Development and validation of a predictive model for low cardiac output syndrome after surgical repair of tetralogy of Fallot
Highlight box
Key findings
• SpO2, MBP, and CVP are independent risk factors for LCOS after surgical repair of TOF.
What is known and what is new?
• Multiple predictive models have already been developed to predict outcomes in TOF. However, none serves to predict LCOS after TOF repair.
• This study incorporated pre- and intraoperative characteristics to develop a predictive model for LCOS after surgical repair of TOF in children.
What is the implication, and what should change now?
• Nomograms are developed to calculate the risk of individual patients and contribute to diagnosis and treatment.
Introduction
Tetralogy of Fallot (TOF) is the most common cyanotic congenital heart disease (CHD). Outcomes of surgical repair of TOF have significantly improved over time, but low cardiac output syndrome (LCOS) remains a serious postoperative complication for children with TOF, which often leads to increased morbidity and mortality (1). The incidence of LCOS after surgical repair of TOF in the literature ranges from 8.16–34.8% (2,3). The occurrence of LCOS after surgery is influenced by many factors, such as preload, afterload, myocardial contractility, heart rate, and rhythm. While the most likely cause of LCOS after TOF repair is ventricular diastolic dysfunction and failure (4). Imaging examinations such as cardiovascular magnetic resonance can be used to assess the diastolic function after operation for patients with TOF (5). Early identification of LCOS and timely management are critical for better outcomes.
The risk factors for postoperative LCOS after CHD surgery have been identified previously. A meta-analysis which included 18 studies showed that age, type of CHD, cardiac reoperation, biventricular shunt before operation, cardiopulmonary bypass (CPB) time, aortic cross-clamp (ACC) time, postoperative residual shunt, cTn-1 level 2 h after CPB >14 ng/mL and postoperative 24 h MR-ProADM level >1.5 nmol/L were independent risk factors of LCOS after CHD surgery (6). However, these risk factors for LCOS in CHD patients may not be relevant to patients with TOF undergoing surgical repair, given the heterogeneities of CHD. Identifying the risk factors for impaired postoperative myocardial performance may help to improve risk stratification and perioperative management for the treatment of TOF. To date, few studies have identified the predictors of LCOS after surgical repair of TOF. Richardson et al. reported that previous palliative shunt procedure, chronic hypoxia, age <5 years, severe postoperative right ventricular hypertension, and extensive right ventricular outflow tract reconstruction were risk factor for LCOS after surgical repair of TOF (7).
In recent years, statistical predictive models like the nomogram have been developed to generate a simple graphical representation for individualized prognostic predictions. Nomograms are commonly used to predict the prognosis with high accuracy compared to prognostic grouping or scoring systems (8). Multiple predictive models have already been developed to predict outcomes in TOF. Liu et al. (9) developed and validated a risk-classifier-oriented nomogram for in-hospital complications in children with TOF repaired at an older age. This nomogram showed good discrimination and good fit both in the training and external validation datasets. Liu et al. (10,11) identified and integrated the risk covariates to build and validate prediction nomograms for cardiovascular disorder and acute lung injury after surgical repair of TOF. They found that the nomograms integrating perfusate oxygenation slightly improved the risk discrimination compared with covariate-based nomogram alone both in the training and validation datasets. So far, no predictive models have been developed to predict LCOS after TOF repair.
The purpose of this study was to identify the predictors of LCOS after surgical repair of TOF and to develop a prediction model incorporating pre- and intraoperative characteristics for LCOS within 24 hours after surgical repair of TOF in children. Based on their availability and clinical adaptability, preoperative and intraoperative risk factors in the literature were selected for model development which might contribute to the accuracy of the current model for predicting LCOS. We present this article in accordance with the TRIPOD reporting checklist (available at https://tp.amegroups.com/article/view/10.21037/tp-22-498/rc).
Methods
Study design and population
In this retrospective cohort study, patients with TOF who underwent surgical repair under cardiopulmonary bypass at Shanghai Children’s Medical Center (SCMC) from 2021 to 2022 were identified from the hospital database. Patients who underwent palliative surgery were excluded from this study. The training dataset consisted of patients in 2021, while the validation dataset consisted of patients in 2022. The study was conducted in accordance with the Declaration of Helsinki (as revised in 2013). The study was approved by the Institutional Review Board of Shanghai Children’s Medical Center (SCMC) (No. SCMCIRB-K2022071-1). Written informed consent was obtained from all the parents of the patients.
