Outcomes of percutaneous transluminal renal angioplasty for pediatric renovascular hypertension: a 12-year retrospective single-center experience
Highlight box
Key findings
• PTRA for the treatment of pediatric RVH was associated with improved blood pressure control and long-term preservation of renal function, as reflected by an increase in affected kidney size and a higher GFR.
What is known and what is new?
• There are numerous pediatric case reports and series supporting the benefit of PTRA in BP control in children with RVH. However, the impact of PTRA on the affected kidney size and GFR remains to be investigated and is poorly understood.
• The increased length and GFR of the affected kidneys after PTRA predict the recovery of parenchyma and the long-term preservation of renal function.
What is the implication, and what should change now?
• Long-lasting hypoperfusion may lead to severe and irreversible renal atrophy, even in RVH children who are having well-controlled of the illness by antihypertensive drugs. Prompt revascularization is essential to protect long-term renal function.
Introduction
Renovascular disease underlies 5–10% of all childhood hypertension (1). Oral antihypertensives are the first-line therapy for renovascular hypertension (RVH); however, pediatric patients typically present with remarkably elevated blood pressure (BP), which is poorly controlled by medication. Endovascular therapy may be attempted when BP control is suboptimal or pharmacological adverse effects are significant. There are numerous pediatric case reports and series on the use of percutaneous transluminal renal angioplasty (PTRA), with a growing number supporting the benefit of PTRA in BP control in children with RVH (2-19). However, the impact of PTRA on the affected kidney size and glomerular filtration rate (GFR) remains to be investigated and is poorly understood (14,18). The present study retrospectively analyzed data collected over the course of 12 years to understand the outcomes of 37 children with RVH treated with PTRA. The purpose of this study was to evaluate the effect of PTRA on BP control and the preservation of renal function as reflected by the change in GFR and kidney size. We present this article in accordance with the STROBE reporting checklist (available at https://tp.amegroups.com/article/view/10.21037/tp-23-215/rc).
Methods
Patients
All children at our center with a presumptive diagnosis of RVH and poor BP control despite taking antihypertensive medication were referred for non-invasive imaging, including duplex Doppler ultrasonography, computed tomography, and magnetic resonance imaging. The inclusion criteria for PTRA were (I) patients with stenosis ≥60% on digital subtraction angiography (DSA) (1,19); (II) patients with poor BP control despite antihypertensive medication; and (III) patients with a condition tolerant to PTRA. The exclusion criteria were (I) diffuse stenosis of renal artery; and (II) patients with active-stage Takayasu arteritis (TA) with an elevated erythrocyte sedimentation rate and C-reactive protein levels. With approval from the Institutional Review Board of the Peking University First Hospital, the medical records of consecutive pediatric patients under 18 years old who underwent PTRA treatment for RVH at our center between January 2010 and January 2022 were retrospectively reviewed. Data on demographics, clinical presentation, etiology, BP measurements, antihypertensive medications, computed tomography images, and ultrasound and diethylenetriaminepentaacetic acid (DTPA) scans were collected.
Pediatric hypertension
BP was measured with an electronic sphygmomanometer after the patient had sat and rested for 5 minutes. All BP measurements were taken thrice on the right arm using a cuff fitted to arm circumference, and the average was taken. BP ratio (BPR), i.e., ratio of actual BP value to the 95th percentile value (based on sex, age, and height percentiles), was generated for systolic BP (SBP) and diastolic BP (DBP) to allow for more reliable BP comparisons among different age and sex groups (20). Pediatric hypertension was defined as the average clinic measured SBP ratio (SBPR) and/or DBP ratio (DBPR) ≥1. Sex hormone assays, renin assays, thyroid hormone assays, and other tests were completed during hospitalization.
Patients were assessed for the presence of target organ damage due to hypertension. Hypertensive encephalopathy was diagnosed when patients presented with a sharply elevated BPR and diffuse brain dysfunction, such as severe headache, vomiting, blurred vision, seizures, and coma, accompanied by papilledema (21). Cardiac parameters were measured by two-dimensional echocardiography performed by a pediatric cardiologist according to the American Society of Echocardiography Pediatric guidelines (22). Left ventricular mass > the 95th percentile height was defined as left ventricular hypertrophy (LVH) (23). A fundoscopy examination was performed to assess the presence of hypertensive retinopathy.
