Highly expressed miR-210 in the peripheral blood is closely associated with asphyxiated neonates
Highlight box
Key findings
• miR-210 was highly expressed in the peripheral blood of asphyxiated neonates.
• miR-210 has a certain diagnostic value for neonatal asphyxia.
What is known and what is new?
• Literature reports that the miR-210 expression is significantly correlated with the hypoxia signaling pathways.
• The high expression of miR-210 in the peripheral blood of neonates with asphyxia may be related to the cerebral anoxic injury.
What is the implication, and what should change now?
• miR-210 can be applied in the auxiliary diagnosis and treatment of asphyxiated newborns.
Introduction
In China, neonatal brain injury caused by asphyxia is one of the most common reasons for neonatal disability and death. Asphyxia has high rates of morbidity and disability, with an unclear mechanism (1). Traditional indicators and clinical symptoms have limited specificity for diagnosing neonatal brain injury caused by asphyxia, and subjective evaluations are primarily used in clinical settings; however, they also have limited sensitivity and specificity, insufficient stability, and often lag in terms of pathophysiological changes (2). Each individual’s tolerance to hypoxia varies greatly, making it difficult to diagnose and prognostically predict neonatal brain injury caused by asphyxia early. Neonatal brain injury caused by asphyxia is an issue tormenting the field of pediatrics. Therefore, it is crucial to untimely identify asphyxial hypoxia before the initiation of brain injury by using an early targeted treatment to block the injury.
MicroRNAs (miRNAs) are a class of noncoding small-molecule RNAs that can rapidly and efficiently degrade target messenger RNAs (mRNAs) or inhibit protein translation by binding to target sites (3,4). miRNAs play a role in almost all physiological and pathological processes and can act as early predictive molecular markers of diseases (5-7). A study revealed that when the brain tissue is damaged, brain miRNAs are altered and secreted into the peripheral blood circulation (8). Therefore, miRNAs can serve as diagnostic markers of brain tissue injury (9). Furthermore, related animal experiments have suggested that miR-210 expression is downregulated in the brain tissue of model rats and that it participates in the process of brain injury (10). To date, however, only a few clinical studies have reported the role of miR-210 in the diagnosis of brain injury in neonates with early asphyxia.
In the present study, we determined the changes in miR-210 expression in the peripheral blood of neonates with asphyxia and analyzed the correlation between miR-210 and clinical indicators of brain injury in early asphyxia. Furthermore, we elucidated the clinical characteristics of its upregulation in predicting brain injury prognosis. Moreover, we identified the miR-210 target gene-related pathways and diseases and analyzed the network interactions among the miR-210 target genes associated with neurological and cardiovascular diseases. We present this article in accordance with the STARD reporting checklist (available at https://tp.amegroups.com/article/view/10.21037/tp-23-106/rc).
Methods
Patient information
The study subjects were 27 neonates with asphyxia (gestational age >37 weeks) who had been admitted to the Neonatology Department of The Third People’s Hospital of Dongguan between January 2015 and December 2016. The control group comprised 26 normal neonates who were recruited during the same period. The patients in the asphyxia group have a gestational age of 37–42 (40.62±0.71) weeks and weighed 2.6–4.1 (3.55±0.42) kg. The patients in the control group had a gestational age of 37–42 (40.62±0.74) weeks and weighed 2.6–4.4 (3.59±0.45) kg. The neonates in the control group had no diseases. A difference test was conducted to determine the comparability of the groups for analysis. All the study participants’ parents signed an informed consent form consenting to their child’s inclusion in the study. The study was conducted according to the Declaration of Helsinki (as revised in 2013) and approved by the Ethics Committee of The Third People’s Hospital of Dongguan (No. 2022-KYSB-014). None of the selected patients were treated for mild hypothermia.
The inclusion criteria were formulated according to the diagnostic criteria for neonatal asphyxia jointly formulated by the American Academy of Pediatrics and the College of Obstetrics and Gynecology in 1996 and were as follows: neonates (I) with severe metabolic/mixed acidosis (pH <7.00) in the umbilical artery blood; (II) with an Apgar score of 0–3 points and duration of >5 min; (III) with early neurological manifestations, such as convulsions, coma, or hypotonia; and (IV) with evidence of multiple organ dysfunction during premature delivery. The patients who met the above criteria were diagnosed with asphyxia. Neonates who met the abovementioned criteria and had multiorgan (≥3 organs) damage and/or hypoxic–ischemic encephalopathy were diagnosed with severe asphyxia.
