Pulmonary outcomes of congenital diaphragmatic hernia patients based on defect size (CDH Study Group Stage)
Highlight box
Key findings
• Large CDH defects (CDHSG C and D defects) are associated with worse long-term pulmonary outcomes.
• Impulse oscillometry can be useful tool for early detection of airway disease in CDH patients.
What is known and what is new?
• Previous studies have shown that postnatal morbidity is linked to diaphragmatic defect size.
• However, this study improves understanding of the substantial burden of pulmonary morbidity which persists following NICU discharge and introduces the value of impulse oscillometry in CDH patients.
What is the implication, and what should change now?
• These observations could be an essential component to identify at risk CDH groups who might benefit from a nuanced and comprehensive pulmonary management approach, by developing screening tools, protocolized management, and guidance for families regarding CDH-related long-term morbidities in accordance with defect size.
Introduction
Congenital diaphragmatic hernia (CDH) affects 1 in 2,000–3,000 newborns annually (1). CDH is a cascade of events beginning with an early embryologic insult involving a diaphragmatic defect allowing subsequent migration of abdominal organs into the thoracic cavity. This dual hit, including fundamental pulmonary vasculopathy, alongside mechanical compression of the lungs, results in pulmonary hypoplasia and abnormal pulmonary vascular growth, with ensuing cardiopulmonary sequelae (2,3). Survivorship has steadily increased, largely a consequence of advances in pre and postnatal medical care, including delayed surgical repair, refinements in extracorporeal life support (ECLS) (4), and progression toward standardized treatment protocols (5-10). Despite treatment advances, it is well-recognized that almost all CDH patients have some degree of pulmonary compromise and suffer from disease-specific long-term morbidity (11-13).
About 30–50% of CDH survivors subsequently experience long-term pulmonary complications as a result of overarching pulmonary hypoplasia, including pulmonary hypertension, chronic lung disease, exacerbated susceptibility to respiratory tract infections, ventilator-associated lung injury, and ventilatory impairments, with an increased rate of readmissions post neonatal intensive care unit (NICU) discharge (14).
Given the heterogenous clinical challenges, pulmonary function testing is the central component of disease characterization and management. Pulmonary function abnormalities are reported in 28–52% of patients, with obstructive ventilatory impairment being the predominant finding (15,16). Early diagnosis of pulmonary function abnormalities is instrumental to prevent long-term remodeling of the airways and improve prognosis. Impulse oscillometry (IOS), is a type of forced oscillation technique delivering a spectrum of frequencies on the airway during tidal breathing to determine lung function, and compared with spirometry, this test does not require the patient’s special cooperation, is effort independent, simple, noninvasive, repeatable, and provides comprehensive respiratory physiological parameters. IOS measurements can be used to identify and monitor the disease progression of asthma in at-risk younger patients (mainly over 3 years) (17).
Multiple studies have sought indicators and tools that can assist healthcare providers to predict post-discharge morbidity for CDH survivors (14). Several patient characteristics including prenatal diagnosis and major cardiac or chromosomal anomalies predict the types and frequency of morbidity that affect these infants in the postnatal inpatient setting (18-20); however, the staging system developed by the Congenital Diaphragmatic Hernia Study Group (CDHSG) based on diaphragmatic defect size is the strongest predictor of many outcomes, including morbidity at discharge (13).
In this study, defect size A and B are categorized as low-risk (LR) and defect size C and D as high-risk (HR) to compare pulmonary outcomes (Figure 1). We hypothesized that HR CDH survivors (C/D), as opposed to LR (A/B), experience more significant adverse pulmonary morbidities after discharge. Given the variable severity that characterizes this disease, these observations could be essential to identifying groups who might benefit from a comprehensive pulmonary management approach. In addition, the introduction of IOS testing to clinical practice could contribute to a better understanding of postnatal lung growth and the prevention of chronic lung morbidity. We present this article in accordance with the STROBE reporting checklist (available at https://tp.amegroups.com/article/view/10.21037/tp-23-14/rc).
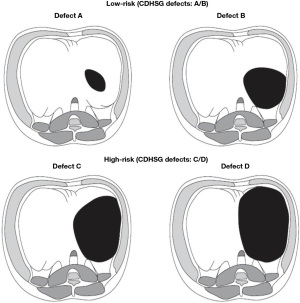
Methods
Study subjects
Patients with Bochdalek CDH (n=133) were seen between January 2012 and April 2022 at a single children’s hospital within an adult hospital system, with a subset seen at the comprehensive multidisciplinary CDH clinic (n=102), were enrolled. The study was conducted in accordance with the Declaration of Helsinki (as revised in 2013). The study was approved by the ethics committee of The University of Texas Health Science Center at Houston of Institutional Review Board approval (No. HCS-MS-18-1036) and individual consent for this retrospective analysis was waived. CDH survivors with other primary chronic pulmonary conditions, such as cystic fibrosis, primary ciliary dyskinesia, and immunodeficiency disorders with pulmonary manifestations were excluded.
Study participants were categorized based on hernia defect size as established by the CDHSG (21). Defects are categorized on an increasing gradient scale with “A” defects being the smallest, and “D” defects being the largest. “A” defect is the smallest, usually confined “intramuscular,” with >90% of the hemidiaphragm present; this defect involves <10% of the circumference of the chest wall. “B” defect is 50–75% of the hemidiaphragm present; this defect involves <50% of the chest wall. “C” defect is <50% of the hemidiaphragm present; this defect involves >50% of the chest wall. “D” defect is the largest (previously known as “agenesis”) with the complete or near complete absence of the diaphragm and <10% hemidiaphragm present; this defect involves >90% of the chest wall. Surgically, it is an absent posterior rim beyond the spine, an absent posterior-lateral rim, and an anterior/anterior-medial rim which is minuscule.
The categorization into LR (A/B) and HR (C/D) is appropriate based on the alignment of outcomes among these groups in previous publications (13,21). Pulmonary outcomes included: the prevalence of asthma, pulmonary hypertension, the need for ECLS, the number of days of mechanical ventilation during the NICU stay, oxygen at discharge, spirometry, IOS, and perfusion scans to determine compromise on the ipsilateral side.