RVH etiology
The RVH etiologies were diagnosed as follows: diagnosis of TA was based on typical angiographic abnormalities, including occlusion, dilatation, or a thickened arterial wall, in addition to low-grade fever and an elevated erythrocyte sedimentation rate ≥20 mm/h or C-reactive protein levels, as per the acknowledged diagnostic criteria (24). Fibromuscular dysplasia (FMD) was presumptively diagnosed if the patient had a focal lesion in the renal artery with a “string of beads” angiographic appearance or no evidence of other genetic or vasculitis syndromes (25). Moyamoya disease (MMD) was diagnosed if cerebral angiography showed stenosis or occlusion at the terminal portion of the internal carotid artery, at the proximal portion of the anterior cerebral arteries, and/or the middle cerebral artery, as well as abnormalities in the vascular networks adjacent to the occlusive or stenotic lesions in the arterial phase (26). Diagnosis of neurofibromatosis type 1 (NF1) was based on clinical symptoms and molecular genetic testing (27).
PTRA procedure
A multidisciplinary team comprising pediatric nephrologists, pediatric rheumatoid immunologists, interventional radiologists, and vascular surgeons made management decisions for children with a suspected RVH diagnosis. For children under 10 years of age or with poor compliance, general anesthesia was chosen to ensure the safety of the procedures; otherwise, local anesthesia was used (28). Systemic heparinization (50 U/kg of heparin sodium injection, SH PHARMA, Shanghai, China) was performed for all procedures. Selective renal arteriography was performed after abdominal aortography. Lesion stenosis location was characterized as follows: ostial—involving the renal ostium and within 5 mm of the aortic origin; truncal—confined to the main renal artery; and branch—confined to branch vessels or with a portion of the stenosis extending into the divisional or segmental branches.
The number and sizes of balloons (NC Trek, Abbott, IL, USA; or Sterling, Boston Scientific, Marlborough, MA, USA) were chosen according to the adjacent renal artery without stenosis, as well as the dilatation outcome. Technical success was characterized as a residual stenosis ≤30% on angiography after first time PTRA. In patients with severe residual stenosis (>50%), which were considered as technically unsuccessful angioplasty, bailout stent selection was made at the surgeon’s discretion. Intravascular pressure measurements were not performed mainly due to the small size of the pediatric vasculature. Post-procedure angiography was performed to assess lesion length, dilatation response, and residual stenosis.
Postoperative care and follow-up
A daily dose of 50 U/kg of low-molecular-weight heparin (FRAXIPARINE, Aspe Notre-Dame-de-Bondeville, France) was injected subcutaneously for 3 days following angioplasty or stenting. After angioplasty, aspirin was prescribed for 6 months. When stenting was performed, the dual antiplatelet therapy of aspirin with clopidogrel was prescribed for 6 months, followed by lifelong aspirin. Angiotensin-converting enzyme inhibitors (ACEIs) and angiotensin receptor blockers (ARBs) were used according to indications. BP was measured at 3 time points: before PTRA, before discharge, and at last follow-up. BPR was calculated based on the patient’s BP, age, and height at the time. At 1, 3, 6, and 12 months after discharge, the children were scheduled for follow-up visits, which continued every 6 to 12 months thereafter. The follow-up included BP measurement, antihypertensive drugs, and assessment of kidney size and renal artery patency using ultrasound and of GFR via DTPA scan. To compare with natural physical development of kidneys in healthy children, the kidney length ratio (KLR) of age-based percentile pre and post intervention, which was presented as percentile value, was calculated (29).
Based on follow-up findings, clinical outcomes of the initial PTRA were defined as: cure—BP reduced to below the 95th percentile without antihypertensive drugs; improvement—SBP and DBP reduced by ≥15% of the pre-intervention level with the same or fewer medications; and failure—SBP and DBP reduced by <15% of the pre-intervention level or a worsening in BP level, as well as the occurrence of restenosis, necessitating additional interventional treatment (6). Clinical benefit included cure and improvement. Restenosis was defined as stenosis ≥60% on DSA at follow-up. Complications including renal arterial rupture, embolization, dissection or aneurysm were recorded.
Statistical analysis
SPSS version 26 (IBM, Armonk, NY, USA) was used for statistical analysis. Results are presented as median and range, mean and standard deviation (SD), interquartile range, and proportion. A paired t-test was used for comparisons of BPR, kidney size, KLR, and GFR. The number of antihypertensive drugs were compared using the Wilcoxon signed-rank test. Continuous and categorical variables affecting clinical benefit and restenosis were analyzed using the independent sample t-test and Fisher exact test, respectively. Multivariate analysis was performed to evaluate factors that may predict restenosis, using a logistic regression model. Kaplan-Meier survival analysis was performed to estimate the time-related clinical benefit after PTRA. P<0.05 denoted statistical significance.
