The development of prediction model for cuffed tracheal tube size from the middle finger in pediatrics: a concise and feasible approach
Highlight box
Key findings
• Middle finger circumference could predict the appropriate size for the cuffed tracheal tube in pediatrics.
What is known and what is new?
• Previously, middle finger length was established as a predictor for the proper size of uncuffed tracheal tubes in pediatrics.
• This study demonstrates that both middle finger circumference and middle finger length can predict the suitable size for cuffed tracheal tubes in pediatrics, with middle finger circumference showing superior predictive capability.
What is the implication, and what should change now?
• The middle finger circumference has potential for predicting the size of the cuffed tracheal tube in pediatric patients, and further large-scale validation studies are required to confirm its accuracy and reliability.
Introduction
In the field of pediatric anesthesia, the selection of the appropriate size of the tracheal tube is crucial. Given the distinctive morphology and anatomy of the pediatric airway, the use of an ill-fitting tracheal tube can result in serious complications. Opting for a larger tracheal tube size may lead to tissue edema, local ischemia, and even subglottic stenosis, while selecting a smaller size may result in insufficient ventilation, poor end-tidal gas monitoring, and anesthetic gas leakage due to inadequate tracheal seal (1). When intubating with a cuffed tracheal tube, opting for a smaller size enables cuff inflation to achieve seal; however, overinflation carries the potential for higher intracuff pressure and subsequent damage to the tracheal mucosa (2). Multiple intubation attempts resulting from the incorrect selection of tracheal tube size may cause airway edema and potentially trigger hypoxemia (3). Consequently, the development of a precise strategy for the accurate selection of tracheal tube size stands as a crucial prerequisite to ensure the safety of airway management in pediatric patients.
Currently, the prediction of tracheal tube size in pediatric patients predominantly relies on age-based formulas, but it is not always accurate because of the variable rate of child development (4). To enhance the precision of tracheal tube size determination, some growth and developmental indicators have been studied for the prediction of the tracheal tube size, such as height (5) and weight (6). Machine learning has also been introduced to prediction using multiple data, encompassing age, sex, height, weight, body mass index (BMI), BMI classification, ideal BMI, and chest radiographs (7). Additionally, alternative imaging techniques, such as ultrasound, have gradually been incorporated into prediction strategies (8). However, despite their improved accuracy compared to age-based formulas, the complexity of these alternative methods and the requirement for advanced tools present obstacles to their widespread clinical adoption. The fingers could be a simple indicator for the selection of the tracheal tube size. The width of the fifth finger has been proven to be related to the tracheal tube size (9). In a recent study, a simple formula based on middle finger measurements demonstrated significantly superior predictive accuracy for uncuffed tracheal tube size when compared to age-based formulas (10). Nevertheless, cuffed tracheal tubes have been recently increasingly used in clinical practice (9,10). Research has substantiated the benefits of cuffed tracheal tubes, demonstrating enhanced ventilation efficacy, a reduction in the number of intubation attempts, and a decrease in adverse perioperative respiratory events (11).
The association between middle finger length and cuffed tracheal tube size in pediatric patients remains unexplored. More importantly, the association between additional measurements such as middle finger circumference and the cuffed tracheal tube size remains uncertain. Thus, we hypothesized that an association exists between the middle finger measurements and the appropriate size of cuffed tracheal tubes which has the potential to serve as a simple predictive indicator. This study aimed to explore the relationship between optimal cuffed tracheal tube size with the length and circumference of the middle finger and to develop a linear model for predicting the appropriate cuffed tracheal tube size. We present this article in accordance with the TRIPOD reporting checklist (available at https://tp.amegroups.com/article/view/10.21037/tp-23-502/rc).
Methods
Patients
This prospective study was conducted between April 2023 and July 2023 after obtaining approval from the Ethics Committee of Shanghai Ninth People’s Hospital (No. SH9H-2022-T414-1). This trial was registered at the Chinese Clinical Trial Registry (www.chictr.org.cn) (trial registration number ChiCTR2300069902). The study was conducted in accordance with the Declaration of Helsinki (as revised in 2013).
This prospective study was conducted at a single center, specifically, at Shanghai Ninth People’s Hospital. The inclusion criteria for the study were children aged between 0–12 years [American Society of Anesthesiologists Physical Status (ASA-PS) of I–III] scheduled for elective surgery with tracheal intubation. The exclusion criteria were as follows: the child’s parents have speech communication and cooperation difficulties; patients with open trauma to the head and neck; patients with cervical fractures, neck surgery, or a history of cervical spine disease; emergency surgery; patients who are allergic to relevant medications. Informed consent was taken from all the patients’ guardians.