NICU course and follow-up
The retrospective analysis included patients treated at a single children’s hospital within an adult hospital system NICU (n=133) and patients who were followed in our comprehensive multidisciplinary CDH clinic (n=102/133). Data on demographics, birth weight, gestational age, prenatal diagnosis, intrathoracic liver, observed/expected lung head circumference ratio (o/e LHR), type of repair, pulmonary hypertension at the time of discharge, the need for ECLS, need for oxygen at discharge, and a number of mechanical ventilation days were recorded. Pulmonary hypertension was based on echocardiogram findings of indirect signs of pulmonary hypertension such as increased tricuspid jet, elevated right ventricular systolic pressure, and/or interventricular septal flattening. In addition, for the purpose of this study, patients with evidence of pulmonary hypertension on echocardiogram and warranting treatment for pulmonary hypertension such as O2, sildenafil, and/or bosentan were included. CDH patients surviving NICU discharge were followed longitudinally to determine prospective events of asthma prevalence, all-cause rehospitalization (counting each rehospitalization as one event), emergency room visits (ER) (counting each visit as one event), and the total number of days of rehospitalizations. In addition, spirometry and IOS were performed on some of the patients followed at our comprehensive multidisciplinary CDH clinic.
Comprehensive multidisciplinary CDH clinic
A subset of 102 patients (77%) surviving hospital discharge whose families decided to continue care at the dedicated comprehensive multidisciplinary CDH clinic was followed longitudinally. All CDH patients have the opportunity for follow-up in the clinic, though patients managed prior to the establishment of the clinic did not have access. The comprehensive multidisciplinary CDH clinic was established in 2016 and central components include pediatricians, pediatric surgeons, pediatric pulmonologists, a dietician, a social worker, and a pediatric neuropsychologist. Comprehensive pulmonary management was delivered through the enhanced medical home with open access to manage acute respiratory conditions to the clinic, Monday through Friday. There is 24/7 direct access via phone to primary care physicians, who can schedule same/next day visits or call ER as needed on nights and weekends. In addition, this medical home has a low patient-provider ratio (≤1:100) and has weekly meetings to discuss all ER visits, hospital, and intensive care unit admissions. The follow-up visits are scheduled based on the CDH subtype (LR: A/B vs. HR: C/D). LR CDH patients were seen 2–4 weeks post-discharge, then every 3 months until 12 months of age, and annually thereafter. In contrast, HR CDH patients were followed more closely as follows: 2–4 weeks post-discharge, monthly visits until 3 months of age, every 3 months until 2 years of age, every 6 months until 4 years of age, and then annually. Patients were assessed for risk of pulmonary complications at each visit.
Asthma
Asthma diagnosis was determined post-NICU discharge based on the pulmonologist’s clinical assessment, considering clinical history, physical examination findings, and pulmonary function tests (IOS and spirometry) for patients seen at the comprehensive multidisciplinary CDH clinic (22-26). Spirometry and IOS were performed to determine the prevalence of asthma in the subset of patients who were ≥3 years of age for IOS, and ≥5 years of age for spirometry and/or were able to perform the maneuvers. For patients not seen in our comprehensive multidisciplinary CDH clinic, diagnosis of asthma was based on the validated asthma screening questionnaire (22,23,26) consisting of seven questions in English or Spanish, administered by a pulmonologist via a phone interview after verbal consent. A positive score on the asthma screening questionnaire was based on a previously described complete algorithm termed Models A to G, involving various combinations of four or five question elements (22), and a three-question abbreviated algorithm (23) with a “yes” to question 1 (asthma) or positive responses to both questions 4 (exercise-related respiratory symptoms) and question 6 (daytime respiratory symptoms). A positive score on the abbreviated algorithm is particularly sensitive and specific in detecting subjects with the highest risk for persistent asthma, based on the National Institutes of Health Guidelines for the Evaluation and Treatment of asthma (27).
Impulse oscillometry
IOS is one type of forced oscillation technique that delivers a spectrum of frequencies in an impulse on the airway during tidal breathing. This determines lung function by measuring the mechanical properties of the lung. The sound waves are transmitted along the bronchial tree by oscillating sound signals of various frequencies, typically 5 and 20 Hz. IOS provides a measure of the total airway resistance [resistance at 5 Hz (R5)], the proximal airway resistance [resistance at 20 Hz (R20)], and the peripheral airway resistance (R5-R20). Reactance at 5 Hz (X5) relates to the physical properties of the lung parenchyma and its ability to expand and facilitate alveolar filling. Frequency response (Fres) is the point at which reactance is zero (when forces of inertia and capacitance are equal). The reactance area is the sum of all the frequency values from X5 to the Fres frequency, that is, it quantifies the respiratory reactance between 5 Hz and Fres. Patients with asthma have increased R5Hz and Fres, while the X5Hz is more negative (28,29).
IOS system (Jaeger MasterSuite, CareFusion, Hoechberg, Germany) was calibrated as per the manufacturer’s recommendations. Testing and analysis were performed in accordance with European respiratory society/American thoracic society guidelines using existing reference values (30,31). Testing was performed with the patient sitting and breathing at tidal volume, the head held in a neutral position, a nose clip in place, legs uncrossed, and the cheeks firmly supported by either the patient or another individual such as the examiner or caregiver.
IOS measurements were compared between all CDH patients (including HR and LR combined) and a CDH reference group. The measurements were also compared between the HR and LR patients, and their respective HR and LR reference group. The reference group consisted of predicted values of healthy children of the same age, height, race, and gender.
Spirometry
Spirometry (Jaeger Master Screen, CareFusion, Hoechberg, Germany) was performed in the clinic, following American Thoracic Society (ATS)/European Respiratory Society guidelines (32). ATS predicted normative values expressed as percent predicted were based on global lung initiative 2012 values (33). Traces not meeting quality and reproducibility criteria were excluded. Spirometry results including FEV1/FVC <80% of predicted fixed lower limit of normal (LLN), concave shape of the flow-volume loop, flow-time curve, and FEF 25–75% were interpreted based on established guidelines. The obstructive airway pattern was defined as FEV1< LLN with FEV1/FVC < LLN (34).
Lung perfusion scan (V/Q scan)
V/Q scans were done as part of our defect size-based protocol implemented in our comprehensive multidisciplinary CDH clinic. V/Q scan for LR (defect size A/B) patients was done at 12 months and 3 years of age. HR (defect size C/D) patients had V/Q scans at 12 months and 3–5 years of age, depending on findings and clinical presentation. V/Q scans were performed to determine the split perfusion ratio on the CDH-affected side as part of routine clinical care. Perfusion scintigraphy was accomplished by microembolization with radiolabeled particles. 99mTc albumin aggregated (Tc-MAA) was injected into a peripheral vein of CDH survivors aged 12 months and older by a pediatric radiologist. Tc-MAA was injected slowly during 3–5 respiratory cycles with the patient in the supine position. Imaging was preferably performed in the upright position to increase chest cavity size and minimize diaphragmatic motion. Supine and decubitus images were obtained when upright images could not be obtained. A value of less than 30% perfusion ratio on the affected side was chosen as the threshold for severe perfusion compromise, based on previous literature (35).