Ethical statement
The study was conducted in accordance with the Declaration of Helsinki (as revised in 2013). The study was approved by the Institutional Review Board of the Peking University First Hospital (No. 2022-443-001) and informed consent was signed by the patients’ parents or legal guardians.
Results
As summarized in Table 1, among the 37 patients enrolled (21 boys and 16 girls), the mean age, weight, and height at first PTRA were 11.51±4.57 (range, 3–17) years, 45.37±22.29 (range, 13.40–106.00) kg, and 1.46±0.26 (range, 0.92–1.85) m, respectively. Twelve patients (32.4%) were asymptomatic, with renal artery stenosis manifesting as elevated BP on physical examination. Ten patients (27.0%) had mild symptoms, including mild dizziness, headache, nausea, or vomiting. After a multidisciplinary assessment, 15 patients (40.5%) were diagnosed with hypertensive target organ damage at discharge. Hypertensive encephalopathy, a hypertensive fundus lesion, and LVH were found in 4 (10.8%), 1 (2.7%), and 11 (29.7%) patients, respectively. Among them, 1 (2.7%) patient was diagnosed with both a hypertensive fundus lesion and LVH. Furthermore, 21 (56.8%) and 13 patients (35.1%) were diagnosed with FMD and TA, respectively. MMD was diagnosed in 2 children (5.4%), and NF1 in 1 child (2.7%).
Table 1
Sociodemographic and clinical characteristics | N (%)/mean ± SD |
---|---|
Sex | |
Male | 21 (56.8) |
Female | 16 (43.2) |
Age (years) | 11.51±4.57 |
Weight (kg) | 45.37±22.29 |
Height (m) | 1.46±0.26 |
Symptoms apart from hypertension | |
Asymptomatic | 12 (32.4) |
Mild symptoms | 10 (27.0) |
Target organ damage | 15 (40.5) |
Hypertensive encephalopathy | 4 (10.8) |
Hypertensive retinal fundus lesion | 1 (2.7) |
LVH | 11 (29.7) |
Etiology | |
FMD | 21 (56.8) |
TA | 13 (35.1) |
NF1 | 1 (2.7) |
MMD | 2 (5.4) |
Lesion side | |
Unilateral | 29 (78.4) |
Bilateral | 8 (21.6) |
Solitary kidney | 6 (16.2) |
SD, standard deviation; LVH, left ventricular hypertrophy; FMD, fibromuscular dysplasia; TA, Takayasu arteritis; NF1, neurofibromatosis type 1; MMD, moyamoya disease.
A total of 45 stenosed renal arteries were identified in 37 patients. Among them, 29 patients (78.4%) had unilateral renal artery stenosis and 6 of them (16.2%) had a solitary kidney. Bilateral lesions were found in eight patients (21.6%). In total, 24 arteries (53.3%) had ostial stenosis, 14 (31.1%) had truncal stenosis, and 7 (15.6%) had branch stenosis (Table 2). Additionally, 8 total occluded renal arteries were found in 7 patients (details are provided in Table S1).
Table 2
Lesion characteristics | N (%) |
---|---|
Lesion location | |
Ostial | 24 (53.3) |
Truncal | 14 (31.1) |
Branch | 7 (15.6) |
Total occlusive renal artery | 8 (17.8) |
Stenosis length | |
<15 mm | 23 (51.1) |
≥15 mm | 22 (48.9) |
Treatment | |
Recanalization failure | 2 (4.4) |
Balloon dilation only | 40 (88.9) |
Balloon dilatation and stent implantation | 3 (6.7) |
For treatment, 43 lesions were treated with balloon dilation, and in 3 lesions, balloon dilatation was followed by stent implantation due to severe residual stenosis (>50%). The technical success rate was 88.9% (40/45). Six total occluded renal arteries were successfully recanalized. In a patient with bilateral renal artery stenosis, the right renal artery with 80% stenosis was dilated successfully, whereas recanalization of the contralateral total occlusive lesion failed. There were no surgery-related complications.
In the 36 patients with PTRA, the SBPR decreased from 1.21±0.17 to 1.04±0.14 (t=9.122, P<0.001) and the DBPR decreased from 1.19±0.27 to 0.97±0.19 within 1 week post-procedure (t=7.634, P<0.001). The SBPR and DBPR of the 36 patients were 1.02±0.13 and 0.95±0.13, respectively, at last follow-up, with a median follow-up of 23.5 (range, 8–108) months. The number of antihypertensive drugs used in the 36 patients with PTRA also decreased from 3 [2, 3] to 2 [2, 2.75] within 1 week post-procedure (P<0.001), and was 1 [0, 2] at last follow-up. Eight patients (22.2%) were diagnosed with hypertensive target organ damage at the last follow-up. A hypertensive retinal fundus lesion and LVH were found in 1 (2.8%) and 8 (22.2%) patients, respectively (Table S2).