Induction of anesthesia
The patients were intravenously anesthetized with a combination of midazolam (0.1 mg/kg), fentanyl (6 µg/kg), propofol (1.5 mg/kg), and rocuronium (0.6 mg/kg). The electrocardiography, noninvasive blood pressure, end-tidal CO2 (ETCO2), and peripheral oxygen saturation (SpO2) were continuously monitored.
After complete muscle relaxation was achieved, an anesthesiologist with over 3 years of experience chose the size of the cuffed tracheal tube (Mallinckrodt™; Lo-Contour Oral/Nasal Tracheal Tube Cuffed, Reinforced; Medtronic, Minneapolis, MN, USA) that they felt was most appropriate for the procedure. The anesthesiologist responsible for the intubation procedure was unaware of the middle finger measurement data during tube size selection.
In cases where resistance was encountered during the insertion of the tracheal tube into the trachea or when a positive airway pressure exceeding 25 cmH2O was required to detect an audible leak, the tube was replaced with an internal diameter (ID) that was 0.5 mm smaller before inflation of the cuff. Cuff inflation was measured (Shiley™ Pressure Control; Covidien, Beyern, Germany) and limited to 25 cmH2O. Mechanical ventilation was initiated with 10 mL/kg tidal volume and a frequency to maintain ETCO2 at 34–38 mmHg. The tube was replaced with the next largest size (0.5 mm larger ID) if a leak around the tube was auscultated at a positive airway pressure <10 cmH2O or peak pressure >25 cmH2O, or a cuff pressure >25 cmH2O was required to seal (12). To determine the leak pressure, the patient was positioned in a supine posture with the head in a neutral position to limit effect on the leak test (13). The need for tracheal tube replacement was recorded. The final size of the tracheal tube recorded was the optimal tracheal tube size.
Study measurements
Demographic data including age, sex, height, weight, and BMI were collected from the patients. The length and circumference of the middle finger were measured using a clean, soft tape measure. The length of the middle finger was measured by determining the distance from the tip of the distal metacarpal to the root of the palm on the palmar aspect. The circumference of the middle finger was measured specifically at the root of the palm. The demonstration of the measurements is shown in Figure 1. To mitigate potential bias, all measurements were performed by the same researcher.
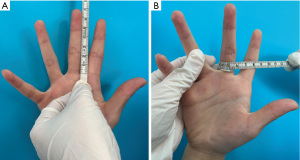
The predicted tracheal tube size according to the age-based formulae recommended for pediatric tracheal tube selection was also recorded. For children under 2 years, Khine formula was used: cuffed tracheal tube ID = [age (years)/4] + 3.0 (14); for children over 2 years, Motoyama formula was used: cuffed tracheal tube ID = [age (years)/4] + 3.5 (15).
Statistical analysis
The primary outcome of this study was to create a reliable formula for selecting the appropriate tracheal tube size with the best-correlated measurement. The measurement data were presented as mean ± standard deviation (SD), whereas the categorical variables were expressed as frequency (%). Linear regression was used to test the association between the length and the circumference of the middle finger (independent variable) and cuffed tracheal tube size (dependent variable). Additionally, age and BMI were included as independent variables in the linear regression analysis. The coefficients of determinations (R2) were calculated which showed the extent to which variation in cuffed tracheal tube size can be accounted for by the independent variable. The mean absolute error (MAE), root mean square error (RMSE), and prediction accuracy were used to evaluate prediction performance. MAE and RMSE were calculated as follows:
Where “yi” represents the actual value, “yp” stands for the predicted value and “n” is the number of observations. The prediction accuracy was the ratio of correct predictions to the total number. A lower MAE and RMSE value typically indicate better performance of an algorithm. A P value <0.05 was used to indicate statistical significance.
We used the method described by Riley et al. to calculate the efficient sample size (16), which is a widely advocated minimal criterion of the sample size to avoid overfitting in regression analysis. Either the length of the middle finger or the circumference of the middle finger functioned as variables. We pre-specified the anticipated R2 (0.8) and used the mean and SD of outcomes in this study sample. A minimum sample size of 235 cases was thus calculated. All data analysis was conducted utilizing the R project software program (R 4.2.2; https://www.r-project.org/).
