Whole-exome sequencing revealed novel genetic alterations in patients with tetralogy of Fallot
Highlight box
Key findings
• We performed whole-exome sequencing (WES) in a large cohort of tetralogy of Fallot (TOF) patients and identified very rare deleterious variations in several genes.
What is known and what is new?
• TOF is a complex congenital heart disease with clinical and genetic heterogeneity.
• We enrolled 17 patients with TOF in this study. Among these patients, 14 had mutations in TOF-related genes, including GJB2, TBX15, CTNS, SPINK1, GATA6, PRIMOL, GDF15, SLC17A9, AIFM1, FOXC2, KLF13, ABCA4, CPA6, FKBP10, ASPA, SBF1, HBA2, IGLL1, GNE, and KLHL10.
What is the implication, and what should change now?
• Our findings may help clinicians determine the potential of WES in detecting TOF and implement early interventions for patients with TOF.
Introduction
Congenital heart disease (CHD) is the most prevalent birth abnormality, and affects around eight out of every 1,000 infants born (1-3). Tetralogy of Fallot (TOF) is the most prevalent and complicated cyanotic CHD, and affects around one out of every 2,500 newborns (4,5). TOF is a condition that affects the heart’s outflow tract and is defined by four specific structural abnormalities identified postnatally: a ventricular septal defect, an anterocephalad deviation of the outflow septum with aortic override, a variable obstruction of the right ventricular outflow tract (RVOT) (pulmonary stenosis), and right ventricle hypertrophy (6-9). With the help of surgical procedures performed throughout childhood, 85–90% of TOF patients now live until the age of at least 30 years (10). However, due to the severe morbidity associated with the scar tissue following surgery and pulmonary regurgitation in adulthood, only 25% of TOF patients have an event-free life beyond the age of 40 years (11,12).
The etiology of TOF is unknown, and no single gene is responsible for the phenotypic characteristics of the disease (13,14). Research on syndromic TOF sufferers has provided crucial insights into some causal genes (15). Notably, 20% of the cases have been linked to a known syndrome or chromosomal abnormality (16). About 15% of TOF patients have 22q11.2 deletion syndrome for which T-box transcription factor is the primary cause (17,18). About 80% of TOF cases are non-syndromic, but the etiology of non-syndromic TOF is not known, mostly because the inheritance is non-Mendelian (19-21). As a result, a polygenic genetic architecture has been theorized and genome-wide techniques have been used to gain insights into the complicated genetic abnormalities responsible for TOF and other CHDs (20-23).
Research has shown that whole-exome sequencing (WES) may reveal novel CHD candidate genes (24). The aim of this study was to find TOF-related pathogenic genes through WES technology performed on the genes of 17 TOF patients, providing a basis for studying TOF pathogenesis. We present this article in accordance with the MDAR reporting checklist (available at https://tp.amegroups.com/article/view/10.21037/tp-23-449/rc).
Methods
Serum sample collection
A total of 17 patients with TOF who underwent cardiac surgery (7 males and 10 females) at Guizhou Provincial People’s Hospital between March 2018 and July 2021 were included in this study. TOF was confirmed by echocardiography, clinical symptoms, signs, and intraoperative findings. Inclusion criteria were confirmed diagnosis of TOF (via review of the medical chart and echocardiograms). Exclusion criteria were patients with TOF with pulmonary atresia, absent of pulmonary valve, or placement of right ventricle to pulmonary artery conduit in case of large coronary artery branch crossing RVOT. The patients’ specific features are detailed in Table 1. The study protocol was approved by the Ethics Committee of Guizhou Provincial People’s Hospital (No. 2018040) and was performed in accordance with the Declaration of Helsinki (as revised in 2013). Informed consent was obtained from the enrolled participants or their legal guardians.