The exclusion criteria were as follows: (I) neonates with other serious diseases that may have affected the comparability of the present study, such as a congenital genetic metabolic disease, neonatal hemorrhage, neonatal hemolytic disease, septic shock, and birth trauma owing to intracranial hemorrhage, and/or (II) those who were diagnosed with hypoxic-ischemic encephalopathy (HIE) and were excluded after 2 weeks of treatment and observation as well as based on laboratory results and head magnetic resonance imaging (MRI).
HIE was diagnosed based on diagnostic criteria, clinical symptoms, Apgar score, and potential of hydrogen (pH) of umbilical cord blood at delivery. Figure 1 presents the study flow chart.
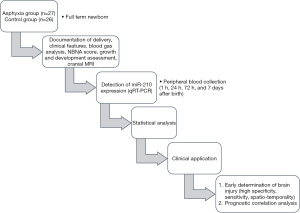
Quantitative real-time polymerase chain reaction
Peripheral blood (1 mL) was collected from the patients in each group within 1 h of their birth. According to the gene sequences, Primer Premier 5 (Premier, Canada) was used to design the primers. Next, total RNA and miRNA were extracted using the TRIzol kit or HiPure Blood/Liquid RNA Kit (Magen, R4163) according to the manufacturer’s instructions. RNA quality was determined using an ultra-micro ultraviolet spectrophotometer to determine RNA concentration and purity. For RNA purity detection, an A260/A280 ratio of 1.8–2.1 indicated that the purity was qualified. Agarose gel electrophoresis was performed to determine the quality of total RNA in the sample. RNA was dissolved in RNA kinase-free water and stored at −80 ℃. Complementary DNA reverse transcription was performed using M-MLV Reverse Transcriptase (Promega, M1705) using the following experimental system: 2 µg of RNA, 1 µL of Primer Mix, and RNase-free water to 15 µL; 5 min at 70 ℃ and 5 min on ice; 5×5 µL of buffer, 6.25 µL of 2 mM dNTP, 1 µL of M-MLV, and 12.75 µL of RNase-free water, for a total reaction mix of 25 µL; and 60 min at 42 ℃. Next, amplification was performed using the ChamQ SYBR qPCR Master Mix (Vazyme, Q341-03). The following formula was used to calculate the relative expression of the target gene: 2−ΔΔCt = 2 − [(ΔCt) Test − (ΔCt) Control].
Gene ontology (GO) and Kyoto Encyclopedia of Genes and Genomes (KEGG) analyses
The DAVID database (https://david.ncifcrf.gov/tools.jsp) was used to determine the targeted genes via classical GO and KEGG pathway enrichment analyses. The data were processed using the cluster Profiler package in R (version 3.14.3) and the org.Hs.eg.db package (version 3.10.0). GO analysis was performed to elucidate the biological processes (BPs) of the genes, which can be used to predict protein function. Furthermore, KEGG pathway analysis was conducted to assign the differentially expressed genes to specific pathways to identify the metabolic pathways that were significantly altered in the disease states.
Construction of the protein-protein interaction (PPI) network
First, the miR-210 target genes involved in neurological or cardiovascular diseases were imputed into the STRING database (https://string-db.org/); the combined score was set to >0.4. Next, the calculation results were imported into Cytoscape-v3.8.2 to construct the PPI network. The line between two points in the PPI network diagram represents the relationship between two genes with direct effects.
Statistical analysis
Statistical analysis was performed using SPSS 21.0 software (SPSS, Armonk, NY, USA). The normally distributed measurement data were presented as mean and standard deviation ( ± standard deviation). Differences between the groups were compared using the t-test. The non-normally distributed measurement data were presented as a median and interquartile range [M (Q)]. The rank-sum test was used to compare the differences between the groups. The count data were presented as the number of cases and percentage [n (%)]. The exact probability method was used to compare the differences between the groups. A P value of <0.05 was considered statistically significant.
Results
miR-210 was highly expressed in the peripheral blood of neonates with asphyxia
We observed that miR-210 expression was significantly upregulated in the asphyxia group compared with the control group (Figure 2A and Table 1). However, we observed that the number of normal deliveries, cord pH, and 1-, 5-, and 10-min Apgar scores were markedly decreased in the asphyxia group compared with the control group (Table 1). We also elucidated the relationship between miR-210 expression and pathological indicators and observed that miR-210 expression was not related to 1-, 5-, and 10-min Apgar scores in the HIE group; the possible reason for this may be the insufficient sample size of our study (Figure 2B).