Results
Baseline characteristics
A total of 272 patients were initially screened. Among them, three patients were excluded because of the loss of measurement information for the middle finger. A further five patients were excluded because of postponed surgery, and three patients were excluded because they underwent a supraglottic airway device. A total of 261 children were included in the final analysis. A flow chart of the study is shown in Figure 2. The baseline characteristics of the study population are presented in Table 1. The mean length of the middle finger was 5.01±1.25 cm, and the mean circumference of the middle finger was 4.36±0.59 cm.
Table 1
Characteristics | Measurement values |
---|---|
Sex | |
Male | 130 (49.8) |
Female | 131 (50.2) |
Age (months) | 46.19±35.83 |
Height (cm) | 107.11±31.31 |
Weight (kg) | 17.80±10.49 |
Middle finger length (cm) | 5.01±1.25 |
Middle finger circumference (cm) | 4.36±0.59 |
Data are shown as n (%) or mean ± standard deviation.
In our cohort, a total of 19 patients had tracheal tubes replaced; the rate of tracheal tube changes was 7.28%. Among these cases, the originally inserted tube size was increased by one size smaller in 11 patients, whereas the inserted tube size was decreased by one size larger in 8 patients.
Regression analysis
The relationship between the median and age and the interquartile range (IQR) of the patients and cuffed tracheal tube size was plotted in Figure 3 which showed no linear relationship, with a distinct turning point occurring at the tube size of 3.5 despite a R2 of 0.78 through linear regression. There was an approximately linear relationship between the mean middle finger circumference and the mean middle finger length with the cuffed tracheal tube size (Figure 4). The R2 in the linear regression between the middle finger circumference and the tracheal tube size was 0.77, indicating that approximately 77% of the variation in the cuffed tracheal tube size can be accounted for by the middle finger circumference (P<0.001). The regression equation calculation can be written as follows:
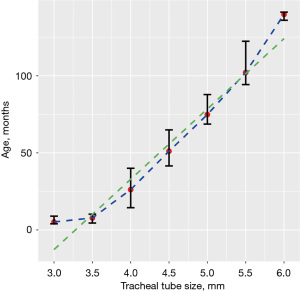
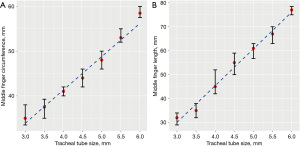
The middle finger length also showed a significant correlation with the tracheal tube size with an R2 value of 0.73 (P<0.001). The regression equation calculation can be written as follows:
When combining the middle finger circumference and middle finger length, the regression equation calculation could be written as follows with an R2 value of 0.83 (P<0.001):
When incorporating the most basic demographic information of age and BMI, the model did not show further improvement, with an R² value of 0.82 (P<0.001). The regression equation can be expressed as follows:
The predictive performance of the four regression equations described above is shown in Table 2. All the regression equations outperformed the conventional age-based formulas, including the Khine formula and the Motoyama formula.
Table 2
Prediction accuracy metrics | Single-variable models | Combination models | ||||
---|---|---|---|---|---|---|
Middle finger circumference | Middle finger length | Age-based formula | Model 1 | Model 2 | ||
MAE | 0.259 | 0.279 | 0.349 | 0.226 | 0.221 | |
RMSE | 0.333 | 0.360 | 0.473 | 0.283 | 0.278 | |
Prediction accuracy (95% CI) |
0.590 (0.530–0.650) |
0.559 (0.499–0.620) |
0.391 (0.332–0.450) |
0.613 (0.554–0.672) |
0.642 (0.576–0.708) |
|
Prediction accuracy within 0.5 mm (95% CI) |
0.966 (0.943–0.988) |
0.962 (0.938–0.985) |
0.923 (0.891–0.956) |
0.989 (0.976–1.000) |
0.985 (0.969–1.000) |
Model 1, middle finger circumference + length. Model 2, middle finger circumference + length + age + body mass index. MAE, mean absolute error; RMSE, root mean square error; CI, confidence interval.
Simplified formula
While the linear regression models combining middle finger circumference, middle finger length, and a model including age and BMI demonstrated superior predictive performance, their complexity presented challenges in terms of practical clinical application. To address this, simplified formulas were proposed, focusing solely on either middle finger circumference or middle finger length.
