Robotic-assisted laparoscopy in pediatric surgical oncology: a narrative review
Introduction
The use of robotic surgery has spread in the pediatric population as precision and stability offered by the technology allow for minimally invasive treatment of more and more complex cases (1). Surgical oncology in children encompasses a wide range of surgical morbidity and difficulty, from simple organ removal to large tumors with vascular involvement and adjacent-organ invasion. All these procedures are to follow the oncologic principles to ensure the best possible oncologic outcome, keeping in mind that our patients have a long life ahead of them. Managing these cases calls for experienced surgeons in minimally invasive surgery (MIS) and a solid oncological background (2).
The aim of this review is to analyze the current state of robotic-assisted laparoscopy in pediatric tumor resection, show the pitfalls in establishing clear guidelines in minimally invasive surgical oncology and describe future developments of the robotic technology. We present this article in accordance with the Narrative Review reporting checklist (available at https://tp.amegroups.com/article/view/10.21037/tp-23-251/rc).
Methods
A literature search of the MEDLINE/PubMed database was conducted, using the terms “robotic surgery”, “pediatric” or “children” and “oncology” or “tumor”. All relevant English-language studies published between 2008 and 2022 were retrieved. All types of studies regarding thoracic, abdominal or oral robotic surgery were sought, including case reports. Literature reviews, meta-analyses or studies with patients’ overlap were excluded.
The search strategy summary is shown in Table 1.
Table 1
Items | Specification |
---|---|
Date of search | May 21st, 2022 |
Databases and other sources searched | MEDLINE/PubMed |
Search terms used | Robotic surgery, pediatric/children, oncology/tumor |
Timeframe | 2008 to 2022 |
Inclusion and exclusion criteria | English-language studies included. Reviews, meta-analyses and studies with patients’ overlap excluded; neurosurgery, orthopedics, ophthalmology and cardiac surgery excluded. |
Selection process | Performed by N.V. (author) |
Robotic surgical oncology in children
The difficulties in establishing eligibility criteria for robotic resection of pediatric tumors lie in the great variability of indications, heterogeneity in tumor histology with their own surgical specificities, and wide range of age and weight (3,4). The literature search we conducted on robotic-assisted surgical oncology (Figure 1) yielded a total of 31 studies reporting 171 cases between 2008 and 2022, including 21 (68%) case reports (Table 2). Only three studies counted ten patients or more. The five most reported procedures were partial or radical adrenalectomy (41 cases), partial or radical nephrectomy (30 cases), anterior or posterior mediastinal mass excision (17 cases), partial or radical ovariectomy (15 cases) and retroperitoneal lymph node dissection (14 cases).
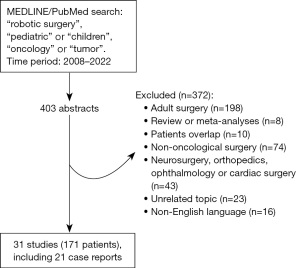
Table 2
Authors | Year | Surgical procedure | Tumor pathology | Total # cases (N=171) | # cases (N=171) | Age at surgery (years) | Conversion rate | Complications |