As summarized in Table 3, 83.3% (30/36) of patients achieved clinical benefit after PTRA, with 10 (27.8%) cured and 20 (55.6%) attaining improvements. Six patients were considered as a failure because of restenosis. The etiology and symptoms were statistically similar between patients with and without clinical failure (P=0.226 and P=0.569, respectively). The clinical benefit after initial PTRA was assessed using a Kaplan-Meier curve (Figure 1).
Table 3
Parameters | Clinical benefit, n (%) | P value |
---|---|---|
Etiology | 0.226 | |
FMD | 18 (90.0) | |
Non-FMD | 12 (75.0) | |
Symptoms apart from hypertension | 0.569 | |
Asymptomatic and mild symptoms | 18 (81.8) | |
Target organ damage | 12 (85.7) |
FMD, fibromuscular dysplasia.
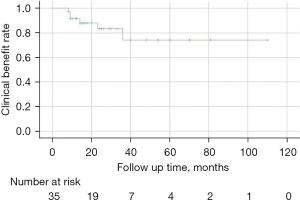
Restenosis occurred in 6 (16.7%) patients and 7 (16.3%) lesions post-procedure at the follow-up that occurred after a median of 7.5 (range, 3–14 months) (Figure 2). One patient with a bilateral restenosis artery with active-stage TA did not undergo endovascular treatment, and the SBPR and DBPR were controlled to 1.05 and 0.97 under antihypertensive drugs at the last follow-up. Four restenosis lesions underwent balloon dilatation, and re-restenosis was found in one lesion 36 months after the second procedure. The remaining restenosis lesion was seen in the NF1 patient in 10 months after the first PTRA and then treated with stent implantation. In-stent restenosis (ISR) occurred 8 months later. PTRA was performed for both re-restenosis lesions in two patients, and no stenosis was present at last follow-up.
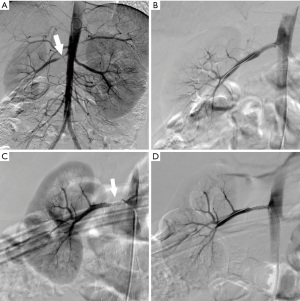
Clinical data and the lesion situation were assessed between lesions with restenosis and patent ones (Table 4). Ostial and long lesions were more likely to develop restenosis after PTRA (P=0.009 and P=0.038, respectively). Stenosis length, post-PTRA stenosis rate, age, and etiology were selected for multivariate analysis, but no variable showed a significant correlation with restenosis in the logistic regression model (Table 5). No patient received open surgery as a reintervention during the follow-up time.
Table 4
Parameters | Restenosis, n (%) | P value |
---|---|---|
Sex | 0.695 | |
Male | 5 (18.5) | |
Female | 2 (12.5) | |
Etiology | 0.680 | |
FMD | 3 (12.5) | |
Non-FMD | 4 (21.1) | |
Symptoms apart from hypertension | 0.999 | |
Asymptomatic and mild symptoms | 4 (14.8) | |
Target organ damage | 3 (18.8) | |
Lesion location | 0.009 | |
Ostial | 7 (31.8) | |
Non-ostial | 0 (0.0) | |
Stenosis length | 0.038 | |
<15 mm | 1 (4.3) | |
≥15 mm | 6 (30.0) | |
Degree of stenosis | 0.681 | |
Occlusive | 1 (16.7) | |
Non-occlusive | 6 (16.2) | |
Post-PTRA stenosis rate | 0.222 | |
<15% | 2 (8.7) | |
15–30% | 5 (25.0) | |
Number of balloons | 0.680 | |
1 | 5 (20.0) | |
2 | 2 (11.1) |
FMD, fibromuscular dysplasia; PTRA, percutaneous transluminal renal angioplasty.
Table 5
Parameters | Beta | Odds ratio (95% CI) | P |
---|---|---|---|
Stenosis length | 0.095 | 1.100 (0.977–1.239) | 0.115 |
Post-PTRA stenosis rate | 0.710 | 2.034 (0.281–14.700) | 0.482 |
Age | 0.049 | 1.051 (0.829–1.332) | 0.683 |
Etiology | 0.473 | 1.605 (0.251–10.277) | 0.618 |
CI, confidence interval; PTRA, percutaneous transluminal renal angioplasty.