Table 1
Case ID | Age (years) | Sex | Echocardiography | Acropachia | Cyanosis | ||
---|---|---|---|---|---|---|---|
RV diastolic diameter (mm) | RVAW (thickened) (mm) | RVOT (mm) | |||||
TOF-1 | 3 | Female | 18.4 | 7.0 | 6.1 | No | Yes |
TOF-2 | 5 | Female | 11.5 | 8.6 | 4.0 | No | No |
TOF-3 | 3 | Male | 14.5 | 4.6 | 5.2 | No | Yes |
TOF-4 | 2 | Female | 10.7 | 5.8 | 5.0 | No | Yes |
TOF-5 | 22 | Female | 24.2 | 6.4 | 7.0 | Yes | Yes |
TOF-6 | 2 | Female | 13.2 | 7.0 | 5.2 | No | No |
TOF-7 | 29 | Female | 22.6 | 12.6 | 6.0 | No | Yes |
TOF-8 | 1 | Female | 8.0 | 6.5 | 7.2 | No | Yes |
TOF-9 | 1 | Male | 14.4 | 6.0 | 6.8 | Yes | Yes |
TOF-10 | 3 | Male | 15.9 | 7.4 | – | Yes | Yes |
TOF-11 | 56 | Male | 28.1 | 8.9 | 12.0 | Yes | Yes |
TOF-12 | 25 | Female | 22.9 | 8.0 | 4.0 | No | Yes |
TOF-13 | 2 | Male | 13.2 | 5.7 | 5.5 | No | Yes |
TOF-14 | 30 | Female | 22.9 | 10.0 | 12.5 | Yes | Yes |
TOF-15 | 12 | Female | 20.2 | 9.2 | 5.0 | Yes | Yes |
TOF-16 | 1 | Male | 13.0 | 7.1 | 3.5 | No | Yes |
TOF-17 | – | Male | – | – | – | – | – |
TOF, tetralogy of Fallot; RV, right ventricle; RVAW, right ventricular anterior wall; RVOT, right ventricular outflow tract.
DNA sample preparation
Genomic DNA was extracted using a QIAamp DNA Blood Kit (TransGen Biotech, China) from peripheral blood samples taken from the patients.
WES and data analysis
The Agilent SureSelect Human Exon V5 kit (Agilent Technologies) was used to extract and collect genomic DNA from the blood to generate the library for WES. The library preparations were sequenced on the Illumina HiSeq 2000 platform, supplied by Illumina Inc. (San Diego, CA, USA) and 100-bp paired-end reads were yielded.
Validation by Sanger sequencing
The primers for the standard polymerase chain reaction assays were designed using Primer 3 software (https://bioinfo.ut.ee/primer3-0.4.0/). Sanger sequencing was used to analyze the amplified DNA fragments directly. The DNASTAR program (https://dnastar.com/) was used to assess the sequencing data by comparing the findings to the reference sequences. The whole sequenced data were trimmed for low-quality sequences and aligned to UCSC human reference genome (GRCh38/HG38) using Burrows-Wheeler Alignment (BWA). The Genome Analysis Toolkit (GATK) and VarScan were used to detect single nucleotide polymorphisms (SNPs) and small insertions/deletions. ANNOVAR was used to annotate the variants with several databases including dbSNP, gnomAD, 1000 Genomes Project, and ExAC. Finally, four online mutation pathogenicity prediction and analysis softwares including PolyPhen, SIFT, MutationTaster, and FATHMM were used to predict the influence of polymorphic variation on coding proteins and conservation, so as to conduct mutation pathogenicity analysis. The primer sequences were as follows: forward primer (FKBP10-F), 5'-GGTCTTTCACGTCCTCCTGA-3', reverse primer (FKBP10-R), 5'-GAATCGAAGAGGGTGCCGT-3'; forward primer (GNE-F), 5'-TGGAGACAGGTTTGATGCCC-3', reverse primer (GNE-R), 5'-TGTCATAGGAAGGGCAGCCT-3'.
Statistical analysis
SPSS 20.0 statistical software was used to analyze the data. The number of mutations, disease-related mutations and sequencing depth of patients were listed and analyzed.
Results
Clinical features of patients with TOF
A total of 17 TOF patients were included in this study and the clinical features of the TOF patients are listed in Table 1.
