. Efficacy of ruxolitinib in acute lymphoblastic leukemia: A systematic review. Leuk Res 2022;121:106925. [Crossref] [PubMed]