In patients treated with PTRA for RVH, sixteen kidneys were measured in the sagittal plane longitudinally by ultrasound at least two times before PTRA. After a follow-up of 9 (range, 3–14 months), sixteen kidneys size changed from 8.83±1.64 to 8.69±1.81 (t=1.980, P=0.066). Twenty-nine kidney lengths were measured before the procedure and at the last follow-up by ultrasound. The mean kidney length and KLR increased from 8.89±1.55 cm and 29.47%±17.28% before the procedure to 9.79±1.51 cm (t=−5.50, P<0.001) and 40.13±11.37 % (t=−3.56, P=0.001) at 18-month (range, 4–104 months) follow-up after initial PTRA.
A DTPA scan was performed on 34 kidneys with renal artery stenosis after PTRA. At 20-month (range, 3–72 months) follow-up after PTRA, the mean GFR significantly increased from 32.28±19.22 to 41.24±13.24 mL/min (t=−4.06, P<0.001).
Discussion
In this 12-year retrospective single-center study of 37 children with RVH, PTRA was associated with high technical success and acute and long-term clinical benefit in terms of BP control, reduction in number of medications, mean kidney length, KLR, and GFR.
With various definition in different guidelines, diagnosis of hypertension in children is often delayed due to hidden onset and neglect of children BP measurement (30). Once elevated BP is detected, children are usually accompanied by severe symptoms, including persistent BP >99th percentile or acute end-organ dysfunction, defined as hypertensive crisis (31). The diagnosis of RVH is challenging, with no consensus regarding which diagnostic modalities to use in children with suspected RVH (32). When RVH is suspected, careful history and physical examination are needed. Initial laboratory tests, including serum creatinine, electrolytes, fasting plasma glucose, renin assays and thyroid hormone assays, help to assess comorbidities and target organ damage in children, and to rule out other causes of hypertension, such as primary aldosteronism and hyperthyroidism (33). Secondly, imaging studies are essential for the diagnosis of RVH. The sensitivity and specificity of Doppler ultrasound with RVH children range from 63–90% to 68–95%, respectively, affected by professional’s ability, patient’s cooperation, children’s age and body mass index (34). Magnetic resonance angiography (MRA) relies highly on patient cooperation to avoid motion artifacts, while computed tomography angiography (CTA) involves ionizing radiation exposure. As the gold standard for diagnosis of RVH, DSA offers the additional advantage of possible treatment during the same procedure. PTRA is indicated for RVH patients when medication is insufficient. Based on the previous literature standards and multidisciplinary team decision, PTRA was performed in pediatric patients with stenosis ≥60% in our center (1,19). For hypertension children with mild renal artery stenosis, careful follow-up was insisted, monitoring ultrasonic image of renal artery, renin-angiotensin-aldosterone system and medical therapy to gain well BP control. Rates of PTRA technical success, beneficial BP response, and restenosis were found to be 93.9%, 68.4%, and 25.5%, respectively, in a meta-analysis of PTRA based on 17 observational noncontrolled studies involving 384 pediatric patients (35), and are comparable to those in the present study.
FMD and TA are the main causes of RVH in children; rarer causes include NF1, MMD, and external compression by tumor (1). In this series, 56.8% of RVH cases were secondary to FMD, which is consistent with findings in previous Chinese study (18), although TA was the leading cause of RVH in Asian children in one study (1), and it has been noted that it is challenging to definitively distinguish between FMD and TA, as TA frequently presents nonspecific clinical findings and no inflammatory components (25). PTRA is not recommended for TA patients when patients are in active disease or are responding well to medications (24). Although some prior studies reported a better response in the FMD group (4,14), there was no significant difference in outcomes by etiology in this study.
There is a general delay in the diagnosis of pediatric hypertension due to insufficient awareness and the difficulty of BP measurements during childhood (1). Children with RVH often exhibit sharply elevated BP with poor medication control, resulting in damage to target organs. In this study, target organ damage occurred in 15 (40.5%) patients, 11 of whom were diagnosed with LVH. However, asymptomatic or mild RVH was not associated with clinical benefit in terms of PTRA outcomes.