---|---|---|---|---|---|---|---|---|
Meehan et al. | 2008 | Mediastinal mass excision | Neuroblastic tumor | 5 | 2 | 9.8 [2–17] | 0 | 0 |
Mature teratoma | 1 | |||||||
Germ cell tumor | 1 | |||||||
Inflammatory mass of unclear etiology | 1 | |||||||
Rogers et al. | 2008 | Partial adrenalectomy | Pheochromocytoma | 1 | 1 | 14 | 0 | 0 |
Meehan et al. | 2008 | Radical ovariectomy | Mature teratoma | 10 | 1 | NA | 40% (4/10) | Incomplete resection (neuroblastoma) |
Partial ovariectomy | NA | 1 | ||||||
Unilateral adrenalectomy | Neuroblastoma | 6 | ||||||
Pheochromocytoma | ||||||||
Resection of pancreatic tumor | NA | 1 | ||||||
Retroperitoneal tumor resection | Ganglioneuroma | 1 | ||||||
Cost et al. | 2012 | RPLND | Paratesticular embryonal rhabdomyosarcoma | 2 | 1 | 15 | 0 | 0 |
Malignant non-seminomatous germ-cell tumor | 1 | |||||||
Cost et al. | 2012 | Partial nephrectomy | Clear cell renal carcinoma | 1 | 1 | 14 | 0 | 0 |
Kokot et al. | 2013 | TORS | Synovial sarcoma | 1 | 1 | 15 | 0 | 0 |
Cost et al. | 2015 | Radical nephrectomy | Wilms tumor (prechemotherapy) | 1 | 1 | 14 | 0 | 0 |
Glaser et al. | 2017 | RPLND | Non-seminomatous germ cell tumor | 2 | 1 | 15 and 17 | 0 | 0 |
Ectomesenchymoma with a spindle-cell rhabdomyosarcoma component | 1 | |||||||
Hu et al. | 2017 | Distal pancreatectomy | Insulinoma | 1 | 1 | 9 | 0 | 0 |
Yadav et al. | 2018 | Partial nephrectomy | Wilms tumor | 1 | 1 | 1.5 | 0 | Lymphorrhea |
Agarwal et al. | 2018 | Radical prostatectomy | Embryonal rhabdomyosarcoma | 1 | 1 | 7 | 0 | 0 |
Hagendoorn et al. | 2018 | Pancreatoduodenectomy | Solid pseudopapillary tumor | 1 | 1 | 10 | 0 | Delayed gastric emptying |
Arnold et al. | 2018 | TORS | Neurofibroma | 1 | 1 | 6 | 0 | Incomplete resection |
Liang et al. | 2018 | Pancreatic enucleation | Insulinoma | 1 | 1 | 9 | 0 | 0 |
Varda et al. | 2018 | Partial nephrectomy | Papillary renal cell carcinoma | 4 | 1 | 3–17 | 0 | 0 |
Unilateral adrenalectomy | Ganglioneuroma | 1 | ||||||
RPLND | Paratesticular rhabdomyosarcoma | 2 | ||||||
Non-seminomatous germ-cell tumor | ||||||||
Lalli et al. | 2019 | Distal pancreatectomy | Solid pseudopapillary tumor | 1 | 1 | 17 | 0 | 0 |
Llorens de Knecht et al. | 2019 | Radical prostatectomy | Primitive prostatic carcinoid tumor | 1 | 1 | 3 | 0 | Two febrile episodes, death due to local recurrence and bone metastasis |
Xie et al. | 2019 | Ovarian tumorectomy | Mature teratoma | 4 | 3 | 7.5 [1–13] | 0 | 0 |
Mucinous cystadenoma | 1 | |||||||
Navarrete Arellano et al. | 2019 | Unilateral adrenalectomy | Pheochromocytoma | 4 | 1 | 4.8 (average) | 25% (1/4) | 0 |
Radical nephrectomy | NA | 1 | ||||||
Mediastinal mass excision | Mature teratoma | 1 | ||||||
Retroperitoneal mass excision | Lipoma | 1 | ||||||
Canevari et al. | 2020 | TORS | Base-tongue Ewing sarcoma | 1 | 1 | 16 | 0 | 0 |
Brown et al. | 2020 | RPLND | Testicular or paratesticular cancer (NA) | 4 | 4 | NA | NA | NA |
Mitra et al. | 2020 | Unilateral adrenalectomy | Ganglioneuroblastoma | 3 | 2 | 2–13 | 0 | 0 |
Pheochromocytoma | 1 | |||||||
Silveri et al. | 2020 | Partial nephrectomy | Perivascular epithelioid cell tumor | 1 | 1 | 14 | 0 | 0 |