Data collection
Preoperative, intraoperative, and postoperative data were obtained from medical records. The literature investigating factors associated with LCOS was examined. Based on their availability and clinical adaptability, 8 preoperative and intraoperative factors were selected for model development. Preoperative data included age, gender, weight, and peripheral oxygen saturation (SpO2). Intraoperative data included cardiopulmonary bypass (CPB) time, aortic cross-clamp (ACC) time, mean blood pressure (MBP) and central venous pressure (CVP) recorded at the conclusion of the cardiopulmonary bypass. Postoperative data included the duration of ventilation support, cardiac intensive care unit (CICU) and hospital stay, in-hospital mortality and complications (e.g., LCOS, ventricular fibrillation).
Study outcomes
Our primary outcome was LCOS within 24 hours after surgical repair of TOF. Since invasive measurement of cardiac index is not routine, LCOS is mainly defined on the basis of a combination of clinical signs and abnormal parameters (12): (I) cardiac index <2.2 L·min-1·m-2, (II) vasoactive inotropic score >20 (13), (III) lactic acid >3 mmol/L (27 mg/dL) or at least 2 mmol/L (18 mg/dL) higher than the baseline, (IV) urine output <1 mL·kg-1·h-1, (V) core body-peripheral skin temperature difference >7 degrees, (VI) central venous oxygen saturation <50% or arterial-central venous oxygen saturation difference >40% (14,15), (VII) systolic blood pressure <5th percentile of age by sex (16), (VII) serious adverse events such as extracorporeal membrane oxygenation (ECMO) or ventricular assist device support, cardiac arrest or death. Patients were considered meeting the primary outcome if two or more of the aforementioned criteria were shown.
Statistical analysis
Continuous variables were presented as mean ± standard deviation or median with interquartile range (IQR) and the difference was analyzed by student’s t-test or Mann-Whitney U test, as appropriate. Categorical variables were presented as absolute number or percentage and the difference was analyzed by Pearson χ2 test or Fisher’s exact test. Univariable and multivariable logistic regression models were used to assess factors associated with LCOS. Variables with P<0.1 in the univariable model were entered into the multivariable model. For binary outcome measures, we hypothesized a minimum of 5 events per variable is required in order to prevent overfitting (17). In the training dataset, the effective sample size was attained (33 events for 5 variables in the multivariable logistic regression model and 3 variables in the nomogram. A nomogram was generated based on independent risk factors in the multivariable logistic regression model. A receiver operating characteristic (ROC) curve was constructed to determine the area under the ROC curve (AUC) with 95% confidence interval. A threshold value of AUC >0.7 indicated good performance of the prediction model. The optimal cut-off value was calculated to maximize the Youden index. The sensitivity and specificity were reported at the optimal cut-off value. The calibration of the nomogram was evaluated by the calibration curve and the Hosmer-Lemeshow test was used to assess the good fit. Decision curve analysis (DCA) was used to evaluate the net benefits of the nomogram at different threshold probabilities. R version 4.2.1 (R Foundation for Statistical Computing) was used for statistical analyses. A two-sided P<0.05 was considered statistically significant.
Results
Patient characteristics
The training dataset included 137 patients (85 male), among whom 33 (24.08%) had postoperative LCOS. While the validation dataset included 101 patients, among whom 25 (24.75%) had postoperative LCOS. The overall median age and weight were 194 (IQR, 149–345) days and 7.5 (IQR, 6.3–8.6) kg, respectively, in the training dataset. While the overall median age and weight were 175 (IQR, 132–234) days and 7.3 (6.5–8.40) kg, respectively, in the validation dataset. The median SpO2 were 95% (IQR, 85–98%) and 90% (IQR, 89–98%), respectively, in the training and validation datasets. The preoperative characteristics of patients who did and did not develop postoperative LCOS are presented in Table 1. There was a significant difference in SpO2 between the 2 groups (P=0.002 and 0.003, respectively) both in the training and validation datasets. There was no significant difference in gender (P=0.688 and 0.526, respectively), age (P=0.819 and 0.281, respectively), and weight (P=0.639 and 0.132, respectively) between the 2 groups both in the training and validation datasets.