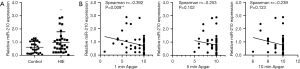
Table 1
Index | Control | HIE | Statistical value | P value |
---|---|---|---|---|
Gender (male/female) | 14/9 | 16/10 | χ2=0.002 | 0.962 |
Mode of delivery (normal/cesarean)* | 19/4 | 13/13 | χ2=4.379 | 0.036 |
Cord pH (mean ± SD)** | 7.40±0.04 | 7.21±0.15 | t=5.964 | 0 |
1-min Apgar (mean ± SD)** | 9.76±0.56 | 7.08±2.38 | t=5.523 | 0 |
5-min Apgar (mean ± SD)** | 10 | 8.58±1.55 | t=4.470 | 0 |
10-min Apgar (mean ± SD)** | 10 | 9.04±1.18 | t=4.146 | 0 |
miR-210 expression level (mean ± SD)* | 0.61±0.44 | 0.99±0.80 | t=–2.194 | 0.034 |
*, P<0.05; **, P<0.01. SD, standard deviation; HIE, hypoxic-ischemic encephalopathy.
The relationship between miR-210 expression and the clinical indicators
The incidence of coma, low muscle tension, and respiratory distress in the miR-210 high-expression group were higher than those in the miR-210 low-expression group (Table 2), and the difference was statistically significant. However, no significant differences were found in white blood cell (WBC) numbers, procalcitonin (PCT) levels, pH, neonatal behavioral neurological assessment (NBNA) abnormalities, abnormal imaging examinations, clinical irritation, and high muscle tension between the two groups.
Table 2
Index | miR-210 low-expression group (n=10) | miR-210 high-expression group (n=17) | Z/t | P |
---|---|---|---|---|
miR-210 [M (Q)] | 0.25 (0.28) | 1.17 (1.17) | −4.452 | <0.001 |
WBC (mean ± SD, ×109) | 19.61±7.36 | 15.20±7.57 | 1.539 | 0.135 |
PCT [M (Q), ng/mg] | 0.17 (2.44) | 0.57 (5.49) | −0.360 | 0.719 |
pH (mean ± SD) | 7.28±0.11 | 7.19±0.17 | 1.822 | 0.080 |
Abnormal NBNA, n (%) | 2 (18.2) | 7 (38.9) | – | 0.412 |
Abnormal examination, n (%) | 5 (45.5) | 3 (16.7) | – | 0.197 |
Clinical irritation, n (%) | 3 (16.7) | 5 (45.5) | – | 0.197 |
Coma, n (%) | 0 (0.0) | 3 (27.3) | – | 0.045 |
High muscle tone, n (%) | 2 (11.1) | 5 (45.5) | – | 0.071 |
Low muscle tone, n (%) | 0 (0.0) | 5 (45.5) | – | 0.004 |
Respiratory distress, n (%) | 4 (22.2) | 10 (90.9) | – | <0.001 |
The miR-210 high expression group was higher than the average value of the control group, while the miR-210 low expression group was lower than the average value. M (Q), median (interquartile range); WBC, white blood cell; SD, standard deviation; NBNA, neonatal behavioral neurological assessment.
Relationship between miR-210 expression and the indicators of neonatal asphyxia
Differences in miR-210 expression between the normal and abnormal NBNA groups, normal and abnormal low-pH groups, and normal image and abnormal impact examination groups were not significant (Table 3). miR-210 expression in the severe clinical symptom group was significantly higher than that in the mild clinical symptom group.
Table 3
Project | Group | Number | miR-210 | Z/t | P |
---|---|---|---|---|---|
NBNA [M (Q)] | Normal | 21 | 0.74 (0.93) | −1.233 | 0.218 |
Abnormal | 6 | 1.04 (1.81) | |||
Low pH [M (Q)] | Normal | 12 | 1.17 (0.92) | −0.659 | 0.510 |
Abnormal | 15 | 0.76 (0.75) | |||
Image inspection (mean ± SD) | Normal | 12 (total: 19; 7 missed) | 1.01±0.65 | 0.276 | 0.786 |
Abnormal | 8 | 0.93±0.73 | |||
Clinical symptoms [M (Q)] | Severe | 14 | 1.17 (1.21) | 1.990 | 0.047 |
Mild | 13 | 0.52 (0.60) |
Image inspection: the brain magnetic resonance imaging data of 7 patients were missing due to the dispermission of their condition or the refusal of their family members. M (Q), median (interquartile range); SD, standard deviation; NBNA, neonatal behavioral neurological assessment.
