Behavior of immunoglobulin G antibodies for SARS-COV-2 in Mexican pediatric patients with comorbidities: a prospective comparative cohort study
Highlight box
Key findings
• Association between the presence of comorbidities and high levels of IgG units in pediatric patients with COVID-19. By the third month less than 35% have immunity.
What is known and what is new?
• In the adult population, IgG levels have been found with severe disease; it has also been reported that patients with comorbidities have more severe cases of infection.
• We describe the behavior of memory immunoglobulin (IgG) in pediatric patients and the differences between patients with comorbidities and severity.
What is the implication, and what should change now?
• We hope that our results contribute to fill this gap and thus provide data to support and guide vaccination programs, focused on pediatric patients and those with comorbidities, as well as establishing treatment and diagnosis in patients with high levels of IgG.
Introduction
Humanity has recently been affected by the COVID-19 pandemic; an acute respiratory illness caused by the coronavirus 2 that causes severe acute respiratory syndrome (SARS-CoV-2). The SARS-CoV-2 genome is positive single-stranded RNA which is part of the Coronaviridae family and the β-coronavirus genus. It has a 30 kilobases (Kb) genome and encodes multiple proteins including spike protein (S), envelope protein (E), membrane protein (M), nucleocapsid (N), and nonstructural proteins and uses angiotensin converting enzyme 2 (ACE-2) receptor to infect human cell (1). Most patients are asymptomatic or have moderate symptoms, but about 15% develop severe pneumonia and 5% acute respiratory distress syndrome (ARDS), septic shock or multiple organ failure. The virus anchors to the epithelial cells of the respiratory tract and migrates to the lower airways until it infects the alveolar cells. The lesions are characterized by diffuse (typically bilateral) alveolar damage, fibrinogen deposition, and hyaline membrane formation. Type II pneumocytes are victims of a high level of replication that occasionally generates robust immune responses, including a cytokine storm. Lung tissue injury and cytokine storm cause ARDS and respiratory failure (2). Given this scenario, it is encouraging to mention that there is evidence that the child population is less susceptible to this disease, and they represent 1–5% of the cases diagnosed by COVID-19 (3), the mortality is rare and have better prognosis. The clinical features of COVID-19 in children include fever, cough, and a large percentage of them are asymptomatic, which may contribute to transmission. However, it is not known why children are less susceptible than adults to COVID-19, it has been suggested that it could be due to differences in the expression or function of the ACE-2 receptor or differences in the function of the immune system (4) and the other hypothesis is that there may be a type of cross-immunity due to previous infection with other coronaviruses.
Humoral and cellular immunity are needed for COVID-19 recovery and protection against new episodes of the disease, however, the comprehension, of the immunity to SARS-CoV-2 continues to develop (5). Most people infected with SARS-CoV-2 show an antibody response between day 10 and day 21 after infection. Detection of antibodies in mild cases can be after four weeks or more and in some patients the antibodies are not detected. In 2020, Ni et al. established that the immunoglobulin M (IgM) and immunoglobulin G (IgG) antibodies developed during infection are mainly directed against the N and S proteins (6). The S protein includes S1 and S2 subunits that aid in host infection, while the N protein plays an important role in the transcription and replication of viral RNA (7). The presence of IgM, IgG and IgA, may be related to disease severity and a study in the adult population associated that IgA and IgG levels were much higher in patients with severe disease compared with patients with mild or moderate disease (7), It has also been reported that patients with comorbidities present with cases of more severe infection (8), however, longitudinal follow-up studies explain the dynamics of humoral response in the pediatric population are needed. A study published in the pediatric population that compare the behavior of the humoral response by the severity of the infection (9), however, it is not described in relation to the presence of comorbidities and the severity of the infection.
Some reports show that IgM antibodies are detected in more than 90% of patients with COVID-19 at 22 days post-infection, while IgG antibodies are detected in 100% of cases between days 17–19 post-infection. IgM and IgG concentrations increase for 3 weeks after the onset of symptoms, before beginning to decrease, mainly in asymptomatic patients or those with mild symptoms (10). Similarly, less than 40% have positive antibodies among patients within 1 week of symptom onset, rapidly increasing to 100% (total antibodies), 94.3% (IgM), and 79.8% (IgG) at 15 days (11).