Table 1
Variable | Training dataset | Validation dataset | |||||
---|---|---|---|---|---|---|---|
LCOS (n=33) | Non-LCOS (n=104) | P value | LCOS (n=25) | Non-LCOS (n=76) | P value | ||
Age, day | 193 (132, 406) | 196 (149.75, 302.75) | 0.819 | 155 (128, 216.00) | 182 (135.25, 240.00) | 0.281 | |
Weight, kg | 7.90 (6.40, 9.00) | 7.50 (6.30, 8.50) | 0.639 | 7.05±1.26 | 7.60±1.65 | 0.132 | |
Male | 19 (57.6) | 66 (63.5) | 0.688 | 13 (52.0) | 47 (61.8) | 0.526 | |
SpO2, % | 85 (80, 95) | 95 (90, 98) | 0.002 | 90 (84, 91) | 93 (90, 98) | 0.003 | |
MBP, mmHg | 61.64±8.58 | 68.13±8.71 | <0.001 | 63.42±7.94 | 65.77±8.57 | 0.229 | |
CVP, mmHg | 13.67 (13.00, 14.67) | 12.33 (11.00, 13.33) | <0.001 | 14.75±1.82 | 12.82±1.54 | <0.001 | |
CPB time, min | 106.00 (85.00, 140.00) | 88.00 (66.50, 105.25) | 0.002 | 97.80±25.11 | 84.00 (72.75, 98.00) | 0.046 | |
ACC time, min | 64.00 (50.00, 81.00) | 51.50 (41.00, 66.25) | 0.003 | 59.88±14.96 | 51.00 (41.75, 61.00) | 0.051 | |
CICU stay, day | 6 (4, 7) | 3 (2, 4) | <0.001 | 5.08±1.53 | 4.00 (3.00, 5.00) | 0.001 | |
Ventilation, hour | 72.03 (49.28, 121.85) | 26.16 (21.31, 48.26) | <0.001 | 48.93 (25.03, 68.57) | 42.59 (23.66, 46.94) | 0.025 | |
Hospital stay, day | 19.00 (16.00, 22.00) | 13.00 (11.00, 16.00) | <0.001 | 17.04±3.75 | 15.00 (13.00, 17.00) | 0.055 | |
Delayed chest closure | 6 (18.2) | 1 (1) | 0.001 | 4 (16.0) | 0 (0) | 0.003 | |
ECMO | 3 (9.1) | 1 (1) | 0.043 | 3 (12.0) | 0 (0) | 0.017 | |
Ventricular fibrillation | 4 (12.1) | 0 (0) | <0.001 | 3 (12.0) | 1 (1.3) | 0.074 | |
Reoperation | 3 (9.10) | 2 (1.92) | 0.090 | 1 (4.0) | 0 (0) | 0.557 | |
Peritoneal dialysis | 10 (30.30) | 1 (0.96) | <0.001 | 8 (32.0) | 0 (0) | <0.001 | |
Death | 1 (3) | 1 (1) | 0.425 | 1 (4.0) | 0 (0) | 0.557 |
Continuous variables are presented as mean ± standard deviation or median (interquartile range) according to data distribution. Categorical variables are presented as number (percentage). ACC, aortic cross-clamp; CICU, cardiac intensive care unit; CPB, cardiopulmonary bypass; CVP, central venous pressure; ECMO, extracorporeal membrane oxygenation; LCOS, low cardiac output syndrome; MBP, mean blood pressure.