Analysis of the WES findings in patients with TOF
WES was used to examine the genetic etiology of TOF in the 17 patients. Only variants with a minor allele frequency of <1% in the Single Nucleotide Polymorphism Database (dbSNP), 1000G (1000 Genomes Project, http://www.internationalgenome.org/), ESP6500 (Exome Variant Server, http://evs.gs.washington.edu/EVS/), and gnomAD databases (Genome Aggregation database, https://gnomad.broadinstitute.org/) were retained. The retained variations were filtered based on the TOF candidate genes that had been chosen. In total, 21 variations in 20 genes were discovered in 14 individuals, including 21 heterozygous variants in GJB2 (Gap Junction Protein Beta 2), TBX15 (T-Box Transcription Factor 15), CTNS (Cystinosin, Lysosomal Cystine Transporter), SPINK1 (Serine Peptidase Inhibitor Kazal Type 1), GATA6 (GATA Binding Protein 6), PRIMOL (Homo sapiens primase and DNA directed polymerase), GDF15 (Growth Differentiation Factor 15), SLC17A9 (Solute Carrier Family 17 Member 9), AIFM1 (Apoptosis Inducing Factor Mitochondria Associated 1), FOXC2 (Forkhead Box C2), KLF13 (KLF Transcription Factor 13), ABCA4 (ATP Binding Cassette Subfamily A Member 4), CPA6 (Carboxypeptidase A6), FKBP10 (FKBP Prolyl Isomerase 10), ASPA (aspartoacylase), SBF1 (SET Binding Factor 1), HBA2 (Hemoglobin Subunit Alpha 2), IGLL1 (Immunoglobulin Lambda Like Polypeptide 1), GNE (Glucosamine (UDP-N-Acetyl)-2-Epimerase/N-Acetylmannosamine Kinase), and KLHL10 (Kelch Like Family Member 10) (Table 2). None of the above genes were discovered in the WES data of the three participants in the control group.
Table 2
Case ID | Gene | Variations (mRNA) | Variations (amino acid) | Pathogenicity evidence | gnomAD |
---|---|---|---|---|---|
TOF-1 | GJB2 | NM_004004.6:c.299_300del | p.His100fs | P | f=4.1e-5 |
TBX15 | NM_001330677.2:c.980G>A | p.Arg327His | VUS | f=5e-4 | |
TOF-2 | CTNS | NM_004937.3:c.611_613ACG | p.Asp205del | LP | f=8.2e-6 |
TOF-3 | SPINK1 | NM_001354966.1:c.194+2T>C | splicing | P | f=2.8e-4 |
TOF-4 | GATA6 | NM_005257.5:c.151G>A | p.E51K | P | f=1.7e-4 |
VSD | NM_001300767.2:c.-123T>G | NA | P | f=4e-4 | |
GDF15 | NM_004864.3:c.651G>C | p.Ala218Pro | VUS | f= NA | |
TOF-5 | SLC17A9 | NM_001302643.2:c.-56C>T | p.Arg9Cys | P | f= NA |
TOF-8 | AIFM1 | NM_001130846.3:c.13C>T | p.Leu5Phe | P | f=2.3e-4 |
FOXC2 | NM_005251.2:c.1061T>A | p.Leu354Gln | LP | f= NA | |
TOF-9 | KLF13 | NM_001302461.2:c.319T>A | p.Ser107Thr | VUS | f= NA |
TOF-11 | PRIMOL1 | NM_001300767.2:c.-123T>G | NA | P | f=4e-4 |
ABCA4 | NM_000350.3:c.1531C>T | p.Arg511Cys | LP | f=2.1e-4 | |
KLF13 | NM_001302461.2:c.319T>A | p.Ser107Thr | VUS | f= NA | |
TOF-12 | CPA6 | NM_020361.5:c.544C>T | p.Arg182Ter | LP | f=2.5e-5 |
TOF-13 | FKBP10 | NM_021939.3:c.831dupC | p.Gly278fs | P | f=1.9e-4 |
TOF-14 | ASPA | NM_000049.3:c.79G>A | p.Gly27Arg | P | f=1.6e-5 |
SBF1 | NM_001365819.1:c.4693A>G | p.Thr1565Ala | LP | f=3.2e-4 | |
TOF-15 | HBA2 | NM_000517.6:c.427T>C | p.Ter143Gln | P | f=4.9e-5 |
IGLL1 | NM_001369906.1:c.428C>T | p.Pro143Leu | LP | f=2e-4 | |
TOF-16 | GNE | NM_005476.7:c.527A>T | p.Asp176Val | LP | f=4.1e-5 |
KLF13 | NM_001302461.2:c.310G>C | p.Glu104Gln | VUS | f= NA | |
TOF-17 | KLHL10 | NM_001329596.2:c.673G>A | p.Ala225Thr | P | f=4.2e-4 |
IGLL1 | NM_001369906.1:c.428C>T | p.Pro143Leu | LP | f=2e-4 |
TOF, tetralogy of Fallot; P, pathogenic; VUS, variants of uncertain significance; LP, likely pathogenic; NA, not available.