Receiver operating characteristic (ROC) curve analysis of miR-210
The results of the ROC curve analysis showed that the area under the curve was 0.671 (0.526–0.816), which was statistically significant; thus, miR-210 appeared to possess a certain diagnostic value. The sensitivity of this diagnosis was 40.7%, and the specificity was 88.5% (Figure 3).
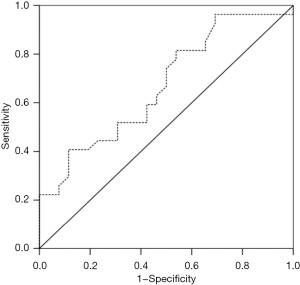
The potential target genes of miR-210 were associated with neurodevelopmental and cardiovascular diseases
We also performed a bioinformatics analysis of the target genes of miR-210 involved in neurodevelopmental and cardiovascular diseases. A total of 278 miR-210 target genes were associated with cardiovascular diseases, 670 target genes were associated with neurodevelopmental diseases, and 142 target genes were associated with both neurodevelopmental and cardiovascular diseases (Figure 4A). In the BP domain, the top 10 GO pathways associated with neurodevelopmental diseases included nervous system development, neurogenesis, neuron part, and neuron generation, differentiation, and development, whereas the top three GO pathways associated with cardiovascular and cerebrovascular diseases included cardiovascular and circulatory system development and circulatory system processes (Figure 4B).
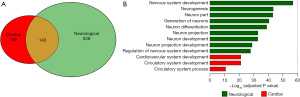
KEGG analysis of miR-210 target genes
We performed a KEGG pathway analysis of the target genes of miR-210 and found that the genes were mainly associated with metabolic pathways, cancer pathways, the phosphatidylinositol-3-kinase (PI3K)/serine-threonine kinase (Akt) signaling pathway, endocytosis, and the mitogen-activated kinase-like protein (MAPK) signaling pathway (Figure 5A). The KEGG disease analysis revealed that the target genes of miR-210 were mainly associated with nervous system diseases, congenital metabolism disorders, musculoskeletal diseases, cancers, cardiovascular diseases, and immune system diseases (Figure 5B).
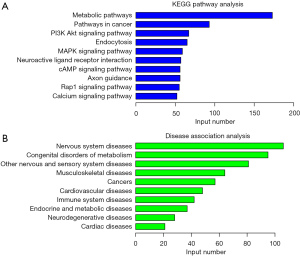
Analysis of the target genes of miR-210 associated with the regulation of neurological or cardiovascular diseases
Further analyses showed that 102 miR-210 target genes were associated with autism (Figure 6A), and 26 miR-210 target genes were associated with epilepsy (Figure 6B). We analyzed the network interactions of the target genes of miR-210 involved in neurological or cardiovascular diseases. We then identified several potential target genes associated with miR-210 and neurological or cardiovascular diseases using the network diagram (Figure 7).
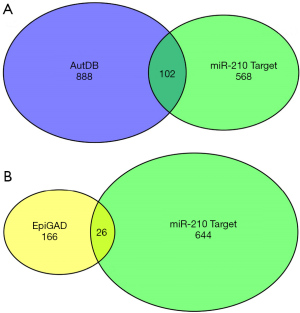
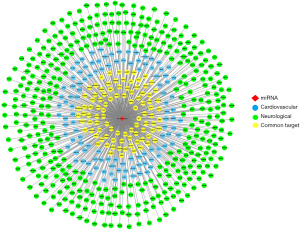
Discussion
Neonatal asphyxia is a systematic syndrome of multiple organ damage due to hypoxemia, hypercapnia, and acidosis caused by an inability to breathe spontaneously after birth, and its pathogenesis may involve antepartum, intrapartum, or postpartum intrauterine hypoxia and respiratory and circulatory disturbances during childbirth (11). The incidence rate of neonatal asphyxia in China is 13.9%, and 15.6% of these patients suffer from disabilities of different degrees (12). Furthermore, 25–50% of disabled children with cerebral malaria aged under one year have HIE (13). Moreover, neonatal asphyxia and associated complications account for 33% of all neonatal deaths (14). Pathologically, hypoxia mainly leads to cerebral edema and neuronal necrosis, whereas ischemia prominently causes cerebrovascular infarction and white-matter softening (15). A retrospective study showed that the incidence of organ damage after asphyxia was 85.0%, whereas that of multiple organ damage was 57.5% (16). Brain injury is the main form of injury after asphyxiation.