Nishio et al. | 2020 | Radical cystectomy | Embryonal rhabdomyosarcoma | 1 | 1 | 6 | 0 | 0 |
Nota et al. | 2021 | Distal pancreatectomy | Neuroendocrine tumor | 1 | 1 | 11 | 0 | 0 |
van Ramshorst et al. | 2021 | Central pancreatectomy | Solid pseudopapillary tumor | 1 | 1 | 16 | 0 | Grade B pancreatic fistula |
Schulte Am Esch et al. | 2021 | Pancreatic enucleation | Insulinoma | 1 | 1 | 10 | 0 | Pancreatic pseudocyst |
Lowrey et al. | 2021 | Unilateral adrenalectomy | Adrenocortical tumor | 1 | 1 | 4 | 0 | 0 |
Li et al. | 2021 | Partial or radical cystectomy | Bladder/prostate rhabdomyosarcoma | 8 | 8 | NA | NA | NA |
Vatta et al. | 2022 | Radical ovariectomy | Mature teratoma | 13 | 4 | 13.2 [8–16.9] | 0 | 0 |
Partial ovariectomy | Mature teratoma | 5 | ||||||
Serous cystadenoma | ||||||||
Mucinous cystadenoma | ||||||||
Seromucinous cystadenoma | ||||||||
Pelvic mass excision | Serous papillary cystadenofibroma of the fallopian tube | 2 | ||||||
Mediastinal mass excision | Ganglioneuroblastoma | 1 | ||||||
Perirenal mass excision | Neuroblastoma recurrence | 1 | ||||||
Blanc et al. | 2022 | Unilateral adrenalectomy | Neuroblastic tumor | 93 | 18 | 8.2 [3.6–13] | 8% (7/93) | Pneumothorax (n=2), postoperative collection (n=1), anastomotic stenosis (n=1), bowel adhesion (n=1) |
Pheochromocytoma | ||||||||
Adrenocortical adenoma | ||||||||
Bilateral adrenalectomy | McCune Albright | 5 | ||||||
Carney complex | ||||||||
Pheochromocytoma | ||||||||
Radical nephrectomy | Wilms tumor | 17 | ||||||
Undifferentiated renal sarcoma | ||||||||
Radical nephrectomy with caudal splenopancreatectomy | Wilms tumor | 1 | ||||||
Partial nephrectomy | Wilms tumor | 6 | ||||||
Tubular papillary carcinoma | ||||||||
Metanephric adenoma | ||||||||
Nephrogenic rest | ||||||||
Central pancreatectomy | Focal congenital hyperinsulinism | 3 | ||||||
Somatostatinoma | ||||||||
Distal pancreatectomy | Focal congenital hyperinsulinism | 1 | ||||||
Mediastinal mass excision | Neuroblastic tumor | 10 | ||||||
Mature teratoma | ||||||||
Thymectomy | Thymoma | 4 | ||||||
Myasthenia | ||||||||
Prophylactic thymectomy for MEN1 | ||||||||
Partial gastrectomy | Inflammatory myofibroblastic tumor | 1 | ||||||
Radical gastrectomy (totalization) | Inflammatory myofibroblastic tumor | 1 | ||||||
Pulmonary lobectomy | Bronchial carcinoid tumor | 1 | ||||||
Pelvic mass excision | Lipoma | 9 | ||||||
Embryonal rhabdomyosarcoma | ||||||||
Neurofibroma | ||||||||
Inflammatory myofibroblastic tumor | ||||||||
Mature teratoma | ||||||||
Germ cell tumor | ||||||||
Neuroblastic tumor | ||||||||
Paraganglioma | ||||||||
Omentectomy | Inflammatory myofibroblastic tumor | 1 | ||||||
Mesenteric mass excision | Leiomyoma | 1 | ||||||
RPLND | Seminomatous or Non-seminomatous germ cell tumor | 4 | ||||||
Retroperitoneal tumor resection | Neuroblastic tumor | 10 | ||||||
Paraganglioma |
In the "Age at surgery" column, data are presented as median [range], number, or range unless otherwise specified. NA, not available; RPLND, retroperitoneal lymph-node dissection; TORS, transoral robotic surgery; MEN1, multiple endocrine neoplasia type 1.