In addition, we analyzed the clinical symptoms of the TOF patients with gene variations and found that patients with FKBP10 and GEN variants had more serious clinical symptoms (thickening and obvious stenosis of RVOT, pulmonary valve thickening and stenosis). Sanger sequencing was used to validate the variants. Heterozygous variants c.831dupC in FKBP10 (Figure 1A) and c.527A>T in GEN (Figure 1B) were identified in the TOF patients.
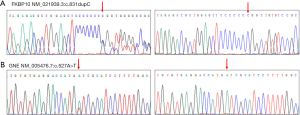
Analyses of the variations discovered in the TOF patients
An in-silico analysis was conducted to analyze the 21 different variations. First, we examined the frequency with which the variations appeared. The gnomAD database (Genome Aggregation database, https://gnomad.broadinstitute.org/) reported that all of these variants were unusual, and six of these variants were not found in the 1000G databases (1000 Genomes Project, http://www.internationalgenome.org/). Thus, the frequency of these mutations in the study was very low, which matched the phenomenon of the low incidence of TOF in the population (Table 2). We grouped variants into the following categories according to guidelines from the American College of Medical Genetics and Genomics (ACMG) and Association of Molecular Pathology (AMP): pathogenic (P), likely pathogenic (LP), variant of uncertain significance (VUS), likely benign (LB) and benign (B) (25).
Discussion
The first clinical application of WES indicated that the success rate of mutation detection in individuals with a genetic illness was roughly 25% (26). A recent WES study has identified variants in NOTCH1 and FLT4, the most commonly observed genes, in 7% of TOF cases (27). Jin et al. found dominant FLT4 mutations accounting for 2.3% of TOF cases (28). Some of the mutations in our top gene candidates were de novo, while others were found in patients who appeared to be asymptomatic, which suggested that penetrance was only partially achieved. WES was conducted to analyze the DNA samples of 17 TOF patients, and 21 mutations related to TOF were found in 14 of the included patients. Our findings support the idea that genetic variations in several genes have a role in TOF etiology and that sporadic cases of unknown origin result from a common genetic variation in one of these genes.
FK506 binding protein 10 is encoded by the FKBP10 gene, which is found on chromosome 17q21.2 and belongs to the immunophilins family of proteins with Peptidylprolyl cis/trans isomerase (PPIase) activity, which aids in the appropriate folding of type I collagen before the triple helices are assembled (29). Alanay et al. in 2010 reported that a mutation of the FKBP10 gene was involved in osteogenesis imperfecta (OI) type XI (30). A total of 191 somatic FKBP10 point mutations that are not synonymous have been documented in the COSMIC database (http://cancer.sanger.ac.uk/cosmic). In the Yup ik Inuit community, Barnes et al. discovered the biallelic c.877 879delTAC (p.Tyr293del) mutation in FKBP10, which causes Kuskokwim syndrome with subtle skeletal symptoms, such as congenital contractures and osteopenia without fractures (31). In this study, we identified a novel mutation, c.831dupC, in a patient with TOF. Moreover, a mutation in GNE (UDP-N-acetyloglucosamine 2-epimerase/N-acetylmannosamine kinase) was identified. The GNE c.527A>T (p.Asp176Val) variant is a well-described pathogenic variant (32,33). Previous research has shown that 20% of GNE myopathy mice (30 weeks of age) develop endomysial fibrosis, amyloid deposition, and ringed vacuoles in cardiomyocytes (34).
Owing to the complexity of TOF, for personalized diagnosis and treatment for TOF patients, WES may be necessary to help identify the pathological mutations. The use of WES may not only reveal the genetic pathophysiology of individuals with unexplained TOF, but may also have extensive potential on the application in the screening and early identification of patients with the disorder. The poor treatment results and the uncertain etiology of TOF cause patients to suffer both psychologically and physically. More effective treatment options will be able to be designed and patient compliance will probably increase if the genetic causes of the disease can be identified early in the course of illness development. In addition, future causality studies are warranted to translate the observations into preclinical validation. This study has some limitations, and the sample size is small. In future, the number of samples should be further increased and an in-depth research will be conducted.
Conclusions
In conclusion, we identified several genetic variants that were associated with TOF and confirmed that variants of FKBP10 and GNE were associated with TOF severity, which help determine the potential of WES in detecting TOF and implement early interventions for patients with TOF.