Data analysis
Frequencies (with percentages) were used to describe the categorical variables. Descriptive statistics of the median and interquartile range were used for continuous variables. Mann–Whitney U test and Fisher’s exact test were used for continuous data as needed. All statistical testing was performed in Stata/IC v.13.1 (Stata Corp, College Station, TX, USA) and statistical significance was assumed at a two-sided alpha of 0.05.
Results
NICU outcomes among CDH survivors
One hundred and thirty-three total patients were discharged from the NICU and 102 (77%) of these patients were seen in the comprehensive multidisciplinary CDH clinic. There were no statistically significant differences in demographics (age, gender, and race), birth weight, or gestational age; however, baseline characteristics such as prenatal diagnosis, intrathoracic liver, laterality, o/e LHR, and surgical repair varied significantly between LR CDH [n=79, defect A: n=25/79 (32%), defect B: n=54/79 (68%)] and HR CDH [n=54, defect C: n=45/54 (83%), defect D: n=9/54 (17%)] (Table 1). The overall prevalence of pulmonary hypertension at discharge was 19% (n=25/133). HR CDH had a higher prevalence of pulmonary hypertension at discharge [HR: 16/54 (30%) vs. LR: 9/79 (12%), P=0.009]. Similarly, ECLS utilization [HR: 19/54 (35%) vs. LR: 4/79 (5%), P<0.001] was higher in HR CDH. HR CDH patients required a longer median period of ventilation compared with LR CDH [HR: 17 days (IQR: 12–27) vs. LR: 5 days (IQR: 2–9), P<0.001] (Table 2). The total length of NICU stay was also significantly higher in HR CDH [HR: 59 days (IQR: 31–91) vs. LR: 17 days (IQR: 12–31), P<0.001]. A significantly higher number of HR patients were discharged on oxygen [HR: 16/54 (30%) vs. LR: 2/79 (3%), P<0.001] (Table 2).
Table 1
Characteristics | All patients, n=133 | LR (defect type A/B), n=79 | HR (defect C/D), n=54 | P value |
---|---|---|---|---|
Age (months), median (IQR) | 91 (53–128) | 88 (54–123) | 97 (53–141) | 0.348 |
Male gender, n (%) | 66 (50) | 41 (52) | 25 (46) | 0.526 |
Race | ||||
Caucasian, n (%) | 59 (44) | 34 (43) | 25 (46) | 0.447 |
Hispanic, n (%) | 42 (32) | 24 (30) | 18 (33) | |
Black, n (%) | 15 (11) | 9 (11) | 6 (11) | |
Asian, n (%) | 8 (6) | 4 (5) | 4 (7) | |
Other, n (%) | 9 (7) | 8 (10) | 1 (2) | |
Birth weight (kg), median (IQR) | 2.98 (2.61–3.35) | 3.03 (2.69–3.40) | 2.94 (2.56–3.18) | 0.135 |
Gestational age (weeks), median (IQR) | 38 (37–39) | 38 (37–39) | 38 (37–39) | 0.440 |
Laterality of CDH | ||||
Left, n (%) | 108 (81) | 70 (88) | 38 (70) | 0.023* |
Right, n (%) | 24 (18) | 9 (12) | 15 (28) | |
Bilateral, n (%) | 1 (1) | 0 (0) | 1 (2) | |
Prenatal diagnosis, n (%) | 76 (57) | 39 (49) | 37 (69) | 0.028* |
Liver intrathoracic, n (%) | 44 (33) | 13 (17) | 31 (57) | <0.001* |
o/e LHR, median (IQR) | 41.6 (33.0–52.4) | 45.8 (36.5–53.4) | 39.3 (25.4–47.4) | 0.175 |
Surgical repair | ||||
Primary, n (%) | 48 (36) | 48 (61) | 0 (0) | <0.001* |
Patch, n (%) | 85 (64) | 31 (39) | 54 (100) |
*, P<0.05. CDH, congenital diaphragmatic hernia; LR, low-risk; HR, high-risk; IQR, interquartile range; o/e LHR, observed/expected lung head circumference ratio.
Table 2
Outcomes | All patients, n=133 | LR (defect type A/B), n=79 | HR (defect C/D), n=54 | P value |
---|---|---|---|---|
Pulmonary hypertension at discharge, n (%) | 25 (19) | 9 (12) | 16 (30) | 0.009* |
Receipt of ECLS, n (%) | 23 (18) | 4 (5) | 19 (35) | <0.001* |
Mechanical ventilation days, median (IQR) | 8.5 (4–17) | 5 (2–9) | 17 (12–27) | <0.001* |
Discharged on oxygen | ||||
Overall, n (%) | 18 (14) | 2 (3) | 16 (30) | <0.001* |
Vent, n (%) | 3 (2) | 0 (0) | 3 (6) | |
Nasal cannula, n (%) | 15 (11) | 2 (3) | 13 (24) | |
Room air, n (%) | 115 (87) | 77 (97) | 38 (70) | |
Length of NICU stay (days), median (IQR) | 28.5 (15–59) | 17 (12–31) | 59 (31–91) | <0.001* |
Age at discharge (weeks), median (IQR) | 4.8 (2.9–9.7) | 3.4 (2.0–6.7) | 8.4 (4.7–13.0) | <0.001* |
*, P<0.05. NICU, neonatal intensive care unit; CDH, congenital diaphragmatic hernia; LR, low-risk; HR, high-risk; ECLS, extracorporeal life support; IQR, interquartile range.
Outcomes among CDH survivors following NICU discharge
Pulmonary outcomes, such as asthma, pulmonary hypertension, and health care utilization were determined in our complete cohort of patients surviving NICU discharge (n=133) and who were seen in the comprehensive multidisciplinary CDH clinic (n=102). The prevalence of asthma in CDH patients was n=37/133 (28%) and it was significantly higher in the HR group [HR: 20/54 (37%) vs. LR: 17/79 (22%), P=0.05]. Only 4% (n=5/133) of CDH patients had CDH-associated pulmonary hypertension, and all these patients belonged to the HR group. There was a higher percentage of patients with rehospitalizations in the HR group (HR: 32/54 (59%) vs. LR: 33/79 (42%), P=0.019). Patients in the HR group had a significantly longer duration of hospital stay [HR: 9 (IQR: 2–27) vs. LR: 4 (IQR: 1–8), P=0.035]. Additionally, there was an increase in the average number of rehospitalizations in the HR group [HR: 1 (IQR: 0–2) vs. LR: 0 (IQR: 0–1), P=0.019]. There was no difference in the number of ER visits between the HR and LR groups [HR: 1 (IQR: 0–3) vs. LR: 0.5 (IQR: 0–2), P=0.245] (Table 3).