More than two years after the pandemic of COVID-19 caused by the SARS-COV-2, there is a great lack of information and the role of antibody persistence in the development of long-lasting protection against the virus is controversial. However, to understand long-lasting immunity after the infection, a proper characterization of humoral immune response is required mainly in the pediatric population and even more so in the pediatric population with comorbidities. Our aim was to describe the behavior of memory immunoglobulins (IgG) in pediatric patients and to know if there is a difference between patients with comorbidities. We present this article in accordance with the STROBE reporting checklist (available at https://tp.amegroups.com/article/view/10.21037/tp-22-454/rc).
Methods
A prospective comparative cohort study, carried out in a single-center the Hospital Infantil de México Federico Gómez from June 2020 to January 2021, with a follow up of 6 months after the initial diagnosis. The study was conducted in accordance with the Declaration of Helsinki (as revised in 2013). The study was approved by the Ethics Committee of Hospital Infantil de México Federico Gómez (No. HIM-031-2021). A written statement of informed consent was obtained from the parent or guardian and as well as informed assent in cases in which it applied. All experiments involving SARS-CoV-2 were conducted in a biosafety level 2 laboratory.
For this study, we cover all the patients that was a confirmed case of SARS-Cov-2 from 1 month to 17 years 11 months with a positive real-time polymerase chain reaction (RT-PCR) test for SARS-CoV-2, the follow-up of the disease’s evolution and measurement of IgG antibodies was collected. We obtained the clinical data, and comorbidities like arterial hypertension, diabetes, obesity, and cancer, the initial symptoms were recorded as well as the evolution regarding the severity of COVID-19 and the need for hospitalization, intensive care unit or mechanical of the patients who had confirmed their participation by signing an informed consent form. Clinical data were obtained from all the study subjects and the convalescent stage was confirmed, defined as the absence of symptoms for more than 15 days in mild cases, and with negative RT-PCR test results for SARS-CoV-2. The follow up was carried out through medical consultation with an appointment every month that included direct interrogation, examination and peripheral blood collection for the IgG quantification.
The diagnosis was performed thru nasopharyngeal swabs in a Hanks Balanced Salt Solution. The extraction of ribonucleic acid (RNA) was carried out in an automated way with the QIAamp® Viral RNA Mini Accessory Set kit and the QIAcube Connect equipment. The viral genes ORF1ab, N, S, and the human RNase P gene (which allows for the assessment of the quality of the sample) were detected using the following commercial kits: Detection kit for 2019 Novel Coronavirus (2019-nCoV) RNA (RT-PCR-Fluorescence Probing) Cat. DA-930 (Daan Gene Co., Ltd., Sun Yat-sen University, China) and Applied Life Technologies Corporation, Thermofisher® Cat. A47532, in the thermocyclers QuantStudio 5 RT-PCR Systems, ThermoFisher® and CFX96 Real time system, C1000, Touch Thermal cycler, BIORAD®, following the manufacturers’ recommendations and the current operational guidelines established by the Institute of Epidemiological Diagnosis and Reference (InDRE).
Peripheral blood samples were collected each month during de 6 months of follow up for the determination of the induced humoral immune response in convalescent patients with COVID-19.
The detection of antibodies was carried out in peripheral blood using chemiluminescence microparticle immunoassay (CMIA). IgG class antibodies against the SARS-CoV-2 nucleocapsid protein were detected using an automated equipment ARCHITECT 11000SR, Abbott®, and values above 1.4 BAU/mL were considered positive. For the patients that did not express IgG the result was reported in the analysis as 0.
Statistical analyses
Statistical analyses were implemented using SPSS version 20 software (SPSS Inc., IBM, Armonk, NY, USA) and Excel. Qualitative variables were expressed in frequencies and percentages and quantitative variables in medians and interquartile ranges (Kolmogorov-Smirnov test demonstrated non-parametric behavior of the variables). A comparison test was performed using Chi-squared test and Fisher’s exact test. The Mann-Whitney U-test was used to measure the quantitative variables. For the repeated measures ANOVA was used to compare the means of the antibody levels. A two-sided P value <0.05 was considered statistically significant.