In this study, 83.3% of patients with RVH achieved clinical benefit, and the rest experienced restenosis. However, all patients experienced a decline in BP or antihypertensive medicine reduction after PTRA, which was sustained in the long-term. Recanalization failed in two total occlusive lesions, whereas six total occlusive arteries were successfully dilated. Although the residual stenosis rate and the number of balloons used are expected to reflect the complexity of lesion dilatation, these factors did not reach statistical significance in this study. Ostial stenosis and a lesion length ≥15 mm were strongly associated with restenosis (P=0.009 and P=0.038, respectively), which is partially consistent with the results of Sharma et al. and Zhu et al. (3,11), and may be due to abdominal aorta involvement, which is more likely to cause elastic recoil in ostial stenosis. A longer lesion indicates more fibrotic vessels and the persistence of vessel wall inflammation despite clinical or laboratory evidence of quiescent disease (36). TA is prone to affect long segmental arteries and the origin of the primary branches of the aorta; this study, however, did not show a significant difference between the tendency of postoperative restenosis and RVH etiology. It should be noted that the multivariate analysis did not identify a predictor of restenosis. We attributed this to limited data, which were not sufficient to make a convincing multivariable logistic regression model.
Currently, balloon angioplasty is the first-line endovascular intervention for pediatric RVH. At our center, stenting was deployed in children for the following indications: (I) elastic recoil after balloon dilatation; (II) renal artery flow-limiting dissection; and (III) acute occlusion of the lesion during the procedure. In this series, stent deployment was applied to 3 total occlusive lesions out of the 43 first-time-treated lesions. Of the seven restenosis lesions, one restenosis lesion was treated with stenting and developed re-restenosis 8 months later, requiring another PTRA. In general, there are potential long-term drawbacks to deploying renal artery stents in children, including an elevated incidence of restenosis and the failure of stents to adapt to the expected growth of the kidneys and renal arteries (1,12). Rare postoperative complications of stent fractures have also been reported (18,37). We recommend to avoid using stents for the treatment of non-refractory renal artery lesions.
The size of the affected kidney should be considered for evaluation of PTRA’s long-term efficacy. In the study by Chung et al. (14), kidneys smaller than two standard deviations below the average value (z-score <−2) were defined as atrophy. The percentage of atrophic kidneys became higher at follow-up in the medication group compared to the PTRA group (60.0% vs. 26.1%, P=0.037) (14). By restoring renal blood flow, the affected kidneys were significantly enlarged with improved KLR percentile value. The increased length of the kidney after PTRA predicts the recovery of parenchyma and the long-term preservation of renal function.
The recovery of renal function is an essential reference for determining the long-term benefits of PTRA in children. In a previous study, the estimated GFR was calculated to assess changes in renal function after PTRA in children with renal artery stenosis. Due to the compensatory mechanism from the healthy contralateral kidney, split renal function is considered to be more accurate. Using a DTPA scan, Zhao et al. evaluated 17 split renal functions with a GFR <40 mL/min before the initial procedure. The mean GFR of affected kidneys significantly increased from 19.85±11.24 to 38.09±1.88 mL/min at the 6-month follow-up after initial PTRA (18). In our study, changes in 34 kidneys assessed by a DTPA scan showed long-term renal function improvement in pediatric RVH patients. Long-lasting hypoperfusion may lead to severe and irreversible renal atrophy, even in RVH children who are well-controlled by antihypertensive drugs. Prompt revascularization is essential to protect long-term renal function.
The present study has the inherent limitations and biases of its retrospective, single-center design, which calls for caution in result interpretation and generalization. The sample size was small because of the low incidence of pediatric RVH. For longer-term assessments, we failed to eliminate the influence of the natural development of kidney function on preoperative and postoperative GFR changes in affected kidneys. Lastly, the lack of natural renal function data in RVH children without interventional treatment precluded assessing whether PTRA outperforms medical therapy alone in long-term renal function preservation.
Conclusions
In conclusion, PTRA can achieve promising results in pediatric RVH. Long-term renal artery patency is satisfactory after PTRA, and restenosis was associated with lesion location and length. Angioplasty significantly improved BP control and preserved kidney function, as was reflected by the increase in affected kidney size and the higher GFR.
Acknowledgments
Funding: This work was supported by
Footnote
Reporting Checklist: The authors have completed the STROBE reporting checklist. Available at https://tp.amegroups.com/article/view/10.21037/tp-23-215/rc
Data Sharing Statement: Available at https://tp.amegroups.com/article/view/10.21037/tp-23-215/dss
Peer Review File: Available at https://tp.amegroups.com/article/view/10.21037/tp-23-215/prf
Conflicts of Interest: All authors have completed the ICMJE uniform disclosure form (available at https://tp.amegroups.com/article/view/10.21037/tp-23-215/coif). The authors have no conflicts of interest to declare.