Table 3
Outcomes | All patients, n=133 | LR (defect type A/B), n=79 | HR (defect C/D), n=54 | P value |
---|---|---|---|---|
Asthma, n (%) | 37 (28) | 17 (22) | 20 (37) | 0.050* |
CDH associated pulmonary hypertension, n (%) | 5 (4) | 0 (0) | 5 (9) | 0.006* |
Patients that had rehospitalizations, n (%) | 65 (49) | 33 (42) | 32 (59) | 0.050* |
Number of rehospitalizations, median (IQR) | 1 (0–1) | 0 (0–1) | 1 (0–2) | 0.019* |
Average days of rehospitalization, median (IQR) | 5 (2–15) | 4 (1–8) | 9 (2–27) | 0.035* |
Patients that had ER visits, n (%) | 66 (50) | 37 (47) | 29 (54) | 0.472 |
Number of ER visits, median (IQR) | 1 (0–3) | 0.5 (0–2) | 1 (0–3) | 0.245 |
*, P<0.05. CDH, congenital diaphragmatic hernia; NICU, neonatal intensive care unit; LR, low-risk; HR, high-risk; IQR, interquartile range; ER, emergency room.
Outcomes among CDH survivors at comprehensive multidisciplinary CDH clinic
Of the 133 CDH patients, a total of 102 patients were followed at the comprehensive multidisciplinary CDH clinic. Of those, 42% were HR (n=43/102) and 58% were LR (n=59/102). A lung perfusion scan was performed in 38% (n=39/102) of CDH patients. Perfusion ratio on the ipsilateral side was significantly lower in the HR CDH group [HR: 29 (IQR: 20–33) vs. LR: 38 (IQR: 34–42), P<0.001] (Figure 2). A perfusion ratio of less than 30% on the CDH ipsilateral side was seen in 13% (n=5/39) of patients with a V/Q scan; all 5 patients belonged to the HR group. All HR CDH patients with a perfusion ratio of less than 30% developed asthma [HR: 5/13 (38%), (P=0.002)].
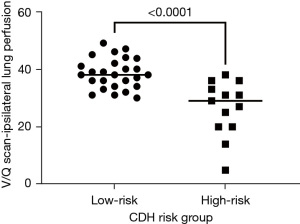
Pulmonary function tests
Spirometry
A total of 17 patients underwent spirometry [forced vital capacity (FVC), forced expiratory volume in one second (FEV1), and FEV1/FVC] at their baseline (waiting at least 4 weeks after a pulmonary infection). The median values were FVC 92% predicted (1.6 L), FEV1 86% predicted (1.3 L), FEV1/FVC 92% predicted (86%), and FEF 25–75% was 66% predicted (1.5 L). Of these, 3 patients (17%) had an obstructive pattern; 3 patients (18%) had a restrictive pattern, and 11 patients (65%) had normal spirometry. There was no significant difference in expiratory flows between HR and LR CDH patients. The FVC median was 88.8% predicted (IQR: 85–117) in the HR group vs. 87% predicted (IQR: 79.5–99.5) in the LR group (P=0.441). The FEV1 median was 79% predicted (IQR: 67.4–102) in the HR group vs. 89% predicted (IQR: 77–94) in the LR group (P=0.885). The FEV1/FVC median was 85% (IQR: 79–96) in the HR group vs. 96.5% (92.5–103) in the LR group (P=0.111). The FEF 25–75% median was 58% predicted (IQR: 38–70) in the HR group vs. 74.5% predicted (IQR: 63–93.5) in the LR group (P=0.149).
Impulse oscillometry
All IOS participants
IOS was performed in 24 patients measuring resistance at 5Hz (R5Hz), reactance at 5Hz (X5Hz), and resonant frequency (Fres). The median R5Hz for the CDH group was 10.37 (IQR: 8.6–12.2) kPa/(L/s) vs. 11.62 (IQR: 8.2–13.4) kPa/(L/s) in the reference group (P=0.07); the median X5Hz for CDH group was −3.57 [IQR: (−3.9) to (−2.63)] kPa/(L/s) vs. −2.84 [IQR: (−3.6) to (−1.095)] kPa/(L/s) in the reference group (P=0.02); the median Fres was 18.19 (IQR: 17.1–20.4) vs. 25.15 (IQR: 21.1–29.1) L/s in the reference group (P=0.001).
LR Group
The resistance, reactance, and resonant frequency were similar compared to the reference group. Specifically, the median R5Hz was 10.72 kPa/(L/s) in LR CDH vs. 11.3 kPa/(L/s) in LR reference (P=0.134); the median X5Hz was −2.23 kPa/(L/s) in LR CDH vs. −3.62 kPa/(L/s) in LR reference (P=0.012); the median Fres was 23.57 L/s in LR CDH vs. 18.29 L/s in LR reference (P=0.026) (Figure 3).
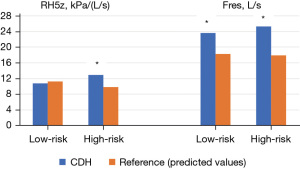
HR group
The resistance and Fres were increased in the CDH group compared to the reference group. Specifically, the median R5Hz was 12.95 kPa/(L/s) in HR CDH vs. 9.8 kPa/(L/s) in the HR reference group (P=0.010), and the median Fres was 25.34 L/s in HR CDH vs. 17.86 L/s in the HR reference group (P=0.004) (Figure 3). However, the reactance was similar in both groups. The median X5Hz was −3.16 kPa/(L/s) for the HR CDH vs. −3.15 kPa/(L/s) in the HR reference group (P=0.735).
HR vs. LR CDH groups
The R5Hz median was 12.95 (IQR: 7.59–11.7) in the HR group vs. 10.72 (IQR: 9.9–12.74) in the LR group (P=0.111). The X5Hz median was −3.16 [IQR: (−3.97) to (−2.42)] in the HR group vs. −2.23 [IQR: (−4.07) to (−3.11)] in the LR group (P=0.385). The Fres median was 25.34 (IQR: 16.27–19.51) in the HR group vs. 23.57 [IQR: 17.56 to (−23.13)] in the LR group (P=0.622). HR CDH patients had an increased prevalence of asthma by IOS measurements compared with LR CDH patients (8 vs. 2, P=0.038), but spirometry did not detect this difference (LR 0 vs. HR 2 patients, P=0.471).