Results
During the study period, 237 patients presented positive RT-PCR for SARS-COV-2, 124 (52.3%) were male, with a median age of 8.5 years, 52 (22%) patients had some type of comorbidity and 110 patients (46%) were hospitalized for severe COVID-19.
Of the total number of patients with positive RT-PCR, 147 patients (62%) had a positive test for IgG, of the group with comorbidities there were 35 patients (23.8%) respectively, while of the group without comorbidities 60% expressed positive ranges for IgG, showing no differences according to sex or age (Table 1), In Table 2, we present the types of comorbidities we found in the patients. In the group of comorbidities, 17 patients (48.6%) required hospitalization due to severe COVID-19, of that 9 were males (53%) with no statistical significance.
Table 1
Variable | Total (n=147) | Without comorbidity (n=112) | With comorbidity (n=35) | P |
---|---|---|---|---|
Age in years†, median [IQR] | 10 [11] | 5 [9] | 7 [9] | 0.24 |
Male‡ | 73 (49.7%) | 56 (50.0%) | 17 (48.6%) | 0.51 |
Hospitalized for severe COVID-19‡ | 64 (43.5%) | 47 (42.0%) | 17 (48.6%) | 0.49 |
†, Mann-Whitney U test. ‡, χ2-tests. SARS-COV-2, severe acute respiratory syndrome coronavirus 2; IgG, immunoglobulin G.
Table 2
Comorbidities | Total (n=35) | Percentage |
---|---|---|
Oncological | 10 | 28.57 |
Genetic | 5 | 14.29 |
Cardiovascular | 4 | 11.43 |
Gastroenterology | 4 | 11.43 |
Autoimmune | 3 | 8.57 |
Neurological | 3 | 8.57 |
Pneumology | 3 | 8.57 |
Metabolic | 1 | 2.86 |
Renal | 1 | 2.86 |
Psychomotor retardation | 1 | 2.86 |
SARS-COV-2, severe acute respiratory syndrome coronavirus 2; IgG, immunoglobulin G.
Patients with comorbidities reach higher IgG levels than patients without comorbidities the basal titters were: 5.17 for patients without comorbidities vs. 6.96 for the group with comorbidities (P<0.001) (Figure 1). At the same time the percentage of positive expression is higher in the group with comorbidities. The percentage of decrease in the positivity of the test is similar in both groups, for the first month, they start with 60% and 67% for the group with comorbidities and without respective comorbidities, while for the sixth month it is 5.8% and 2.7% respectively (Figure 2).
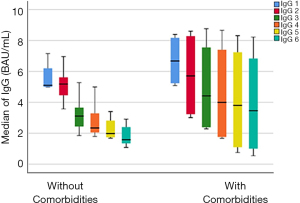
Of the 237 included in the study, 110 patients required hospital management. In Figure 3, we show that the IgG are higher in the patients that require hospital management due to moderate COVID-19 (P<0.001). In Table 3, we present the differences between the severity of COVID-19, the zeros represent that the patients did not express IgG against SARS-CoV-2 nucleocapsid protein. Patients with severe COVID-19 have more humoral responses with the production of more IgG than patients with mild COVID-19 (P=0.001).