The scarcity and great diversity of pediatric tumors are a serious impediment in building the large series needed to establish robotic surgery oncologic guidelines.
Initially intended for damage control surgery on the battlefield, robotic surgery was first reported in 1998 by Himpens et al. (5). Meininger et al. (6) were then the first to borrow this adult-designed surgical technology and use it in children in 2001. Seven years later, robotic surgery was first used for pediatric tumor resection (7).
Contrary to adult surgery, the development of a new technology in children cannot wait for randomized control trials. This was the case with laparoscopy and robotic surgery is no exception. Potential benefits and pitfalls are inferred from retrospective studies (8). Pediatric robotic-assisted surgical oncology has spread without ironclad proof of safety and effectiveness (9), hence the initial skepticism that surrounded its initial use.
The use of MIS in surgical oncology has long been debated. The main concern was the risk of incomplete resection and recurrence (10,11). When performing such procedures, abiding by the oncologic principles is paramount: avoid tumor spillage, en-bloc macroscopically complete resection, optimal lymph node resection and preserving adjacent organs (3,12,13). MIS does not worsen the oncologic outcome as long as the above-mentioned principles are respected, as shown by Bouty et al. (14) or Blanc et al. (15) for the resection of Wilms tumor using laparoscopy or robotic-assisted surgery, respectively. Furthermore, MIS is associated with a shorter time to adjuvant chemotherapy in large adult series (16,17) and might, therefore, improve the oncologic outcome in selected cases.
Several other concerns have been addressed in the literature: the greater risk of port-site tumor recurrence, which has been disproven by adult series (18,19); the lack of haptic feedback as a possible cause of tumor rupture, which has been replaced by an enhanced visualization of the tension applied to the tissue and limited tumor manipulation (20); and the need for a larger scar to extract the tumor since the latter can be concealed and reduced to the minimum to allow safe extraction (21,22).
While the benefits of MIS on postoperative outcome are being reported in children (23,24), they have been established for robotic surgery in adult studies. For adult pancreatic resection, MIS has been shown to decrease intraoperative blood loss and postoperative complications, reduce time to oral intake and length of hospital stay, without any difference on mortality and reoperation rate compared to an open approach in a meta-analysis by Nigri et al. (25). Robotic pancreatic resection has also been shown to decrease the conversion rate compared to laparoscopy in adults with benign or malignant lesions of the distal pancreas (26), decrease the rate of postoperative fistula compared to open Whipple’s procedure in adults (27) and significantly improve spleen preservation in pancreatic caudal resections compared to laparoscopy in adult benign or malignant pancreatic lesions (28). A randomized control trial on robotic versus laparoscopic oncologic gastrectomy in adults has also yielded great results with a higher Clavien ≥II complication rate in the laparoscopic group, no difference regarding the number of retrieved lymph nodes or surgical curability and an improved postoperative recovery in the robotic group (29). A comparative study on robotic versus open thymectomy in adult myasthenia and thymoma has shown a significant decrease of postoperative pain and hospital stay without any difference in operative time and postoperative complications (30). In retroperitoneal lymph node dissection, a large cohort study of testicular and para-testicular cancer, including pediatric patients, reported a significantly shorter length of hospital stay using the robotic technology compared to a non-robotic approach (31). MIS retroperitoneal lymph node dissection is also associated with a lower rate of retrograde ejaculation and bowel complication compared to an open approach, although the risk of vascular injury and chylous ascites was greater (32).
Vascular involvement remains one the greatest challenges of minimally invasive pediatric surgical oncology (15). Surgical feasibility should be assessed based on tumor characteristics, preoperative imaging possibly with tridimensional reconstruction and surgeon’s experience until clear guidelines are issued.