Acknowledgments
Funding: This study was supported by
Footnote
Reporting Checklist: The authors have completed the MDAR reporting checklist. Available at https://tp.amegroups.com/article/view/10.21037/tp-23-449/rc
Data Sharing Statement: Available at https://tp.amegroups.com/article/view/10.21037/tp-23-449/dss
Peer Review File: Available at https://tp.amegroups.com/article/view/10.21037/tp-23-449/prf
Conflicts of Interest: All authors have completed the ICMJE uniform disclosure form (available at https://tp.amegroups.com/article/view/10.21037/tp-23-449/coif). The authors have no conflicts of interest to declare.
Ethical Statement: The authors are accountable for all aspects of the work in ensuring that questions related to the accuracy or integrity of any part of the work are appropriately investigated and resolved. The study protocol was approved by the Ethics Committee of Guizhou Provincial People’s Hospital (No. 2018040) and was performed in accordance with the Declaration of Helsinki (as revised in 2013). Informed consent was obtained from the enrolled participants or their legal guardians.
Open Access Statement: This is an Open Access article distributed in accordance with the Creative Commons Attribution-NonCommercial-NoDerivs 4.0 International License (CC BY-NC-ND 4.0), which permits the non-commercial replication and distribution of the article with the strict proviso that no changes or edits are made and the original work is properly cited (including links to both the formal publication through the relevant DOI and the license). See: https://creativecommons.org/licenses/by-nc-nd/4.0/.
References
- Hoffman JI, Kaplan S. The incidence of congenital heart disease. J Am Coll Cardiol 2002;39:1890-900. [Crossref] [PubMed]
- Goldmuntz E. The epidemiology and genetics of congenital heart disease. Clin Perinatol 2001;28:1-10. [Crossref] [PubMed]
- Majumdar U, Yasuhara J, Garg V. In Vivo and In Vitro Genetic Models of Congenital Heart Disease. Cold Spring Harb Perspect Biol 2021;13:a036764. [Crossref] [PubMed]
- Goldmuntz E. 22q11.2 deletion syndrome and congenital heart disease. Am J Med Genet C Semin Med Genet 2020;184:64-72. [Crossref] [PubMed]
- Improved national prevalence estimates for 18 selected major birth defects--United States, 1999-2001. MMWR Morb Mortal Wkly Rep 2006;54:1301-5.
- Gioli-Pereira L, Pereira AC, Mesquita SM, et al. NKX2.5 mutations in patients with non-syndromic congenital heart disease. Int J Cardiol 2010;138:261-5. [Crossref] [PubMed]
- Yoshida A, Morisaki H, Nakaji M, et al. Genetic mutation analysis in Japanese patients with non-syndromic congenital heart disease. J Hum Genet 2016;61:157-62. [Crossref] [PubMed]
- Reuter MS, Chaturvedi RR, Jobling RK, et al. Clinical Genetic Risk Variants Inform a Functional Protein Interaction Network for Tetralogy of Fallot. Circ Genom Precis Med 2021;14:e003410. [Crossref] [PubMed]
- Liu X, Lu S, Shao Y, et al. Disorders of gut microbiota in children with Tetralogy of Fallot. Transl Pediatr 2022;11:385-95. [Crossref] [PubMed]
- Pokorski RJ. Long-term survival after repair of tetralogy of Fallot. J Insur Med 2000;32:89-92.