Table 3
Mild COVID-19 (N=83) | Severe COVID-19 (N=64) | P† | |
---|---|---|---|
Male‡, N (%) | 44 (53.0) | 36 (56.3) | 0.7 |
Age in years, median [IQR] | 11 [7–15] | 10 [5–16] | 0.6 |
Comorbidities (yes) ‡, N (%) | 18 (21.7) | 17 (26.6) | 0.7 |
Respiratory symptoms‡, N (%) | 40 (48.2) | 52 (81.3) | 0.001 |
Gastrointestinal symptoms‡, N (%) | 43 (51.8) | 12 (18.8) | 0.001 |
IgG 1 BAU/mL, median (range) | 1.28 (0.42–2.8) | 3.09 (0.68–5.6) | 0.001 |
IgG 2 BAU/mL, median (range) | 0.36 (0.07–2.25) | 3.45 (1.07–5.14) | 0.001 |
IgG 3 BAU/mL, median (range) | 2.58 (2.24–2.9) | 2.28 (1.2–4.22) | 0.001 |
IgG 4 BAU/mL, median (range) | 1.62 (1.1–2.13) | 2.09 (0.78–3.7) | 0.001 |
IgG 5 BAU/mL, median (range) | 0 (0) | 3.76 (0.7–8.3) | 0.001 |
IgG 6 BAU/mL, median (range) | 0 (0) | 4.38 (0.5–8.2) | 0.001 |
†, Mann-Whitney U test. ‡, χ2-tests. SARS-COV-2, severe acute respiratory syndrome coronavirus 2; IgG, immunoglobulin G.
Discussion
In this study, we describe seroprevalence of Anti-SARS-CoV-2 IgG Antibodies in children characterized with the presence of comorbidities. Since March 2020, close to 7 million children have tested positive for SARS-CoV-2 in the United States (12). Levels of antibody represent important markers of protection against severe disease and/or hospitalization (13), in our study, 63% have positive Anti-SARS-CoV-2 IgG, this number is much higher than the reported by Mamishi et al. (14) that was 24% but they report that nearly half of the patients suffered from an underlying disease including cardiovascular or immunodeficiency disorders and malignancies while in our study was the 32%.
It is important to mention a key difference between the immune response of children and adults is that the former show a higher expression of pattern recognition receptors (the proteins that detect aberrant RNA) in the respiratory epithelium and in anti-gen-presenting cells, such as macrophages and dendritic cells. The increase in receptors such as MDA5 and RIG-1 is, in turn, related to a more robust innate response against SARS-CoV-2, compared to the average adult. Some pediatric patients with severe symptoms also show damage to the IFN-1 response system, demonstrating the importance of this system in the pathophysiology of the disease, both in the adult and pediatric population (15).
Despite its selective advantage, the spread of more infectious variants such as omicron showed that children are also susceptible to severe clinical symptoms.
Innate immunity also recruits and coordinates specific T and B leukocytes via chemokines. These cells, along with natural killer cells, are part of acquired immunity. In short, this process generates neutralizing antibodies against protein S, thus preventing the virus from entering cells. Another key difference between young and adult patients is the amount of virgin leukocytes, since the latter group has a smaller reservoir and, therefore, cannot generate memory antibodies in the appropriate proportion to avoid a second serious clinical picture.
Méndez-Echevarría et al. (9) report that pediatric patients with SARS-CoV-2 infection, have humoral response six months after the infection. All the children who required hospital admission remained seropositive, whereas one out of five children presenting with mild or asymptomatic infection, especially those presenting with low antibody titers, lost their antibodies; our results are different since by the sixth month only 6% of our patients with comorbidities presented positive IgG results. Data are scarce regarding the long-term humoral response in children (9); however, some pediatric series report a rate of antibody persistence below 30% after three months of infection and our results are related to these data. The immune response of children is mainly based on antibodies against the spike (S) protein, which the virus uses to enter cells, while antibodies against the N protein, essential for virus replication, are lacking. This lack of data could reflect the fact that children do not generally experience widespread infections (16).
It is evident that antibody responses decline in the months after the onset of symptoms; Yousefi et al. (17) concluded that despite the decrease in the amount of IgG antibodies over time, protective levels of anti-SARS-COV-2 could sustain for a lifetime in people who received a COVID-19 vaccine. The United States reported an increase in hospitalizations in children under five years of age (a population that as of March 2022 was not a candidate for vaccination) with 63% of hospitalized children without specific risk factors (18). The highest rate occurred in children under one year of age.