Discussion
In this single-center study, we demonstrated that infants with HR CDH (CDHSG defects: C/D) have a higher likelihood of experiencing significant morbidities such as asthma, pulmonary hypertension, need for ECLS, and prolonged ventilator dependency in NICU compared to those with LR CDH (CDHSG defects: A/B). These findings are consistent with previous literature (21,36,37). Specifically, there was a twofold increase in the risk of asthma and pulmonary hypertension at discharge in HR CDH. Our study highlights the usefulness of IOS in monitoring lung function in CDH patients, revealing a higher prevalence of asthma in HR CDH when compared to spirometry.
Approximately 75% of CDH patients experience a post-discharge pulmonary morbidity (13) including asthma, and pulmonary hypertension, resulting from pulmonary hypoplasia, ventilator therapy, diaphragm dysfunction, and mechanical changes in the chest wall and spine (38). Previous literature has shown impaired lung function in CDH patients (39-42), with reported asthma prevalence ranging from 23.6% to 30%, which aligns with our study’s finding of 28% prevalence (43-45). The process of bronchioles and blood vessels branching impediment during the embryonic phase can result in acinar hypoplasia (46,47). Pulmonary hypoplasia and prolonged ventilator support in early life could set the stage for structural and functional pulmonary vascular alterations, resulting in synaptic airway growth leading to asthma and obstructive ventilatory impairment (48,49).
While spirometry has traditionally been employed to identify ventilatory impairment in CDH patients (40,44), IOS is now being utilized to study several disease states, including asthma, chronic pulmonary obstructive disease, and interstitial lung diseases. Although most of the studies have been done in children with asthma and are focused on monitoring disease progress and elucidating the effect of bronchodilators, research on IOS implementation in CDH remains limited. When compared to baseline measurements, a 20% decrease in FEV is equivalent to a 50% decrease in X5Hz, and this has demonstrated increased sensitivity for identifying bronchial hyperreactivity compared to spirometry; a feature of asthma (50). Schulze et al. revealed that even at lower doses of methacholine, there were significant changes in IOS parameters compared to baseline, reflecting the sensitivity of the procedure. This allows bronchoprovocation tests to be performed with smaller doses of bronchoconstriction agents (51). Shi et al. compared baseline spirometry and IOS parameters between children with well-controlled asthma and those subsequently presenting with acute exacerbations of asthma (52). Spirometry only detected small differences in the FEV1/forced vital capacity (FVC) ratio between the groups and did not reach statistical significance. However, IOS parameters, including R5Hz, and the frequency dependence of resistance (R5–R20), were significantly different between the two groups (52). Similarly, Gonem et al. showed that entropy of impedance measured over time can differentiate the patients with more frequent exacerbations compared to those with less frequent exacerbations (53).
Severe CDH with large defect sizes represents a population at risk for worsening lung function at an early age (41). In the HR CDH population, IOS measures of R5Hz and Fres were notably higher compared to predicted values of healthy children. A higher proportion of patients in the HR group were diagnosed with asthma based on IOS compared to spirometry. It has been shown that average pulmonary function declines with age relative to the expected population norm. This reflects an arrest of pulmonary parenchymal growth versus evolving emphysema, which predisposes these patients to the future development of obstructive lung disease (54). The increased prevalence of asthma in HR CDH detected by IOS compared to spirometry can be attributed to the ability of IOS to capture subtle changes in lung function by measuring airway resistance and reactance in the central and peripheral airways during tidal breathing. This enables the identification of obstructive changes and declines in asthma control prior to the spirometry (52). This finding may be associated with the limited effectiveness of spirometry in the younger patient population, making tidal breathing techniques an acceptable alternative option (55-57).
An early embryogenic alteration affects the development of pulmonary vasculature, lung parenchyma, and diaphragm. Pulmonary hypertension in CDH survivors may be caused by a combination of factors, including external compression from herniated intra-abdominal contents, persistent poorly perfused ventilated areas representing dead space, and subsequent increased intrapulmonary shunting and hypoxemia, especially in large-size defects (58). The overall prevalence of pulmonary hypertension in our cohort at discharge was 19% with a higher percentage in the HR group. The prevalence of CDH-associated pulmonary hypertension was only 4%. This finding supports existing data that the tendency to develop pulmonary hypertension decreases with time (59). However, previous data reports that the time it takes for these changes to occur is longer because of the current limitations of invasive support measures, like ECLS (59). The prevalence of pulmonary hypertension has been reported between 11–17% for patients on all kinds of treatment including intravenous and oral medications; other studies have reported a range of 4.5–38% (60-62). Despite our cohort consisting of medically complex patients from a comprehensive multidisciplinary CDH clinic, the prevalence of pulmonary hypertension falls within the range reported in previous studies. This likely reflects the effectiveness of our comprehensive pulmonary management approach, which includes round-the-clock access to healthcare providers, integrated within our comprehensive multidisciplinary CDH clinic.
It is well-accepted that capillary growth and alveolar growth are intricately connected. Previous work has shown a worse V/Q ratio based on ECLS requirements and patch repair (larger defect) correlating the severity of defect size. This supports our findings of low perfusion on the affected side in the HR group (35). The requirement for ECLS and patch repair was higher in our HR CDH group. These perinatal variables like patch repair could be surrogates marker for a greater degree of pulmonary hypoplasia present at birth (63). Interestingly in our study, a perfusion ratio of less than 30% on the affected side was associated with asthma specifically in the HR group. These findings could be interpreted as either a greater degree of shunt across distal airways resulting in reduced ventilation, or inherent structural defects in pulmonary vasculature in large-size defects related to pulmonary hypoplasia. It is possible that a V/Q scan serves as an adjunct to a pulmonary function test. This close relationship between asthma and perfusion can help identify a subset of patients at risk for poor long-term functional outcomes.
Fetal lung volume measurement by magnetic resonance imaging (MRI) is a potential predictor of pulmonary hypoplasia (64). Perrone et al. demonstrated that prenatal ultrasound and MRI measurements of lung volume correlate with postnatal outcomes, including survival, ECLS use, defect size, and liver position (19,65). However, it has also been shown that radiological methods to assess the degree of pulmonary hypoplasia are not that reliable (66). Data on predictors for outcomes in the CDH population such as birth weight, Apgar scores, associated anomalies, presence of moderate-to-severe CDH-related pulmonary hypertension, need for higher ventilatory settings, ECLS, and shock is not disease-specific (67-70). The size of the diaphragm defect is disease-specific and correlates with morbidity in liveborn infants with CDH. Furthermore, animal models suggest that a large defect is associated with much smaller lungs (36). Based on our findings we posit that the size of the defect in CDH has a direct impact on the severity of pulmonary hypoplasia. This, in turn, contributes to worse outcomes, as evidenced by adverse events in HR CDH patients.