No objective criteria exist for the diagnosis, grading, and prognosis evaluation of brain injury after neonatal asphyxia. Existing criteria include an abnormal obstetric history, umbilical artery blood gas analysis, and the Apgar scoring system. The sensitivity, specificity, and stability of evaluation criteria based on neurological symptoms and their duration, MRI findings, and NBNA scores after birth are unsatisfactory and associated with a significant time lag in assessing the occurrence and development of brain damage.
miRNA is a 22-nt long endogenous non-coding RNA that affects target mRNA translation via the complementary pairing of base sequences (7,17). Recently, numerous animal experiments have shown the involvement of miRNAs in the preconditioning regulation of cerebral hypoxia, protection of nerve cells, mechanisms related to ischemic nerve injury and apoptosis, and regulation of nerve regeneration after ischemia (18,19). The regulatory effects of miRNAs are characterized by their high evolutionary sequence conservation and strict spatiotemporal and tissue specificity, thus exerting effects before the occurrence of pathophysiological changes (20). Studies have shown the involvement of miRNAs in protecting nerve cells from ischemic preconditioning, regulating ischemic nerve injury- and apoptosis-related mechanisms, and regulating nerve regeneration after ischemia (21-23).
Recent studies have shown angiogenesis in the ischemic injury area after cerebral ischemia, and miR-210 is an ischemia-specific miRNA (10,24). Its expression is stably upregulated in the hypoxic environment in vitro (25). The miRNA can promote nerve function recovery in ischemic regions; however, its underlying regulatory mechanisms have not yet been elucidated. A study has shown that hypoxia upregulates miR-210 expression in human umbilical vein endothelial 12 (HUVE-12) cells, thereby promoting HUVE-12 cells to form blood vessels in vitro (26). We are the first to confirm the role of miR-210 in promoting angiogenesis under hypoxia in vitro, which is the main pathophysiological factor occurring after cerebral ischemia. Further research is necessary to determine if miR-210 participates in the regulation of neovascularization after the occurrence of cerebral ischemia (27).
Herein, we revealed that miR-210 was highly expressed in the peripheral blood of neonates with asphyxia and possessed certain diagnostic values for diagnosing neonatal asphyxia. Further, we found that high miR-210 expression in the peripheral blood was associated with a significant increase in the incidence of coma, hypotonia, and respiratory distress, indicating its involvement in the occurrence of brain hypoxia injury after asphyxia and was positively correlated with the clinical manifestations of brain injury. No statistically significant differences were observed in mild asphyxia symptoms with low specificity, such as clinical agitation and high muscle tension, between the two groups. Similarly, no statistically significant differences were found in abnormal WBC numbers, PCT levels, pH, NBNA scores, and abnormal imaging examinations between the two groups. These findings suggest that the protective mechanism of miR-210 is unrelated to the WBC and PCT systems.
A change in the pH of the umbilical cord blood indicates the blood pH of the body during a relatively short period at the time of delivery. Children with ischemia and hypoxia in the perinatal period might be treated effectively to reverse the internal environment of asphyxia and hypoxia before brain cell asphyxia damage occurs. Treatment conditions and individual differences in newborns’ tolerance to hypoxia affect the final degree of brain cell damage. The NBNA score partly reflects the recovery state of children after brain injury. Abnormal NBNA scores in the miR-210 high-expression group were lower than those in the miR-210 low-expression group. Despite the low P value, the results suggested that miR-210 expression was probably involved in brain protection. Nonetheless, future comprehensive studies with larger samples and multiple phases, such as 2 days and 7 days after asphyxia, need to be performed to verify these findings. For the in-depth analysis, the asphyxia group was divided into the normal and abnormal NBNA groups, normal and abnormal pH-value groups, and normal image examination and abnormal impact examination groups according to each clinical index for comparison; however, no significant differences were found in miR-210 expression among these groups. miR-210 expression in the severe clinical symptom group was notably higher than that in the mild clinical symptom group, and the difference was statistically significant, supporting the above results.