The spread of the disease is of concern because a proportion of patients develop post-infectious syndromes and/or chronic symptoms. In May 2020, the UK Royal College of Paediatrics and Child Health defined pediatric systemic inflammatory syndrome (PIMS) as the presence of persistent fever and evidence of dysfunction of one or more organs plus other findings, including partial or full criteria for Kawasaki disease in the absence of another infectious cause (19). It is estimated that 8 out of 100,000 patients of Hispanic or Latino origin will develop PIMS as a result of COVID-19. More importantly, a study in pediatric population (10 to 17 years old) shows that there are significant differences in the prevalence of dyspnea, respiratory problems, loss of smell, insomnia, and various other symptoms in patients with previous infection (20) therefore, vaccination in these countries should be focused on children between 6 months and 5 years of age who have not been included in the vaccination schedule and are the population that currently requires hospital treatment.
Cantini et al. (21) assessed a low IgG/IgM seroprevalence in a center that might help to define where the contagion risk is and need faster intervention and comment that there is still a lack about patients’ immunity duration, we hope that our results contribute to the filling of that lack and thus provide data to support and guide vaccination programs, focused on pediatric patients and with comorbidities.
The study has several limitations, first, the few variables that were included in the analysis, second, the few patients who had comorbidities and presented positive IgG, however, it was possible to have an insight characterization of the behavior of immunoglobulins.
Conclusions
In conclusion, we describe the Anti-SARS-CoV-2 IgG Antibodies among children by the presence of comorbidities in México and provide important insight about the pediatric immune response before COVID-19. We found an association between the presence of comorbidities and high levels of IgG units in pediatric patients with COVID-19. Additionally, patients with more severe course of the disease have higher levels of IgG and by the third month less than 35% have immunity, it is important to recognize that the immune response of the pediatric population to COVID-19 still has much to explore and understand.
Acknowledgments
Funding: This research was funded by Consejo Nacional de Ciencia y Tecnología (CONACYT) (No. CONACYT-PAACTI/312936).
Footnote
Reporting Checklist: The authors have completed the STROBE reporting checklist. Available at https://tp.amegroups.com/article/view/10.21037/tp-22-454/rc
Data Sharing Statement: Available at https://tp.amegroups.com/article/view/10.21037/tp-22-454/dss
Peer Review File: Available at https://tp.amegroups.com/article/view/10.21037/tp-22-454/prf
Conflicts of Interest: All authors have completed the ICMJE uniform disclosure form (available at https://tp.amegroups.com/article/view/10.21037/tp-22-454/coif). The authors have no conflicts of interest to declare.
Ethical Statement: The authors are accountable for all aspects of the work in ensuring that questions related to the accuracy or integrity of any part of the work are appropriately investigated and resolved. The study was conducted in accordance with the Declaration of Helsinki (as revised in 2013). The study was approved by the Ethics Committee of Hospital Infantil de México Federico Gómez (No. HIM-031-2021), and a written statement of informed consent was obtained from the parent/guardian and as well as informed assent in cases in which it applied.
Open Access Statement: This is an Open Access article distributed in accordance with the Creative Commons Attribution-NonCommercial-NoDerivs 4.0 International License (CC BY-NC-ND 4.0), which permits the non-commercial replication and distribution of the article with the strict proviso that no changes or edits are made and the original work is properly cited (including links to both the formal publication through the relevant DOI and the license). See: https://creativecommons.org/licenses/by-nc-nd/4.0/.