Ethical Statement: The authors are accountable for all aspects of the work in ensuring that questions related to the accuracy or integrity of any part of the work are appropriately investigated and resolved. The study was conducted in accordance with the Declaration of Helsinki (as revised in 2013). The study was approved by the Institutional Review Board of the Peking University First Hospital (No. 2022-443-001) and informed consent was signed by the patients’ parents or legal guardians.
Open Access Statement: This is an Open Access article distributed in accordance with the Creative Commons Attribution-NonCommercial-NoDerivs 4.0 International License (CC BY-NC-ND 4.0), which permits the non-commercial replication and distribution of the article with the strict proviso that no changes or edits are made and the original work is properly cited (including links to both the formal publication through the relevant DOI and the license). See: https://creativecommons.org/licenses/by-nc-nd/4.0/.
References
- Patel PA, Cahill AM. Renovascular hypertension in children. CVIR Endovasc 2021;4:10.
- Casalini E, Sfondrini MS, Fossali E. Two-year clinical follow-up of children and adolescents after percutaneous transluminal angioplasty for renovascular hypertension. Invest Radiol 1995;30:40-3. [Crossref] [PubMed]
- Sharma S, Thatai D, Saxena A, et al. Renovascular hypertension resulting from nonspecific aortoarteritis in children: midterm results of percutaneous transluminal renal angioplasty and predictors of restenosis. AJR Am J Roentgenol 1996;166:157-62. [Crossref] [PubMed]
- Tyagi S, Kaul UA, Satsangi DK, et al. Percutaneous transluminal angioplasty for renovascular hypertension in children: initial and long-term results. Pediatrics 1997;99:44-9. [Crossref] [PubMed]
- Courtel JV, Soto B, Niaudet P, et al. Percutaneous transluminal angioplasty of renal artery stenosis in children. Pediatr Radiol 1998;28:59-63. [Crossref] [PubMed]
- Shroff R, Roebuck DJ, Gordon I, et al. Angioplasty for renovascular hypertension in children: 20-year experience. Pediatrics 2006;118:268-75. [Crossref] [PubMed]
- Bayazit AK, Yalcinkaya F, Cakar N, et al. Reno-vascular hypertension in childhood: a nationwide survey. Pediatr Nephrol 2007;22:1327-33. [Crossref] [PubMed]
- Bayrak AH, Numan F, Cantaşdemir M, et al. Percutaneous balloon angioplasty of renovascular hypertension in pediatric cases. Acta Chir Belg 2008;108:708-14. [Crossref] [PubMed]
- Radanović B, Cacić Z, Perkov D, et al. Endovascular therapy of renovascular hypertension in children: single center analysis. Eur J Pediatr Surg 2009;19:135-40. [Crossref] [PubMed]
- Srinivasan A, Krishnamurthy G, Fontalvo-Herazo L, et al. Angioplasty for renal artery stenosis in pediatric patients: an 11-year retrospective experience. J Vasc Interv Radiol 2010;21:1672-80. [Crossref] [PubMed]
- Zhu G, He F, Gu Y, et al. Angioplasty for pediatric renovascular hypertension: a 13-year experience. Diagn Interv Radiol 2014;20:285-92. [Crossref] [PubMed]
- Kari JA, Roebuck DJ, McLaren CA, et al. Angioplasty for renovascular hypertension in 78 children. Arch Dis Child 2015;100:474-8. [Crossref] [PubMed]
- Alexander A, Richmond L, Geary D, et al. Outcomes of percutaneous transluminal angioplasty for pediatric renovascular hypertension. J Pediatr Surg 2017;52:395-9. [Crossref] [PubMed]
- Chung H, Lee JH, Park E, et al. Long-Term Outcomes of Pediatric Renovascular Hypertension. Kidney Blood Press Res 2017;42:617-27. [Crossref] [PubMed]
- Agrawal H, Moodie D, Qureshi AM, et al. Interventions in children with renovascular hypertension: A 27-year retrospective single-center experience. Congenit Heart Dis 2018;13:349-56. [Crossref] [PubMed]
- Lee Y, Lim YS, Lee ST, et al. Pediatric renovascular hypertension: Treatment outcome according to underlying disease. Pediatr Int 2018;60:264-9. [Crossref] [PubMed]
- Lobeck IN, Alhajjat AM, Dupree P, et al. The management of pediatric renovascular hypertension: a single center experience and review of the literature. J Pediatr Surg 2018;53:1825-31. [Crossref] [PubMed]
- Zhao L, Zhao X, Hu X, et al. Midterm Outcomes of Angioplasty for Pediatric Renovascular Hypertension. J Vasc Interv Radiol 2022;33:399-407. [Crossref] [PubMed]
- Guo J, Wu S, Zhang F, et al. Long-term outcomes of angioplasty for pediatric renovascular hypertension: A single-center experience. Vascular 2023;31:122-30. [Crossref] [PubMed]
- Flynn JT, Kaelber DC, Baker-Smith CM, et al. Clinical Practice Guideline for Screening and Management of High Blood Pressure in Children and Adolescents. Pediatrics 2017;140:e20171904. Erratum in: Pediatrics 2017;140:e20173035 Erratum in: Pediatrics 2018;142:e20181739. [Crossref] [PubMed]
- Seeman T, Hamdani G, Mitsnefes M. Hypertensive crisis in children and adolescents. Pediatr Nephrol 2019;34:2523-37.