The current study is subject to limitations typically associated with single-center studies including lower volume, potential care evolution over the time examined, and the potential for non-universal generalizability. However, it is important to note that selection bias was unlikely given the no significant difference in demographic features between HR and LR groups. Furthermore, similarity in the distribution of the CDH population by defect size compared to previous literature add to the generalizability of our findings (37). Further, we did not have non-CDH controls to estimate the prevalence of asthma, hence we used published data on the prevalence of asthma in the general pediatric population (8% of children 0–17 years of age) (71,72) compared to 28% which we identified in the CDH group. The number of CDH survivors who underwent objective assessment for asthma via both spirometry and IOS was limited due to age and technical challenges associated with performing these maneuvers. Moreover, collection during the pandemic was limited for a myriad of reasons. Finally, our comprehensive multidisciplinary CDH clinic was launched in 2016, limiting follow-up for patients discharged 2012–2015.
Conclusions
This study reinforces existing literature that patients with large defect size are at increased risk for long-term pulmonary morbidities. These data provide valuable insight for risk-stratified pulmonary follow-up in CDH survivors and are helpful in developing screening tools, protocolized management, and guidance for families regarding outcomes in CDH-related long-term morbidities in accordance with diaphragm defect size. In addition, our study reports the application of IOS in the CDH patient population underscoring the importance of early detection and monitoring of lung disease, particularly in the HR CDH group.
Acknowledgments
The authors would like to thank the Ladybug Foundation for their funding, which helped to support this study.
Funding: This article was supported in part by
Footnote
Provenance and Peer Review: This article was commissioned by the Guest Editor (Antonio F. Corno) for the series “The Impact of the Progresses of Knowledge and Technologies in Pediatrics” published in Translational Pediatrics. The article has undergone external peer review.
Reporting Checklist: The authors have completed the STROBE reporting checklist. Available at https://tp.amegroups.com/article/view/10.21037/tp-23-14/rc
Data Sharing Statement: Available at https://tp.amegroups.com/article/view/10.21037/tp-23-14/dss
Peer Review File: Available at https://tp.amegroups.com/article/view/10.21037/tp-23-14/prf
Conflicts of Interest: All authors have completed the ICMJE uniform disclosure form (available at https://tp.amegroups.com/article/view/10.21037/tp-23-14/coif). The series “The Impact of the Progresses of Knowledge and Technologies in Pediatrics” was commissioned by the editorial office without any funding or sponsorship. CKJ serves as the Vice President for the Texas Society of Sleep Professionals (non for profit). The authors have no other conflicts of interest to declare.
Ethical Statement: The authors are accountable for all aspects of the work in ensuring that questions related to the accuracy or integrity of any part of the work are appropriately investigated and resolved. The study was conducted in accordance with the Declaration of Helsinki (as revised in 2013). The study was approved by the ethics committee of The University of Texas Health Science Center at Houston of Institutional Review Board approval (No. HCS-MS-18-1036) and individual consent for this retrospective analysis was waived.
Open Access Statement: This is an Open Access article distributed in accordance with the Creative Commons Attribution-NonCommercial-NoDerivs 4.0 International License (CC BY-NC-ND 4.0), which permits the non-commercial replication and distribution of the article with the strict proviso that no changes or edits are made and the original work is properly cited (including links to both the formal publication through the relevant DOI and the license). See: https://creativecommons.org/licenses/by-nc-nd/4.0/.
References
- Harting MT, Lally KP. The Congenital Diaphragmatic Hernia Study Group registry update. Semin Fetal Neonatal Med 2014;19:370-5. [Crossref] [PubMed]
- Keijzer R, Liu J, Deimling J, et al. Dual-hit hypothesis explains pulmonary hypoplasia in the nitrofen model of congenital diaphragmatic hernia. Am J Pathol 2000;156:1299-306. [Crossref] [PubMed]
- Zani A, Chung WK, Deprest J, et al. Congenital diaphragmatic hernia. Nat Rev Dis Primers 2022;8:37. [Crossref] [PubMed]
- Guner Y, Jancelewicz T, Di Nardo M, et al. Management of Congenital Diaphragmatic Hernia Treated With Extracorporeal Life Support: Interim Guidelines Consensus Statement From the Extracorporeal Life Support Organization. ASAIO J 2021;67:113-20. [Crossref] [PubMed]
- Zalla JM, Stoddard GJ, Yoder BA. Improved mortality rate for congenital diaphragmatic hernia in the modern era of management: 15 year experience in a single institution. J Pediatr Surg 2015;50:524-7. [Crossref] [PubMed]
- Gupta VS, Harting MT, Lally PA, et al. Mortality in Congenital Diaphragmatic Hernia: A Multicenter Registry Study of Over 5000 Patients Over 25 Years. Ann Surg 2023;277:520-7. [Crossref] [PubMed]
- Weber TR, Kountzman B, Dillon PA, et al. Improved survival in congenital diaphragmatic hernia with evolving therapeutic strategies. Arch Surg 1998;133:498-502; discussion 502-3. [Crossref] [PubMed]
- Okuyama H, Kubota A, Oue T, et al. Inhaled nitric oxide with early surgery improves the outcome of antenatally diagnosed congenital diaphragmatic hernia. J Pediatr Surg 2002;37:1188-90. [Crossref] [PubMed]
- Downard CD, Jaksic T, Garza JJ, et al. Analysis of an improved survival rate for congenital diaphragmatic hernia. J Pediatr Surg 2003;38:729-32. [Crossref] [PubMed]
- Javid PJ, Jaksic T, Skarsgard ED, et al. Survival rate in congenital diaphragmatic hernia: the experience of the Canadian Neonatal Network. J Pediatr Surg 2004;39:657-60. [Crossref] [PubMed]