Intraoperative data
Intraoperative data are presented in Table 1. The overall median CPB and ACC time were 89 (IQR, 71–113) and 54 (IQR, 44–73) minutes, respectively, in the training dataset. While the overall median CPB and ACC time were 86 (IQR, 73–102) and 52 (IQR, 43–65.5) minutes, respectively, in the validation dataset. The overall mean MBP was 66.57±9.09 mmHg and the overall median CVP was 13 (IQR, 11.33–13.67) mmHg, respectively, in the training dataset. While the overall mean MBP was 65.19±8.44 mmHg and the overall median CVP was 13.33 (IQR, 12–14.42) mmHg, respectively, in the validation dataset. Patients who developed postoperative LCOS had longer CPB (P=0.002) and ACC time (P=0.003), and lower MBP (P<0.001) and higher CVP (P<0.001) in the training dataset. While patients who developed postoperative LCOS had longer CPB (P=0.046) and higher CVP (P<0.001) in the validation dataset.
Postoperative results
Postoperative results are presented in Table 1. The overall in-hospital mortality rate was 1.5% (n=2) and 1.0% (n=1), respectively, in the training and validation datasets. The overall median CICU stay was 4 (IQR, 3–5) days in both the training and validation datasets. The overall hospital stay was 14 (IQR, 12–18) and 16 (IQR, 13–18) days, respectively, in the training and validation datasets. The overall median length of ventilator support was 42.77 (IQR, 22.33–65.80) and 43.78 (24.07, 50.00) hours, respectively, in the training and validation datasets. Delayed sternal closure, ECMO, and peritoneal dialysis were performed in 7 patients (5.11%), 4 patients (2.92%) and 11 patients (8.03%), respectively, in the training dataset while 4 patients (3.96%), 3 patients (2.97%) and 8 patients (7.92%), respectively, in the validation dataset. Ventricular fibrillation developed in 4 patients (2.92% and 3.96%, respectively) both in the training and validation datasets. Patients who developed LCOS required longer mechanical ventilation support (P<0.001), postoperative cardiac intensive care unit stay (P<0.001), and hospital stay (P<0.001) in the training dataset. The incidence of delayed chest closure (P=0.001), ECMO support (P=0.043), ventricular fibrillation (P<0.001), and peritoneal dialysis (P<0.001) were significantly higher in patients who developed LCOS in the training dataset. There was no significant difference in the incidence of reoperation (P=0.090) between the 2 groups, nor a significant difference in in-hospital mortality (P=0.425) in the training dataset. Patients who developed LCOS required longer mechanical ventilation support (P<0.025) and postoperative cardiac intensive care unit stay (P=0.001) in the validation dataset. The incidence of delayed chest closure (P=0.003), ECMO support (P=0.017), and peritoneal dialysis (P<0.001) were significantly higher in patients who developed LCOS in the validation dataset. There was no significant difference in the length of hospital stay (P=0.055), in the incidence of ventricular fibrillation (P=0.074), reoperation (P=0.557), or in-hospital mortality (P=0.557) between the 2 groups in the validation dataset.
Logistic regression analysis
Results of univariable and multivariable logistic analyses for risk factors for postoperative LCOS in the training dataset are provided in Table 2. In the univariable analysis, SpO2 (P=0.001), CPB time (P<0.001), ACC time (P=0.006), MBP (P=0.001) and CVP (P<0.001) were risk factors for postoperative LCOS. In the multivariable analysis, SpO2 (P=0.016), MBP (P=0.004), and CVP (P=0.002) were independent risk factors for postoperative LCOS.
Table 2
Variable | Univariable analysis | Multivariable analysis | |||||
---|---|---|---|---|---|---|---|
OR | 95% CI | P value | OR | 95% CI | P value | ||
Gender | 1.280 | 0.577–2.841 | 0.544 | ||||
Age | 1.000 | 1.000–1.001 | 0.227 | ||||
Weight | 1.015 | 0.955–1.080 | 0.624 | ||||
SpO2 | 0.935 | 0.897–0.974 | 0.001 | 0.942 | 0.898–0.989 | 0.016 | |
MBP | 0.913 | 0.866–0.962 | 0.001 | 0.905 | 0.846–0.968 | 0.004 | |
CVP | 2.201 | 1.511–3.208 | <0.001 | 1.867 | 1. 257–2.775 | 0.002 | |
CPB time | 1.018 | 1.008–1.028 | <0.001 | 1.014 | 0.993–1.035 | 0.186 | |
ACC time | 1.025 | 1.007–1.043 | 0.006 | 1.001 | 0.965–1.039 | 0.956 |
ACC, aortic cross-clamp; CI, confidence interval; CPB, cardiopulmonary bypass; CVP, central venous pressure; MBP, mean blood pressure; OR, odds ratio.