The bioinformatics analysis revealed that 142 target genes of miR-210 were associated with both neurodevelopmental and cardiovascular diseases, suggesting that miR-210 might participate in neurodevelopmental and cardiovascular disease progression. The miR-210 target genes were also associated with the metabolic, cancer, PI3K/Akt, and MAPK pathways, which can be examined as potential indicators while studying miR-210 target genes. Moreover, we also identified 102 and 26 miR-210 target genes associated with autism and epilepsy, respectively. The network interaction analysis showed miR-210 target genes associated with neurological or cardiovascular diseases. The autism- and epilepsy-related miR-210 target genes predicted based on the database require long-term follow-up and further investigation. Moreover, we plan to analyze more relevant clinical variables in our future study.
Conclusions
Based on the relationship between miR-210 expression and each clinical observation and prognosis, we suggest that miR-210 can be used as a new indicator of post-asphyxial hypoxic-ischemic brain injury. We found that these miR-210 target genes were associated with neurodevelopmental and cardiovascular diseases, as well as with autism and epilepsy. We further identified several miR-210 target genes associated with neurological or cardiovascular diseases using the PPI network maps. Thus, this study provides a basis for further research on using miR-210 in treating newborns with asphyxia and those with neurological or cardiovascular diseases.
Acknowledgments
Funding: This work was supported by the Dongguan Social Science and Technology Development Project (No. 2014108101011).
Footnote
Reporting Checklist: The authors have completed the STARD reporting checklist. Available at https://tp.amegroups.com/article/view/10.21037/tp-23-106/rc
Data Sharing Statement: Available at https://tp.amegroups.com/article/view/10.21037/tp-23-106/dss
Peer Review File: Available at https://tp.amegroups.com/article/view/10.21037/tp-23-106/prf
Conflicts of Interest: All authors have completed the ICMJE uniform disclosure form (available at https://tp.amegroups.com/article/view/10.21037/tp-23-106/coif). The authors have no conflicts of interest to declare.
Ethical Statement: The authors are accountable for all aspects of the work in ensuring that questions related to the accuracy or integrity of any part of the work are appropriately investigated and resolved. The study was conducted according to the Declaration of Helsinki (as revised in 2013). All the study participants’ parents signed an informed consent form consenting to their child’s inclusion in the study. This study was approved by the Ethics Committee of The Third People’s Hospital of Dongguan (No. 2022-KYSB-014).
Open Access Statement: This is an Open Access article distributed in accordance with the Creative Commons Attribution-NonCommercial-NoDerivs 4.0 International License (CC BY-NC-ND 4.0), which permits the non-commercial replication and distribution of the article with the strict proviso that no changes or edits are made and the original work is properly cited (including links to both the formal publication through the relevant DOI and the license). See: https://creativecommons.org/licenses/by-nc-nd/4.0/.
References
- McNally MA, Soul JS. Pharmacologic Prevention and Treatment of Neonatal Brain Injury. Clin Perinatol 2019;46:311-25. [Crossref] [PubMed]
- Lacaille H, Vacher CM, Bakalar D, et al. Impaired Interneuron Development in a Novel Model of Neonatal Brain Injury. eNeuro 2019;6:ENEURO.0300-18.2019.