- Lund DP, Mitchell J, Kharasch V, et al. Congenital diaphragmatic hernia: the hidden morbidity. J Pediatr Surg 1994;29:258-62; discussion 262-4. [Crossref] [PubMed]
- Coughlin MA, Werner NL, Gajarski R, et al. Prenatally diagnosed severe CDH: mortality and morbidity remain high. J Pediatr Surg 2016;51:1091-5. [Crossref] [PubMed]
- Putnam LR, Harting MT, Tsao K, et al. Congenital Diaphragmatic Hernia Defect Size and Infant Morbidity at Discharge. Pediatrics 2016;138:e20162043. [Crossref] [PubMed]
- American Academy of Pediatrics Section on Surgery. Lally KP, Engle W. Postdischarge follow-up of infants with congenital diaphragmatic hernia. Pediatrics 2008;121:627-32. [Crossref] [PubMed]
- Muratore CS, Kharasch V, Lund DP, et al. Pulmonary morbidity in 100 survivors of congenital diaphragmatic hernia monitored in a multidisciplinary clinic. J Pediatr Surg 2001;36:133-40. [Crossref] [PubMed]
- Wright T, Filbrun A, Bryner B, et al. Predictors of early lung function in patients with congenital diaphragmatic hernia. J Pediatr Surg 2014;49:882-5. [Crossref] [PubMed]
- Bisgaard H, Klug B. Lung function measurement in awake young children. Eur Respir J 1995;8:2067-75. [Crossref] [PubMed]
- Cioci AC, Urrechaga EM, Parreco J, et al. One-year outcomes of congenital diaphragmatic hernia repair: Factors associated with recurrence and complications. J Pediatr Surg 2021;56:1542-6. [Crossref] [PubMed]
- Perrone EE, Karmakar M, Lally PA, et al. Image-based prenatal predictors correlate with postnatal survival, extracorporeal life support use, and defect size in left congenital diaphragmatic hernia. J Perinatol 2022;42:1195-201. [Crossref] [PubMed]
- Jancelewicz T, Brindle ME. Prediction tools in congenital diaphragmatic hernia. Semin Perinatol 2020;44:151165. [Crossref] [PubMed]
- Lally KP, Lasky RE, Lally PA, et al. Standardized reporting for congenital diaphragmatic hernia--an international consensus. J Pediatr Surg 2013;48:2408-15. [Crossref] [PubMed]
- Jones CA, Morphew T, Clement LT, et al. A school-based case identification process for identifying inner city children with asthma: the Breathmobile program. Chest 2004;125:924-34. [Crossref] [PubMed]
- Galant SP, Crawford LJ, Morphew T, et al. Predictive value of a cross-cultural asthma case-detection tool in an elementary school population. Pediatrics 2004;114:e307-16. [Crossref] [PubMed]
- Al-Moamary MS, Alhaider SA, Alangari AA, et al. The Saudi Initiative for Asthma - 2021 Update: Guidelines for the diagnosis and management of asthma in adults and children. Ann Thorac Med 2021;16:4-56. [Crossref] [PubMed]
- Chawes B, Elenius V. Pulmonary function testing for the diagnosis of asthma in preschool children. Curr Opin Allergy Clin Immunol 2022;22:101-6. [Crossref] [PubMed]
- Chan KH, Stark JM, Mosquera RA, et al. Screening for asthma in preschool children with sickle cell disease. J Asthma 2023;60:1787-92.
- Expert Panel Working Group of the National Heart, Lung, and Blood Institute (NHLBI) administered and coordinated National Asthma Education and Prevention Program Coordinating Committee (NAEPPCC); Cloutier MM, Baptist AP, et al. 2020 Focused Updates to the Asthma Management Guidelines: A Report from the National Asthma Education and Prevention Program Coordinating Committee Expert Panel Working Group. J Allergy Clin Immunol 2020;146:1217-70.
- Nowowiejska B, Tomalak W, Radliński J, et al. Transient reference values for impulse oscillometry for children aged 3-18 years. Pediatr Pulmonol 2008;43:1193-7.
- Qi GS, Zhou ZC, Gu WC, et al. Detection of the airway obstruction stage in asthma using impulse oscillometry system. J Asthma 2013;50:45-51. [Crossref] [PubMed]
- Beydon N, Davis SD, Lombardi E, et al. An official American Thoracic Society/European Respiratory Society statement: pulmonary function testing in preschool children. Am J Respir Crit Care Med 2007;175:1304-45. [Crossref] [PubMed]
- Dencker M, Malmberg LP, Valind S, et al. Reference values for respiratory system impedance by using impulse oscillometry in children aged 2-11 years. Clin Physiol Funct Imaging 2006;26:247-50. [Crossref] [PubMed]
- Graham BL, Steenbruggen I, Miller MR, et al. Standardization of Spirometry 2019 Update. An Official American Thoracic Society and European Respiratory Society Technical Statement. Am J Respir Crit Care Med 2019;200:e70-88. [Crossref] [PubMed]
- Quanjer PH, Stanojevic S, Cole TJ, et al. Multi-ethnic reference values for spirometry for the 3-95-yr age range: the global lung function 2012 equations. Eur Respir J 2012;40:1324-43. [Crossref] [PubMed]
- Pellegrino R, Viegi G, Brusasco V, et al. Interpretative strategies for lung function tests. Eur Respir J 2005;26:948-68.
- Dao DT, Kamran A, Wilson JM, et al. Longitudinal Analysis of Ventilation Perfusion Mismatch in Congenital Diaphragmatic Hernia Survivors. J Pediatr 2020;219:160-166.e2. [Crossref] [PubMed]
- Lally KP, Lally PA, et al. Defect size determines survival in infants with congenital diaphragmatic hernia. Pediatrics 2007;120:e651-7.
- Chock VY, Danzer E, Chung S, et al. In-Hospital Morbidities for Neonates with Congenital Diaphragmatic Hernia: The Impact of Defect Size and Laterality. J Pediatr 2022;240:94-101.e6. [Crossref] [PubMed]
- Tan JK, Banton G, Minutillo C, et al. Long-term medical and psychosocial outcomes in congenital diaphragmatic hernia survivors. Arch Dis Child 2019;104:761-7. [Crossref] [PubMed]
- Peetsold MG, Heij HA, Nagelkerke AF, et al. Pulmonary function and exercise capacity in survivors of congenital diaphragmatic hernia. Eur Respir J 2009;34:1140-7. [Crossref] [PubMed]
- Toussaint-Duyster LCC, van der Cammen-van Zijp MHM, Spoel M, et al. Lung function in school-aged congenital diaphragmatic hernia patients; a longitudinal evaluation. Pediatr Pulmonol 2019;54:1257-66. [Crossref] [PubMed]
- Dao DT, Hayden LP, Buchmiller TL, et al. Longitudinal Analysis of Pulmonary Function in Survivors of Congenital Diaphragmatic Hernia. J Pediatr 2020;216:158-164.e2.