The formula for predicting the cuffed tracheal tube size by the middle finger circumference can be simplified as below:
The formula for predicting the cuffed tracheal tube size by the middle finger length can be simplified as below:
The predictions based on the simplified formulas were determined through calculations that were rounded to the nearest size of cuffed tracheal tube. The predictive performance based on the simplified formulas is shown in Table 3. The relationship between the tracheal tube size predicted by the simplified formulas we proposed and the actual cuffed tracheal tube size used is shown in Figure 5. The two simplified formulas based on the middle finger measurement data were both superior to the conventional age-based formulas including Khine formula and Motoyama formula. More importantly, the formula based on the middle finger circumference was much simpler and more accurate in our cohort of patients.
Table 3
Formula | MAE | RMSE | Prediction accuracy (95% CI) |
Prediction accuracy within 0.5 mm (95% CI) |
---|---|---|---|---|
Eq. [7] | 0.292 | 0.364 | 0.517 (0.457–0.578) | 0.973 (0.954–0.993) |
Eq. [8] | 0.323 | 0.413 | 0.487 (0.426–0.547) | 0.935 (0.905–0.965) |
MAE, mean absolute error; RMSE, root mean square error; CI, confidence interval; Eq., equation.
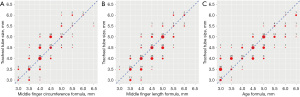
Discussion
In this study, we investigated the association between the middle finger circumference or the middle finger length with the cuffed tracheal tube size. According to the linear regression analysis, we derived two formulas for predicting the optimal tube size. These formulas outperformed the age-based formulas including the Khine formula and Motoyama formula. Notably, the formula based on the middle finger circumference demonstrated superior accuracy and simplicity, making it more suitable for practical application in clinical settings.
Consistent with previous research, our study confirmed that age-based formulas were inadequate in predicting the appropriate cuffed tracheal tube size (17,18). The heterogeneity of children’s growth and development, along with variations in internal organ development, renders a single age indicator insufficient for accurate predictions. Furthermore, our study confirmed a non-linear relationship between age and the cuffed tracheal tube size, further limiting the applicability of age-based formulas. Notably, the age-based formula exhibited a tendency to overestimate the tracheal tube size, particularly at smaller sizes, which was consistent with past studies (1,12). In clinical practice, it is crucial to be cautious of overestimating the tube size, as this can pose a greater risk than opting for a slightly smaller size that may result in laryngotracheal damage.
In recent years, various new tools have emerged for predicting tracheal tube size. A study showed that measurement of the diameter of the trachea at the 7th cervical vertebra (C7) directly on a chest radiograph was a good predictor of the uncuffed tracheal tube size (19). Machine learning techniques are gradually being introduced into predicting both uncuffed and cuffed tracheal tube sizes with simple demographic data (20) and a study has further incorporated data on chest radiographs, including tracheal length from the 6th cervical vertebra (C6) to the carina, tracheal diameter at the level of C7, and tracheal diameter at the level of C6 to achieve good prediction results (7). Additionally, a study utilized 3D printed models of the airway based on computed tomography (CT) images to select the optimal tracheal tube size (21). However, the widespread adoption of these clinical applications has been limited due to concerns related to radiation exposure and the high costs associated with techniques such as CT imaging and 3D printing. Measurement of subglottic diameter on ultrasound images was introduced to improve the prediction accuracy (12,22-25). However, the precision of this method was still not sufficient, and it might be challenging to conduct ultrasound examinations on children in an awake state due to their limited cooperation.
The middle finger can serve as an indicator of growth and development to some extent. The linear relationship between middle finger length and height has been verified (26). Directly measuring the relevant parameters of the middle finger is a simple and non-invasive method that does not require specialized training. In the context of pediatric patients, the length of the middle finger was initially proposed for predicting tracheal intubation depth with cuffed tracheal tubes (27). Subsequently, a previous study confirmed the relationship between middle finger length and uncuffed tracheal tube size (10), and the proposed formula was validated in another study involving a different population group (28). In our study, we confirmed the linear relationship between middle finger length and cuffed tracheal tube size and proposed a new formula that can be used for predicting the size of cuffed tracheal tubes.
Surprisingly, our study revealed that the circumference of the middle finger outperformed the length of the middle finger in accurately predicting the size of cuffed tracheal tubes based on linear regression. Furthermore, when utilizing the formula derived from the regression coefficients, the predictive performance of the middle finger circumference was also superior to that of the middle finger length. Considering the simplicity of the formula based on the middle finger circumference, we recommend its use for predicting the size of cuffed tracheal tubes in clinical practice.