References
- Walls AC, Park YJ, Tortorici MA, et al. Structure, Function, and Antigenicity of the SARS-CoV-2 Spike Glycoprotein. Cell 2020;181:281-92.e6. Erratum in: Cell 2020;183:1735. [Crossref] [PubMed]
- Hu B, Guo H, Zhou P, et al. Characteristics of SARS-CoV-2 and COVID-19. Nat Rev Microbiol 2021;19:141-54. [Crossref] [PubMed]
- Ludvigsson JF. Systematic review of COVID-19 in children shows milder cases and a better prognosis than adults. Acta Paediatr 2020;109:1088-95. [Crossref] [PubMed]
- Balasubramanian S, Rao NM, Goenka A, et al. Coronavirus Disease 2019 (COVID-19) in Children - What We Know So Far and What We Do Not. Indian Pediatr 2020;57:435-42. [Crossref] [PubMed]
- Tian X, Bai Z, Cao Y, et al. Evaluation of Clinical and Immune Responses in Recovered Children with Mild COVID-19. Viruses 2022;14:85. [Crossref] [PubMed]
- Ni L, Ye F, Cheng ML, et al. Detection of SARS-CoV-2-Specific Humoral and Cellular Immunity in COVID-19 Convalescent Individuals. Immunity 2020;52:971-977.e3. [Crossref] [PubMed]
- Chen M, Qin R, Jiang M, et al. Clinical applications of detecting IgG, IgM or IgA antibody for the diagnosis of COVID-19: A meta-analysis and systematic review. Int J Infect Dis 2021;104:415-22. [Crossref] [PubMed]
- Long QX, Tang XJ, Shi QL, et al. Clinical and immunological assessment of asymptomatic SARS-CoV-2 infections. Nat Med 2020;26:1200-4. [Crossref] [PubMed]
- Méndez-Echevarría A, Sainz T, Falces-Romero I, et al. Long-Term Persistence of Anti-SARS-CoV-2 Antibodies in a Pediatric Population. Pathogens 2021;10:700. [Crossref] [PubMed]
- Long QX, Liu BZ, Deng HJ, et al. Antibody responses to SARS-CoV-2 in patients with COVID-19. Nat Med 2020;26:845-8. [Crossref] [PubMed]
- Zhao J, Yuan Q, Wang H, et al. Antibody Responses to SARS-CoV-2 in Patients With Novel Coronavirus Disease 2019. Clin Infect Dis 2020;71:2027-34. [Crossref] [PubMed]
- Zachariah P. COVID-19 in Children. Infect Dis Clin North Am 2022;36:1-14. [Crossref] [PubMed]
- Ward H, Whitaker M, Flower B, et al. Population antibody responses following COVID-19 vaccination in 212,102 individuals. Nat Commun 2022;13:907. [Crossref] [PubMed]
- Mamishi S, Esslami GG, Mohammadi M, et al. Detection of SARS-CoV-2 antibodies in pediatric patients: An Iranian referral hospital-based study. Hum Antibodies 2021;29:217-23. [Crossref] [PubMed]
- Loske J, Röhmel J, Lukassen S, et al. Pre-activated antiviral innate immunity in the upper airways controls early SARS-CoV-2 infection in children. Nat Biotechnol 2022;40:319-24. [Crossref] [PubMed]
- Nogrady B. How kids' immune systems can evade COVID. Nature 2020;588:382. [Crossref] [PubMed]
- Yousefi Z, Taheri N, Dargahi M, et al. Long-Term Persistence of Anti-SARS-COV-2 IgG Antibodies. Curr Microbiol 2022;79:96. [Crossref] [PubMed]
- Marks KJ, Whitaker M, Agathis NT, et al. Hospitalization of Infants and Children Aged 0-4 Years with Laboratory-Confirmed COVID-19 - COVID-NET, 14 States, March 2020-February 2022. MMWR Morb Mortal Wkly Rep 2022;71:429-36. [Crossref] [PubMed]
- Ulloa-Gutiérrez R, Ivankovich-Escoto G, Yamazaki-Nakashimada MA. Multisystem inflammatory syndrome associated with COVID19 in children and adolescents: calling for diagnosis. Síndrome inflamatorio multisistémico asociado a COVID-19 en niños y adolescentes: un llamado al diagnóstico. Rev Chilena Infectol 2020;37:199-201. [Crossref] [PubMed]
- Stephenson T, Pinto Pereira SM, Shafran R, et al. Physical and mental health 3 months after SARS-CoV-2 infection (long COVID) among adolescents in England (CLoCk): a national matched cohort study. Lancet Child Adolesc Health 2022;6:230-9. [Crossref] [PubMed]
- Cantini L, Bastianelli L, Lupi A, et al. Seroprevalence of SARS-CoV-2-Specific Antibodies in Cancer Patients Undergoing Active Systemic Treatment: A Single-Center Experience from the Marche Region, Italy. J Clin Med 2021;10:1503. [Crossref] [PubMed]