- Marven SS, Smith CM, Claxton D, et al. Pulmonary function, exercise performance, and growth in survivors of congenital diaphragmatic hernia. Arch Dis Child 1998;78:137-42. [Crossref] [PubMed]
- Gerall CD, Stewart LA, Price J, et al. Long-term outcomes of congenital diaphragmatic hernia: A single institution experience. J Pediatr Surg 2022;57:563-9. [Crossref] [PubMed]
- Spoel M, van der Cammen-van Zijp MH, Hop WC, et al. Lung function in young adults with congenital diaphragmatic hernia; a longitudinal evaluation. Pediatr Pulmonol 2013;48:130-7. [Crossref] [PubMed]
- El Chehadeh K, Becmeur F, Weiss L. Medium and long-term respiratory outcome in patients operated from congenital diaphragmatic hernia: From a series of 56 patients. Rev Pneumol Clin 2018;74:467-82. [Crossref] [PubMed]
- Mechanisms and limits of induced postnatal lung growth. Am J Respir Crit Care Med 2004;170:319-43. [Crossref] [PubMed]
- George DK, Cooney TP, Chiu BK, et al. Hypoplasia and immaturity of the terminal lung unit (acinus) in congenital diaphragmatic hernia. Am Rev Respir Dis 1987;136:947-50. [Crossref] [PubMed]
- Moschino L, Bonadies L, Baraldi E. Lung growth and pulmonary function after prematurity and bronchopulmonary dysplasia. Pediatr Pulmonol 2021;56:3499-508. [Crossref] [PubMed]
- Kennedy JD. Lung function outcome in children of premature birth. J Paediatr Child Health 1999;35:516-21. [Crossref] [PubMed]
- Bailly C, Crenesse D, Albertini M. Evaluation of impulse oscillometry during bronchial challenge testing in children. Pediatr Pulmonol 2011;46:1209-14. [Crossref] [PubMed]
- Schulze J, Smith HJ, Fuchs J, et al. Methacholine challenge in young children as evaluated by spirometry and impulse oscillometry. Respir Med 2012;106:627-34.
- Shi Y, Aledia AS, Galant SP, et al. Peripheral airway impairment measured by oscillometry predicts loss of asthma control in children. J Allergy Clin Immunol 2013;131:718-23. [Crossref] [PubMed]
- Gonem S, Umar I, Burke D, et al. Airway impedance entropy and exacerbations in severe asthma. Eur Respir J 2012;40:1156-63. [Crossref] [PubMed]
- Panitch HB, Weiner DJ, Feng R, et al. Lung function over the first 3 years of life in children with congenital diaphragmatic hernia. Pediatr Pulmonol 2015;50:896-907. [Crossref] [PubMed]
- Escobar H, Carver TW Jr. Pulmonary function testing in young children. Curr Allergy Asthma Rep 2011;11:473-81. [Crossref] [PubMed]
- Komarow HD, Skinner J, Young M, et al. A study of the use of impulse oscillometry in the evaluation of children with asthma: analysis of lung parameters, order effect, and utility compared with spirometry. Pediatr Pulmonol 2012;47:18-26. [Crossref] [PubMed]
- Frei J, Jutla J, Kramer G, et al. Impulse oscillometry: reference values in children 100 to 150 cm in height and 3 to 10 years of age. Chest 2005;128:1266-73. [Crossref] [PubMed]
- Gupta VS, Harting MT. Congenital diaphragmatic hernia-associated pulmonary hypertension. Semin Perinatol 2020;44:151167. [Crossref] [PubMed]
- Beals DA, Schloo BL, Vacanti JP, et al. Pulmonary growth and remodeling in infants with high-risk congenital diaphragmatic hernia. J Pediatr Surg 1992;27:997-1001; discussion 1001-2. [Crossref] [PubMed]
- Bhombal S, Patel N. Diagnosis & management of pulmonary hypertension in congenital diaphragmatic hernia. Semin Fetal Neonatal Med 2022;27:101383. [Crossref] [PubMed]
- Durward A, Macrae D. Long term outcome of babies with pulmonary hypertension. Semin Fetal Neonatal Med 2022;27:101384. [Crossref] [PubMed]
- Lewis L, Sinha I, Kang SL, et al. Long term outcomes in CDH: Cardiopulmonary outcomes and health related quality of life. J Pediatr Surg 2022;57:501-9. [Crossref] [PubMed]
- Hayward MJ, Kharasch V, Sheils C, et al. Predicting inadequate long-term lung development in children with congenital diaphragmatic hernia: an analysis of longitudinal changes in ventilation and perfusion. J Pediatr Surg 2007;42:112-6. [Crossref] [PubMed]
- Mahieu-Caputo D, Sonigo P, Dommergues M, et al. Fetal lung volume measurement by magnetic resonance imaging in congenital diaphragmatic hernia. BJOG 2001;108:863-8. [Crossref] [PubMed]
- Perrone EE, Abbasi N, Cortes MS, et al. Prenatal assessment of congenital diaphragmatic hernia at north american fetal therapy network centers: A continued plea for standardization. Prenat Diagn 2021;41:200-6. [Crossref] [PubMed]
- Holt PD, Arkovitz MS, Berdon WE, et al. Newborns with diaphragmatic hernia: initial chest radiography does not have a role in predicting clinical outcome. Pediatr Radiol 2004;34:462-4. [Crossref] [PubMed]
- Chaudhary J, Shivprasad B, Lakshmi V, et al. Analysis of Prognostic Factors in Congenital Diaphragmatic Hernia in Neonates. J Indian Assoc Pediatr Surg 2019;24:176-9. [Crossref] [PubMed]
- Skari H, Bjornland K, Frenckner B, et al. Congenital diaphragmatic hernia in Scandinavia from 1995 to 1998: Predictors of mortality. J Pediatr Surg 2002;37:1269-75. [Crossref] [PubMed]
- Boix-Ochoa J, Peguero G, Seijo G, et al. Acid-base balance and blood gases in prognosis and therapy of congenital diaphragmatic hernia. J Pediatr Surg 1974;9:49-57. [Crossref] [PubMed]
- Skari H, Bjornland K, Haugen G, et al. Congenital diaphragmatic hernia: a meta-analysis of mortality factors. J Pediatr Surg 2000;35:1187-97. [Crossref] [PubMed]
- Pearce N, Aït-Khaled N, Beasley R, et al. Worldwide trends in the prevalence of asthma symptoms: phase III of the International Study of Asthma and Allergies in Childhood (ISAAC). Thorax 2007;62:758-66. [Crossref] [PubMed]
- Zahran HS, Bailey CM, Damon SA, et al. Vital Signs: Asthma in Children — United States, 2001–2016. 2018.