- Lu TX, Rothenberg ME. MicroRNA. J Allergy Clin Immunol 2018;141:1202-7. [Crossref] [PubMed]
- Xiong Q, Su H. MiR-325-3p functions as a suppressor miRNA and inhibits the proliferation and metastasis of glioma through targeting FOXM1. J Integr Neurosci 2021;20:1019-28. [Crossref] [PubMed]
- Gierlikowski W, Gierlikowska B. MicroRNAs as Regulators of Phagocytosis. Cells 2022;11:1380. [Crossref] [PubMed]
- Saliminejad K, Khorram Khorshid HR, Soleymani Fard S, et al. An overview of microRNAs: Biology, functions, therapeutics, and analysis methods. J Cell Physiol 2019;234:5451-65. [Crossref] [PubMed]
- Niu L, Sun N, Kong L, et al. miR-634 inhibits human vascular smooth muscle cell proliferation and migration in hypertension through Wnt4/β-catenin pathway. Front Biosci (Landmark Ed) 2021;26:395-404. [Crossref] [PubMed]
- Ma Q, Zhang L, Pearce WJ. MicroRNAs in brain development and cerebrovascular pathophysiology. Am J Physiol Cell Physiol 2019;317:C3-C19. [Crossref] [PubMed]
- Pinchi E, Frati P, Arcangeli M, et al. MicroRNAs: The New Challenge for Traumatic Brain Injury Diagnosis. Curr Neuropharmacol 2020;18:319-31. [Crossref] [PubMed]
- Zhang H, Wu J, Wu J, et al. Exosome-mediated targeted delivery of miR-210 for angiogenic therapy after cerebral ischemia in mice. J Nanobiotechnology 2019;17:29. [Crossref] [PubMed]
- Solevåg AL, Schmölzer GM, Cheung PY. Novel interventions to reduce oxidative-stress related brain injury in neonatal asphyxia. Free Radic Biol Med 2019;142:113-22. [Crossref] [PubMed]
- Li Q, Cui H, Zheng D, et al. Effects of walking exercise during late trimester on pregnancy outcome of low-risk primipara. Zhonghua Yi Xue Za Zhi 2014;94:1722-5. [PubMed]
- Parikh P, Juul SE. Neuroprotective Strategies in Neonatal Brain Injury. J Pediatr 2018;192:22-32. [Crossref] [PubMed]
- Yelamali B, Panigatti P, Pol R, et al. Outcome of newborn with birth asphyxia in tertiary care hospital-a retrospective study. Medica Innovatica 2014;3:59-64.
- Beaudin AE, Waltz X, Hanly PJ, et al. Impact of obstructive sleep apnoea and intermittent hypoxia on cardiovascular and cerebrovascular regulation. Exp Physiol 2017;102:743-63. [Crossref] [PubMed]
- Liu S. Analysis of the incidence, degree and clinical characteristics of organ function damage in asphyxiated newborns. China Foreign Medical Treatment 2013;4:2-4.
- Li Y, Lu L, Wu X, et al. The Multifaceted Role of Long Non-Coding RNA in Gastric Cancer: Current Status and Future Perspectives. Int J Biol Sci 2021;17:2737-55. [Crossref] [PubMed]
- Tu Y, Hu Y. MiRNA-34c-5p protects against cerebral ischemia/reperfusion injury: involvement of anti-apoptotic and anti-inflammatory activities. Metab Brain Dis 2021;36:1341-51. [Crossref] [PubMed]
- Wang X, Chen S, Ni J, et al. miRNA-3473b contributes to neuroinflammation following cerebral ischemia. Cell Death Dis 2018;9:11. [Crossref] [PubMed]
- Boyd SD. Everything you wanted to know about small RNA but were afraid to ask. Lab Invest 2008;88:569-78. [Crossref] [PubMed]
- Yin KJ, Deng Z, Huang H, et al. miR-497 regulates neuronal death in mouse brain after transient focal cerebral ischemia. Neurobiol Dis 2010;38:17-26. [Crossref] [PubMed]
- Sun X, Wang L, Huang X, et al. Regulatory Mechanism miR-302a-3p/E2F1/SNHG3 Axis in Nerve Repair Post Cerebral Ischemic Stroke. Curr Neurovasc Res 2021;18:515-24. [Crossref] [PubMed]
- Yang CC, Wei XP, Fu XM, et al. Down-regulating microRNA-20a regulates CDH1 to protect against cerebral ischemia/reperfusion injury in rats. Cell Cycle 2021;20:54-64. [Crossref] [PubMed]
- Zaccagnini G, Greco S, Voellenkle C, et al. miR-210 hypoxamiR in Angiogenesis and Diabetes. Antioxid Redox Signal 2022;36:685-706. [Crossref] [PubMed]
- Zeng L, Liu J, Wang Y, et al. MicroRNA-210 as a novel blood biomarker in acute cerebral ischemia. Front Biosci (Elite Ed) 2011;3:1265-72. [PubMed]
- Fasanaro P, D'Alessandra Y, Di Stefano V, et al. MicroRNA-210 modulates endothelial cell response to hypoxia and inhibits the receptor tyrosine kinase ligand Ephrin-A3. J Biol Chem 2008;283:15878-83. [Crossref] [PubMed]
- Ivan M, Huang X. miR-210: fine-tuning the hypoxic response. Adv Exp Med Biol 2014;772:205-27. [Crossref] [PubMed]
(English Language Editor: L. Huleatt)