Nomogram construction and model evaluation
Based on the multivariable logistic regression model in the training dataset, an intuitive nomogram integrating SpO2, MBP, and CVP for predicting postoperative LCOS after surgical repair of TOF is constructed and presented in Figure 1. The receiver operating characteristic (ROC) curve was established to evaluate the predictive value of the model. The area under ROC curve was 0.84 (95% CI: 0.77–0.91) and 0.80 (95% CI: 0.70–0.90) in the training and validation datasets, respectively, which meant the prediction ability of the model is stable (Figure 2). At the optimal cut-off value in the training dataset, the sensitivity and specificity were 87.9% and 68.3%, respectively. While at the optimal cut-off value in the validation dataset, the sensitivity and specificity were 68.0% and 78.9%, respectively. The calibration curve for the probability of LCOS showed good agreement between the prediction by nomogram and actual observation both in the training and validation datasets (Figure 3). The Hosmer-Lemeshow test yielded nonsignificant statistics both in the training and validation datasets (P=0.69 and 0.54, respectively), indicating a good fit. The DCA revealed that more net benefits would be obtained by using the nomogram to predict LCOS than that achieved in either the treat-all-patient scheme or the treat-none scheme both in the training and validation datasets (Figure 4).
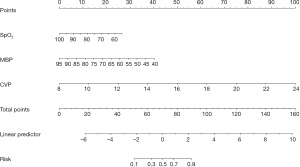
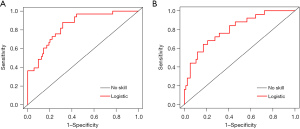
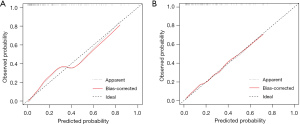
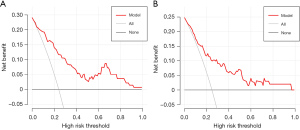
Discussion
This study found that SpO2, MBP and CVP were independent risk factors for postoperative LCOS after surgical repair of TOF. A predictive nomogram based on these pre- and intraoperative characteristics was developed to predict LCOS after surgical repair of TOF in children. This model showed good discrimination, good fit and clinical benefits.
The variables included in the predictive model are all readily available. SpO2 is a routine test item during hospitalization. CVP and MBP are routinely monitored during heart surgery. This nomogram is easy to implement in clinical practice.
Although TOF has traditionally been considered as a right heart defect, it is increasingly accepted that biventricular dysfunction begins early after surgical repair in patients with TOF (18,19). The surgical destruction of pulmonary valve integrity leads to pulmonary regurgitation, right ventricular volume overload, poor right ventricular remodeling, and therefore affecting the diastolic function of both left and right ventricles due to adverse ventricular interaction. Prolonged and uneven postoperative recovery in these patients is characterized in our study. The effects of biventricular circulation are comprehensively considered in this prediction model. MBP can represent left ventricular function and systemic resistance, while CVP reflects right ventricular function and volume status.
This study demonstrated that lower SpO2 was an independent risk factor for postoperative LCOS. Many studies also revealed that preoperative hypoxia would induce structural changes in the myocardium and impair cardiac function in TOF. Preoperative oxygen saturation ≤75% is independent risk factor for LCOS and prolonged intubation period (20-22). The cause of the association is multifactorial. First, hypoxia can initiate cardiac injury process where cardiac fibroblasts convert to myofibroblasts. Myofibroblasts produce a large amount of extracellular matrix which leads to pathologic remodeling, myocardial fibrosis and eventually ventricular dysfunction (23). Second, metabolic reprogramming changes in response to chronic hypoxia may lead to chronic myocardial damage (24). In addition, hypoxia would increase sensitivity to free radical injury of myocytes and trigger systemic inflammatory response, therefore leading to reperfusion injury after cardiac surgery (25).