Our study had some limitations. Firstly, to ensure consistency, we used only one kind of tracheal tube, which might limit the applicability of the proposed formulas to other brands. Further validation is required to assess their suitability for use with different tracheal tube brands. Secondly, the formulas we proposed were applied only to cuffed tracheal tubes, and the association between middle finger measurements, especially the circumference of the middle finger and uncuffed tracheal tube size require further study. Lastly, our study was conducted at a single center and the sample size was limited to Chinese children. Consequently, further researches are needed to assess the applicability of the proposed formulas across diverse patient populations and clinical settings.
Conclusions
Our study demonstrated that middle finger circumference and length were correlated with the cuffed tracheal tube size. We have proposed two simplified formulas based on these measurements, which showed superior performance compared to the conventional age-based formula. Among them, the formula utilizing middle finger circumference is recommended for selecting cuffed tracheal tubes in children, as Eq. [7].
Acknowledgments
Funding: This study was supported by
Footnote
Reporting Checklist: The authors have completed the TRIPOD reporting checklist. Available at https://tp.amegroups.com/article/view/10.21037/tp-23-502/rc
Data Sharing Statement: Available at https://tp.amegroups.com/article/view/10.21037/tp-23-502/dss
Peer Review File: Available at https://tp.amegroups.com/article/view/10.21037/tp-23-502/prf
Conflicts of Interest: All authors have completed the ICMJE uniform disclosure form (available at https://tp.amegroups.com/article/view/10.21037/tp-23-502/coif). T.E. received grants or contracts from National Institutes of Health (NIH). The other authors have no conflicts of interest to declare.
Ethical Statement: The authors are accountable for all aspects of the work in ensuring that questions related to the accuracy or integrity of any part of the work are appropriately investigated and resolved. The study was conducted in accordance with the Declaration of Helsinki (as revised in 2013). The study was approved by Ethics Committee of Shanghai Ninth People’s Hospital (No. SH9H-2022-T414-1) and informed consent was taken from all the patients’ guardians.
Open Access Statement: This is an Open Access article distributed in accordance with the Creative Commons Attribution-NonCommercial-NoDerivs 4.0 International License (CC BY-NC-ND 4.0), which permits the non-commercial replication and distribution of the article with the strict proviso that no changes or edits are made and the original work is properly cited (including links to both the formal publication through the relevant DOI and the license). See: https://creativecommons.org/licenses/by-nc-nd/4.0/.
References
- Shibasaki M, Nakajima Y, Ishii S, et al. Prediction of pediatric endotracheal tube size by ultrasonography. Anesthesiology 2010;113:819-24. [Crossref] [PubMed]
- Krishna SG, Hakim M, Sebastian R, et al. Cuffed endotracheal tubes in children: the effect of the size of the cuffed endotracheal tube on intracuff pressure. Paediatr Anaesth 2017;27:494-500. [Crossref] [PubMed]
- Oshodi A, Dysart K, Cook A, et al. Airway injury resulting from repeated endotracheal intubation: Possible prevention strategies. Pediatr Crit Care Med 2011;12:e34-9. [Crossref] [PubMed]
- Oofuvong M, Nuanjun K, Sangkaew T, et al. Prediction of uncuffed tracheal tube sizes in children and infants using age/weight-based formulae: A retrospective cross-sectional study. Int J Pediatr Otorhinolaryngol 2019;122:105-10. [Crossref] [PubMed]
- Luten RC, Wears RL, Broselow J, et al. Length-based endotracheal tube and emergency equipment in pediatrics. Ann Emerg Med 1992;21:900-4. [Crossref] [PubMed]
- Eipe N, Barrowman N, Writer H, et al. A weight-based formula for tracheal tube size in children. Paediatr Anaesth 2009;19:343-8. [Crossref] [PubMed]
- Zhou M, Xu WY, Xu S, et al. Prediction of endotracheal tube size in pediatric patients: Development and validation of machine learning models. Front Pediatr 2022;10:970646. [Crossref] [PubMed]
- Park S, Shin SW, Kim HJ, et al. Choice of the correct size of endotracheal tube in pediatric patients. Anesth Pain Med (Seoul) 2022;17:352-60. [Crossref] [PubMed]
- King BR, Baker MD, Braitman LE, et al. Endotracheal tube selection in children: a comparison of four methods. Ann Emerg Med 1993;22:530-4. [Crossref] [PubMed]
- Ritchie-McLean S, Ferrier V, Clevenger B, et al. Using middle finger length to determine the internal diameter of uncuffed tracheal tubes in paediatrics. Anaesthesia 2018;73:1207-13. [Crossref] [PubMed]
- Chambers NA, Ramgolam A, Sommerfield D, et al. Cuffed vs. uncuffed tracheal tubes in children: a randomised controlled trial comparing leak, tidal volume and complications. Anaesthesia 2018;73:160-8. [Crossref] [PubMed]
- Altun D, Orhan-Sungur M, Ali A, et al. The role of ultrasound in appropriate endotracheal tube size selection in pediatric patients. Paediatr Anaesth 2017;27:1015-20. [Crossref] [PubMed]
- Schwartz RE, Stayer SA, Pasquariello CA. Tracheal tube leak test--is there inter-observer agreement? Can J Anaesth 1993;40:1049-52. [Crossref] [PubMed]
- Khine HH, Corddry DH, Kettrick RG, et al. Comparison of cuffed and uncuffed endotracheal tubes in young children during general anesthesia. Anesthesiology 1997;86:627-31; discussion 27A. [Crossref] [PubMed]
- Motoyama EK, Davis PJ. Smith’s anesthesia for infants and children. 7th edition. Mosby; 2006.