- Lopez L, Colan SD, Frommelt PC, et al. Recommendations for quantification methods during the performance of a pediatric echocardiogram: a report from the Pediatric Measurements Writing Group of the American Society of Echocardiography Pediatric and Congenital Heart Disease Council. J Am Soc Echocardiogr 2010;23:465-95; quiz 576-7. [Crossref] [PubMed]
- Cantinotti M, Scalese M, Danford D, et al. Reference Standards in Quantitative Pediatric Echocardiography: A Guide to the Nuanced World of Z Scores and Nomograms. J Am Soc Echocardiogr 2023;36:324-6. [Crossref] [PubMed]
- Grayson PC, Ponte C, Suppiah R, et al. 2022 American College of Rheumatology/EULAR classification criteria for Takayasu arteritis. Ann Rheum Dis 2022;81:1654-60. [Crossref] [PubMed]
- Gornik HL, Persu A, Adlam D, et al. First International Consensus on the diagnosis and management of fibromuscular dysplasia. Vasc Med 2019;24:164-89. [Crossref] [PubMed]
- Fujimura M, Tominaga T, Kuroda S, et al. 2021 Japanese Guidelines for the Management of Moyamoya Disease: Guidelines from the Research Committee on Moyamoya Disease and Japan Stroke Society. Neurol Med Chir (Tokyo) 2022;62:165-70. [Crossref] [PubMed]
- Cimino PJ, Gutmann DH. Neurofibromatosis type 1. Handb Clin Neurol 2018;148:799-811. [Crossref] [PubMed]
- Nelson O, Bailey PD Jr. Pediatric Anesthesia Considerations for Interventional Radiology. Anesthesiol Clin 2017;35:701-14. [Crossref] [PubMed]
- Rosenbaum DM, Korngold E, Teele RL. Sonographic assessment of renal length in normal children. AJR Am J Roentgenol 1984;142:467-9. [Crossref] [PubMed]
- Tain YL, Hsu CN. Cardiovascular Risks of Hypertension: Lessons from Children with Chronic Kidney Disease. Children (Basel) 2022;9:1650. [Crossref] [PubMed]
- Xu L, Ba H, Jiang X, et al. Hypertension crisis as the first symptom of renovascular hypertension in children. Ital J Pediatr 2022;48:191. [Crossref] [PubMed]
- Kurt-Sukur ED, Brennan E, Davis M, et al. Presentation, treatment, and outcome of renovascular hypertension below 2 years of age. Eur J Pediatr 2022;181:3367-75. [Crossref] [PubMed]
- de Simone G, Mancusi C, Hanssen H, et al. Hypertension in children and adolescents. Eur Heart J 2022;43:3290-301. [Crossref] [PubMed]
- de Oliveira Campos JL, Bitencourt L, Pedrosa AL, et al. Renovascular hypertension in pediatric patients: update on diagnosis and management. Pediatr Nephrol 2021;36:3853-68. [Crossref] [PubMed]
- Zhao X, Zhao L, Wu L, et al. Efficacy of percutaneous transluminal renal angioplasty for pediatric renovascular hypertension: a meta-analysis. Zhonghua Er Ke Za Zhi 2020;58:661-7. [Crossref] [PubMed]
- Kim ESH, Beckman J. Takayasu arteritis: challenges in diagnosis and management. Heart 2018;104:558-65.
- Vijayvergiya R, Jindal AK, Pilania RK, et al. Complex interventions of abdominal aorta and its branches in children with Takayasu arteritis: Clinical experience from a tertiary care center in north-west India. Int J Rheum Dis 2019;22:140-51. [Crossref] [PubMed]