The growing number of cases entails the development of surgical guidelines for robotic surgery, similar to the use of image-defined risk factors in the laparoscopic treatment of neuroblastic tumors (33,34). Robot-specific guidelines are required as robotic technology has pushed the boundaries of laparoscopic surgery in terms of surgical complexity (3). Robotic surgery is closer to open surgery than laparoscopy with a less steep learning curve, allowing for the resection of larger and more complex tumors than laparoscopy (7,15). The substantial experience of Blanc et al. (3) in robotic surgical oncology led to a primary set of guidelines in patient selection based on tumor location and pathology, as shown in Table 3.
Table 3
Tumor type | Robotic surgery may be considered | Relative contraindications | Formal contraindications |
---|---|---|---|
Thoracic tumors | Paravertebral neuroblastoma | Age <2 years old (limited access) | Encasement of vessel |
Tumor limited to the thymic bed (teratoma, thymoma) | Mediastinal extension (pericardium, esophagus, trachea) | ||
Lung resection (single metastasis) | |||
Renal tumors | Tumor not crossing the ipsilateral border of the spine AND | Tumor with a thin rim of normal parenchyma | Tumor crossing the midline |
Tumor with a thick rim of normal parenchyma AND | Tumor crossing the ipsilateral border of the spine (but not the midline) | Tumor infiltrating extrarenal structures (liver, diaphragm) | |
Tumor without any sign of infiltration of extrarenal structures AND | Tumor with encasement of renal vessels (e.g., sarcomas, carcinomas) | ||
ETV/EPBV <1.5% | ETV/EPBV 1.5–2% | ETV/EPBV >2% | |
Neuroblastic tumors | Paravertebral (thoracic, abdominal or pelvic) neuroblastoma without foramen extension | One or two IDRFs | >2 IDRFs |
Adrenal tumor | Paravertebral neuroblastoma with foramen extension but without spinal component | Any IDRF number involving median vessels and/or both renal pedicles | |
Neuroblastoma of the Zuckerkandl ganglia | Paravertebral neuroblastoma with foraminal and intraspinal extension | ||
ETV/EPBV >1% AND | ETV/EPBV 1–2% | ETV/EPBV >2% | |
No IDRF | |||
Paragangliomas/pheochromocytomas | Encasement of major vessels | ||
Adrenocortical carcinomas | All adrenocortical carcinomas | ||
Solid pseudopapillary neoplasms | All solid pseudopapillary tumors |
ETV/EPBV, ellipsoid tumor volume (0.52 × width × length × height, mm3) over estimated patient blood volume (75 mL/kg for children >3 months); IDRF, image-defined risk factors.
The widespread use of robotic surgery paved the way for future technologic advancements. Current research in pediatric surgical oncology focuses on intraoperative locoregional treatment, improved vision with fluorescence and dyed-loaded specific probes and the many possibilities of enhancement software using the robotic console (35). A case of pediatric pancreatic enucleation using a robotic ultrasound probe with dual visual and ultrasound images integrated in the robotic console was recently reported (36). Tridimensional image overlay can be implemented in the console to guide the surgeon’s hand (37). Such an approach has proven beneficial for patients with a decreased complications rate, better resection margins and preserved renal function in a multicentric study on partial nephrectomy in adult renal cancer (38). Several papers have reported the use of Indocyanine green in tumor resection using FireflyÒ (Intuitive Surgical, Sunnyvale, CA, USA), the dedicated robotic interface for near-infrared-fluorescence-guided surgery, both in pediatric and adult surgery (39-41). Robotic gamma detection probes are also being explored for radio-guided surgery as described by Martelli et al. who used radiopharmaceuticals for the resection of neuroblastic tumors (42).
Andras et al. (43) have reviewed possible applications of integrated artificial intelligence in robotic surgery: as an assessment tool for surgical skills in surgical training, to predict operative time and postoperative outcome based on clinical and intraoperative data, to replace haptic feedback with a suture breakage warning system, or using enhanced reality as surgical guidance. Autonomous robotic surgery with human supervision could be a future prospect as the use of such a technology has already been described on human cadavers (44). With continued miniaturization and instrumentation improvements, robotic surgery should be able to overcome its current challenges in the pediatric population, namely in neonatal surgery with the use of 3-mm robotic instruments (45).