The data from this study demonstrated that elevated CVP was an independent predictor for postoperative LCOS. For patients with TOF, higher CVP indicates the presence of right ventricular restrictive physiology which would lead to LCOS in the early postoperative period after surgical repair (26). The right ventricle fails to dilate effectively and serves merely as a passive conduit for antegrade diastolic pulmonary blood flow during atrial systole. The retrograde flow in the superior vena cava and rapid deceleration of the early rapid filling velocity consistent with right ventricular restrictive physiology would promote LCOS (26). Obstructed venous return and visceral congestion caused by elevated CVP may further result in decreased cardiac output (27). To mitigate this issue, negative pressure ventilation is applied to reduce intrathoracic pressure to increase the systemic venous return, and thus improve the cardiac output (28).
This study demonstrated that lower MBP was also an independent predictor for postoperative LCOS. MBP is related to cardiac output and systemic vascular resistance and also reflects left ventricular end-diastolic pressure. The right ventricular restrictive physiology impairs the left ventricular diastolic function via ventricular interaction which would lead to decreased MBP (29). The decrease in compliance of the left ventricle might also result from the curvature of the ventricular septum into the left ventricle as a result of right ventricular hypertension (26). To maintain cardiac output during the early postoperative period, surgeons may choose to leave an atrial septal defect to allow for right-to-left shunt (30). In addition, lower MBP would further reduce blood supply to the heart and damage cardiac function.
This study demonstrated that longer CPB and ACC time were also associated with postoperative LCOS. Previous studies also demonstrated that cardiopulmonary bypass would cause postoperative myocardial injury and dysfunction because of inflammatory response and ischemic injury and longer CPB and ACC time would increase the risk for LCOS (22,31). Therefore by reducing CPB and ACC time, myocardial injury and the incidence of postoperative LCOS would also be reduced.
LCOS after TOF surgery in children mostly occurs within 24 hours after the surgical repair, indicating that the identifying of preoperative and intraoperative risk factors of LCOS and early LCOS prediction are essential which can provide an early warning so as to achieve prevention as early as possible. Meanwhile, medical staff can alter preoperative and intraoperative modifiable factors to reduce the risk of postoperative LCOS. Early identification of LCOS and timely intervention are effective measures to achieve good outcomes. It is vital to perform frequent clinical evaluation and continuous monitoring for early recognition of LCOS. Common signs of LCOS after TOF repair include tachycardia, elevated right atrial pressure or central venous pressures, low blood pressure, peripheral hypoperfusion, cold limbs, hepatomegaly, oliguria, decreased mixed venous oxygen saturation, and/or acidosis. Management of LCOS varies depending on the source of dysfunction, because preload, afterload, myocardial contractility, heart rate, and/or rhythm can be altered. Maintenance of normal sinus rhythm, right ventricular preload, appropriate myocardial support, and ventilation are key in prevention of LCOS after repair of TOF (4).
Several limitations present in this study. First, this study is a single-center retrospective analysis with a small sample size, hence, the application of the findings here to other populations and institutions needs to be validated in further prospective large-scale multi-center studies. Second, surgical strategy in the study was determined by the cardiac surgeons, and the selection of measurement indicators was not comprehensive, potentially limiting the generalizability of the results found in the study. Third, there were substantial differences in the definitions of the outcomes in different studies, and the variability of the clinical judgment of the intensivists was another possible confounder. Fourth, the study was limited to patients undergoing radical repair. Patients undergoing palliative surgical repair for functionally univentricular circulations were excluded due to the distinct hemodynamic characteristics. Therefore, the applicability of the prediction model to such patients cannot be determined.
Conclusions
In conclusion, this study is the first to incorporate pre- and intraoperative characteristics to develop a predictive model for LCOS after surgical repair of TOF in children. This model showed good discrimination, good fit and clinical benefits.
Acknowledgments
Funding: This study was supported by the Chinese National Natural Science Foundation of China (Nos. 82170307, and 81970267).
Footnote
Reporting Checklist: The authors have completed the TRIPOD reporting checklist. Available at https://tp.amegroups.com/article/view/10.21037/tp-22-498/rc
Data Sharing Statement: Available at https://tp.amegroups.com/article/view/10.21037/tp-22-498/dss
Conflicts of Interest: All authors have completed the ICMJE uniform disclosure form (available at https://tp.amegroups.com/article/view/10.21037/tp-22-498/coif). The authors have no conflicts of interest to declare.