- Chang RK, Gurvitz M, Rodriguez S. Missed diagnosis of critical congenital heart disease. Arch Pediatr Adolesc Med 2008;162:969-74. [Crossref] [PubMed]
- Peck D, Tretter J, Possner M, et al. Timing of Repair in Tetralogy of Fallot: Effects on Outcomes and Myocardial Health. Cardiol Rev 2021;29:62-7. [Crossref] [PubMed]
- Harold JG. Cardiology patient page. Screening for critical congenital heart disease in newborns. Circulation 2014;130:e79-81. [Crossref] [PubMed]
- Hosseinpour AR, González-Calle A, Adsuar-Gómez A, et al. The Predicament of Surgical Correction of Tetralogy of Fallot. Pediatr Cardiol 2021;42:1252-7. [Crossref] [PubMed]
- Goldstein BH, Petit CJ, Qureshi AM, et al. Comparison of Management Strategies for Neonates With Symptomatic Tetralogy of Fallot. J Am Coll Cardiol 2021;77:1093-106. [Crossref] [PubMed]
- Blue GM, Kirk EP, Giannoulatou E, et al. Advances in the Genetics of Congenital Heart Disease: A Clinician's Guide. J Am Coll Cardiol 2017;69:859-70. [Crossref] [PubMed]
- Gioli-Pereira L, Pereira AC, Bergara D, et al. Frequency of 22q11.2 microdeletion in sporadic non-syndromic tetralogy of Fallot cases. Int J Cardiol 2008;126:374-8. [Crossref] [PubMed]
- Griffin HR, Töpf A, Glen E, et al. Systematic survey of variants in TBX1 in non-syndromic tetralogy of Fallot identifies a novel 57 base pair deletion that reduces transcriptional activity but finds no evidence for association with common variants. Heart 2010;96:1651-5. [Crossref] [PubMed]
- Palomino Doza J, Topf A, Bentham J, et al. Low-frequency intermediate penetrance variants in the ROCK1 gene predispose to Tetralogy of Fallot. BMC Genet 2013;14:57. [Crossref] [PubMed]
- Soemedi R, Topf A, Wilson IJ, et al. Phenotype-specific effect of chromosome 1q21.1 rearrangements and GJA5 duplications in 2436 congenital heart disease patients and 6760 controls. Hum Mol Genet 2012;21:1513-20. [Crossref] [PubMed]
- Goodship JA, Hall D, Topf A, et al. A common variant in the PTPN11 gene contributes to the risk of tetralogy of Fallot. Circ Cardiovasc Genet 2012;5:287-92. [Crossref] [PubMed]
- Zaidi S, Choi M, Wakimoto H, et al. De novo mutations in histone-modifying genes in congenital heart disease. Nature 2013;498:220-3. [Crossref] [PubMed]
- Cordell HJ, Bentham J, Topf A, et al. Genome-wide association study of multiple congenital heart disease phenotypes identifies a susceptibility locus for atrial septal defect at chromosome 4p16. Nat Genet 2013;45:822-4. [Crossref] [PubMed]
- Ekure EN, Adeyemo A, Liu H, et al. Exome Sequencing and Congenital Heart Disease in Sub-Saharan Africa. Circ Genom Precis Med 2021;14:e003108. [Crossref] [PubMed]
- Richards S, Aziz N, Bale S, et al. Standards and guidelines for the interpretation of sequence variants: a joint consensus recommendation of the American College of Medical Genetics and Genomics and the Association for Molecular Pathology. Genet Med 2015;17:405-24. [Crossref] [PubMed]
- Yang Y, Muzny DM, Reid JG, et al. Clinical whole-exome sequencing for the diagnosis of mendelian disorders. N Engl J Med 2013;369:1502-11. [Crossref] [PubMed]
- Page DJ, Miossec MJ, Williams SG, et al. Whole Exome Sequencing Reveals the Major Genetic Contributors to Nonsyndromic Tetralogy of Fallot. Circ Res 2019;124:553-63. [Crossref] [PubMed]
- Jin SC, Homsy J, Zaidi S, et al. Contribution of rare inherited and de novo variants in 2,871 congenital heart disease probands. Nat Genet 2017;49:1593-601. [Crossref] [PubMed]
- Barnes AM, Cabral WA, Weis M, et al. Absence of FKBP10 in recessive type XI osteogenesis imperfecta leads to diminished collagen cross-linking and reduced collagen deposition in extracellular matrix. Hum Mutat 2012;33:1589-98. [Crossref] [PubMed]
- Alanay Y, Avaygan H, Camacho N, et al. Mutations in the gene encoding the RER protein FKBP65 cause autosomal-recessive osteogenesis imperfecta. Am J Hum Genet 2010;86:551-9. [Crossref] [PubMed]
- Barnes AM, Duncan G, Weis M, et al. Kuskokwim syndrome, a recessive congenital contracture disorder, extends the phenotype of FKBP10 mutations. Hum Mutat 2013;34:1279-88. [Crossref] [PubMed]
- Mori-Yoshimura M, Hayashi YK, Yonemoto N, et al. Nationwide patient registry for GNE myopathy in Japan. Orphanet J Rare Dis 2014;9:150. [Crossref] [PubMed]
- Zhao J, Wang Z, Hong D, et al. Mutational spectrum and clinical features in 35 unrelated mainland Chinese patients with GNE myopathy. J Neurol Sci 2015;354:21-6. [Crossref] [PubMed]
- Malicdan MC, Noguchi S, Hayashi YK, et al. Prophylactic treatment with sialic acid metabolites precludes the development of the myopathic phenotype in the DMRV-hIBM mouse model. Nat Med 2009;15:690-5. [Crossref] [PubMed]
(English Language Editor: L. Huleatt)