- Riley RD, Ensor J, Snell KIE, et al. Calculating the sample size required for developing a clinical prediction model. BMJ 2020;368:m441. [Crossref] [PubMed]
- Duracher C, Schmautz E, Martinon C, et al. Evaluation of cuffed tracheal tube size predicted using the Khine formula in children. Paediatr Anaesth 2008;18:113-8. [Crossref] [PubMed]
- Manimalethu R, Krishna S, Shafy SZ, et al. Choosing endotracheal tube size in children: Which formula is best? Int J Pediatr Otorhinolaryngol 2020;134:110016. [Crossref] [PubMed]
- Park HP, Hwang JW, Lee JH, et al. Predicting the appropriate uncuffed endotracheal tube size for children: a radiograph-based formula versus two age-based formulas. J Clin Anesth 2013;25:384-7. [Crossref] [PubMed]
- Kim H, Yoon H-K, Lee H, et al. Predicting optimal endotracheal tracheal tube size and depth in pediatric patients using demographic data and machine learning techniques. Korean J Anesthesiol 2023;540-9. [Crossref] [PubMed]
- Park S, Ahn J, Yoon SU, et al. Prediction of endotracheal tube size using a printed three-dimensional airway model in pediatric patients with congenital heart disease: a prospective, single-center, single-group study. Korean J Anesthesiol 2021;74:333-41. [Crossref] [PubMed]
- Kim EJ, Kim SY, Kim WO, et al. Ultrasound measurement of subglottic diameter and an empirical formula for proper endotracheal tube fitting in children. Acta Anaesthesiol Scand 2013;57:1124-30. [Crossref] [PubMed]
- Bharathi BM, Somayaji S, Tulasi T, et al. Prediction of Endotracheal Tube Size in Pediatric Population Using Ultrasonographic Subglottic Diameter and Age-Related Formulas: A Comparative Study. Anesth Essays Res 2022;16:1-6. [Crossref] [PubMed]
- Gupta B, Ahluwalia P. Prediction of endotracheal tube size in the pediatric age group by ultrasound: A systematic review and meta-analysis. J Anaesthesiol Clin Pharmacol 2022;38:371-83. [Crossref] [PubMed]
- Parpucu UM, Şahin N. The Effect of the Ultrasonography Measurement Method and the Conventional Measurement Method Used in Endotracheal Tube Size Selection in the Pediatric Patient Group on Postextubation Complications and Patient Recovery. Cureus 2023;15:e46184. [Crossref] [PubMed]
- Rastogi P, Kanchan T, Menezes RG, et al. Middle finger length--a predictor of stature in the Indian population. Med Sci Law 2009;49:123-6. [Crossref] [PubMed]
- Zhou QH, Xiao WP, Zhou HM. Middle finger length-based tracheal intubation depth improves the rate of appropriate tube placement in children. Paediatr Anaesth 2015;25:1132-8. [Crossref] [PubMed]
- Saikia P, Thottan RS. Length of the middle finger of hand as a simple and reliable predictor of optimal size of uncuffed endotracheal tube in paediatric patients: An observational study. Indian J Anaesth 2021;65:813-9. [Crossref] [PubMed]