Ethical Statement: The authors are accountable for all aspects of the work in ensuring that questions related to the accuracy or integrity of any part of the work are appropriately investigated and resolved. The study was conducted in accordance with the Declaration of Helsinki (as revised in 2013). The study was approved by the Institutional Review Board of Shanghai Children’s Medical Center (No. SCMCIRB-K2022071-1), and informed consent was obtained from all the parents of the patients.
Open Access Statement: This is an Open Access article distributed in accordance with the Creative Commons Attribution-NonCommercial-NoDerivs 4.0 International License (CC BY-NC-ND 4.0), which permits the non-commercial replication and distribution of the article with the strict proviso that no changes or edits are made and the original work is properly cited (including links to both the formal publication through the relevant DOI and the license). See: https://creativecommons.org/licenses/by-nc-nd/4.0/.
References
- Apitz C, Webb GD, Redington AN. Tetralogy of Fallot. Lancet 2009;374:1462-71. [Crossref] [PubMed]
- Wang LC, Li SK, Zhu FT, et al. The effect of transesophageal echocardiography in the surgical treatment of tetralogy of Fallot. Eur Rev Med Pharmacol Sci 2018;22:2084-7. [PubMed]
- Lukács L, Kassai I, Arvay A. Total correction of tetralogy of Fallot in adolescents and adults. Thorac Cardiovasc Surg 1992;40:261-5. [Crossref] [PubMed]
- Forman J, Beech R, Slugantz L, et al. A Review of Tetralogy of Fallot and Postoperative Management. Crit Care Nurs Clin North Am 2019;31:315-28. [Crossref] [PubMed]
- Hu LW, Xiang Y, Qin SY, et al. Vortex formation time as an index of left ventricular filling efficiency: comparison between children volunteers and patients with tetralogy of Fallot. Transl Pediatr 2022;11:869-81. [Crossref] [PubMed]
- Wang P, Fu C, Bai G, et al. Risk factors of postoperative low cardiac output syndrome in children with congenital heart disease: A systematic review and meta-analysis. Front Pediatr 2022;10:954427. [Crossref] [PubMed]
- Richardson JP, Clarke CP. Tetralogy of Fallot. Risk factors associated with complete repair. Br Heart J 1976;38:926-33. [Crossref] [PubMed]
- Balachandran VP, Gonen M, Smith JJ, et al. Nomograms in oncology: more than meets the eye. Lancet Oncol 2015;16:e173-80. [Crossref] [PubMed]
- Liu H, Zheng SQ, Li XY, et al. Derivation and Validation of a Nomogram to Predict In-Hospital Complications in Children with Tetralogy of Fallot Repaired at an Older Age. J Am Heart Assoc 2019;8:e013388. [Crossref] [PubMed]
- Liu H, Lu ZJ, Zhang SP, et al. Association of perfusate oxygenation with cardiovascular disorder in tetralogy of fallot children: A nested case-control study. J Card Surg 2021;36:3607-18. [Crossref] [PubMed]
- Liu H, Zheng SQ, Zeng ZH, et al. Association Between Perfusate Oxygenation and Acute Lung Injury in Tetralogy of Fallot Surgery. Shock 2020;54:21-9. [Crossref] [PubMed]
- Hoffman TM, Wernovsky G, Atz AM, et al. Efficacy and safety of milrinone in preventing low cardiac output syndrome in infants and children after corrective surgery for congenital heart disease. Circulation 2003;107:996-1002. [Crossref] [PubMed]
- Hummel J, Rücker G, Stiller B. Prophylactic levosimendan for the prevention of low cardiac output syndrome and mortality in paediatric patients undergoing surgery for congenital heart disease. Cochrane Database Syst Rev 2017;8:CD011312. [PubMed]
- Parr GV, Blackstone EH, Kirklin JW. Cardiac performance and mortality early after intracardiac surgery in infants and young children. Circulation 1975;51:867-74. [Crossref] [PubMed]
- Samadi M, Malaki M, Ghaffari S, et al. Correlation Between Pediatric Open Heart Surgery Outcomes and Arterial-mixed Venous Oxygen Saturation Differences. J Cardiovasc Thorac Res 2012;4:41-4. [PubMed]
- Drennan SE, Burge KY, Szyld EG, et al. Clinical and Laboratory Predictors for the Development of Low Cardiac Output Syndrome in Infants Undergoing Cardiopulmonary Bypass: A Pilot Study. J Clin Med 2021;10:712. [Crossref] [PubMed]
- Vittinghoff E, McCulloch CE. Relaxing the rule of ten events per variable in logistic and Cox regression. Am J Epidemiol 2007;165:710-8. [Crossref] [PubMed]
- Li VW, Yu CK, So EK, et al. Ventricular Myocardial Deformation Imaging of Patients with Repaired Tetralogy of Fallot. J Am Soc Echocardiogr 2020;33:788-801. [Crossref] [PubMed]
- Roche SL, Grosse-Wortmann L, Friedberg MK, et al. Exercise echocardiography demonstrates biventricular systolic dysfunction and reveals decreased left ventricular contractile reserve in children after tetralogy of Fallot repair. J Am Soc Echocardiogr 2015;28:294-301. [Crossref] [PubMed]
- Sunakawa A, Shirotani H, Yokoyama T, et al. Factors affecting biventricular function following surgical repair of tetralogy of Fallot. Jpn Circ J 1988;52:401-10. [Crossref] [PubMed]
- Akiba T, Nakasato M, Sato S, et al. Left and right ventricular volume characteristics in tetralogy of Fallot and their relationship to arterial oxygen saturation and age. Acta Paediatr Jpn 1996;38:657-60. [Crossref] [PubMed]
- Sandeep B, Huang X, Xu F, et al. Etiology of right ventricular restrictive physiology early after repair of tetralogy of Fallot in pediatric patients. J Cardiothorac Surg 2019;14:84. [Crossref] [PubMed]
- Hoang TT, Manso PH, Edman S, et al. Genetic variants of HIF1α are associated with right ventricular fibrotic load in repaired tetralogy of Fallot patients: a cardiovascular magnetic resonance study. J Cardiovasc Magn Reson 2019;21:51. [Crossref] [PubMed]
- Zhou N, Liu L, Zou R, et al. Circular Network of Coregulated Sphingolipids Dictates Chronic Hypoxia Damage in Patients With Tetralogy of Fallot. Front Cardiovasc Med 2021;8:780123. [Crossref] [PubMed]
- Li RK, Mickle DA, Weisel RD, et al. Effect of oxygen tension on the anti-oxidant enzyme activities of tetralogy of Fallot ventricular myocytes. J Mol Cell Cardiol 1989;21:567-75. [Crossref] [PubMed]
- Cullen S, Shore D, Redington A. Characterization of right ventricular diastolic performance after complete repair of tetralogy of Fallot. Restrictive physiology predicts slow postoperative recovery. Circulation 1995;91:1782-9. [Crossref] [PubMed]
- Su L, Pan P, Li D, et al. Central Venous Pressure (CVP) Reduction Associated With Higher Cardiac Output (CO) Favors Good Prognosis of Circulatory Shock: A Single-Center, Retrospective Cohort Study. Front Med (Lausanne) 2019;6:216. [Crossref] [PubMed]
- Shekerdemian LS, Bush A, Shore DF, et al. Cardiorespiratory responses to negative pressure ventilation after tetralogy of fallot repair: a hemodynamic tool for patients with a low-output state. J Am Coll Cardiol 1999;33:549-55. [Crossref] [PubMed]
- Ahmad N, Kantor PF, Grosse-Wortmann L, et al. Influence of RV restrictive physiology on LV diastolic function in children after tetralogy of Fallot repair. J Am Soc Echocardiogr 2012;25:866-73. [Crossref] [PubMed]
- Sharkey AM, Sharma A. Tetralogy of Fallot: anatomic variants and their impact on surgical management. Semin Cardiothorac Vasc Anesth 2012;16:88-96. [Crossref] [PubMed]
- Chaturvedi RR, Shore DF, Lincoln C, et al. Acute right ventricular restrictive physiology after repair of tetralogy of Fallot: association with myocardial injury and oxidative stress. Circulation 1999;100:1540-7. [Crossref] [PubMed]