Conclusions
The robotic technology may reduce the morbidity of surgical oncology in children and improve the postoperative outcome in terms of pain, recovery, hospital stay and scarring. The oncologic outcome remains the primary goal and robotic-assisted laparoscopy cannot be used at the expense of the oncologic principles. The robotic technology allows the surgeon to push the boundaries of conventional laparoscopy. Specific surgical guidelines are, therefore, necessary to define the indications and contraindications of robotic resection for pediatric tumors. Their heterogeneity and scarcity make it all the more difficult and underline the need to identify expert centers.
Acknowledgments
Funding: None.
Footnote
Provenance and Peer Review: This article was commissioned by the Guest Editor (Ciro Esposito) for the series “Pediatric Robotic Surgery” published in Translational Pediatrics. The article has undergone external peer review.
Reporting Checklist: The authors have completed the Narrative Review reporting checklist. Available at https://tp.amegroups.com/article/view/10.21037/tp-23-251/rc
Peer Review File: Available at https://tp.amegroups.com/article/view/10.21037/tp-23-251/prf
Conflicts of Interest: All authors have completed the ICMJE uniform disclosure form (available at https://tp.amegroups.com/article/view/10.21037/tp-23-251/coif). The series “Pediatric Robotic Surgery” was commissioned by the editorial office without any funding or sponsorship. T.B. is an official proctor in Intuitive Surgical. The authors have no other conflicts of interest to declare.
Ethical Statement:
Open Access Statement: This is an Open Access article distributed in accordance with the Creative Commons Attribution-NonCommercial-NoDerivs 4.0 International License (CC BY-NC-ND 4.0), which permits the non-commercial replication and distribution of the article with the strict proviso that no changes or edits are made and the original work is properly cited (including links to both the formal publication through the relevant DOI and the license). See: https://creativecommons.org/licenses/by-nc-nd/4.0/.
References
- Cundy TP, Shetty K, Clark J, et al. The first decade of robotic surgery in children. J Pediatr Surg 2013;48:858-65. [Crossref] [PubMed]
- Fuchs J, Schafbuch L, Ebinger M, et al. Minimally invasive surgery for pediatric tumors - current state of the art. Front Pediatr 2014;2:48. [Crossref] [PubMed]
- Blanc T, Meignan P, Vinit N, et al. Robotic Surgery in Pediatric Oncology: Lessons Learned from the First 100 Tumors-A Nationwide Experience. Ann Surg Oncol 2022;29:1315-26. Erratum in: Ann Surg Oncol 2021;28:901. [Crossref] [PubMed]
- Galazka P, Czyzewski K, Marjanska A, et al. Minimally Invasive Surgery in Pediatric Oncology: Proposal of Guidelines. Anticancer Res 2019;39:5853-9. [Crossref] [PubMed]
- Himpens J, Leman G, Cadiere GB. Telesurgical laparoscopic cholecystectomy. Surg Endosc 1998;12:1091. [Crossref] [PubMed]
- Meininger DD, Byhahn C, Heller K, et al. Totally endoscopic Nissen fundoplication with a robotic system in a child. Surg Endosc 2001;15:1360. [Crossref] [PubMed]
- Meehan JJ, Sandler A. Pediatric robotic surgery: A single-institutional review of the first 100 consecutive cases. Surg Endosc 2008;22:177-82. [Crossref] [PubMed]
- Meehan JJ. Robotic surgery for pediatric tumors. Cancer J 2013;19:183-8. [Crossref] [PubMed]
- van Dalen EC, de Lijster MS, Leijssen LG, et al. Minimally invasive surgery versus open surgery for the treatment of solid abdominal and thoracic neoplasms in children. Cochrane Database Syst Rev 2015;1:CD008403. [Crossref] [PubMed]
- Cundy TP, Marcus HJ, Clark J, et al. Robot-assisted minimally invasive surgery for pediatric solid tumors: a systematic review of feasibility and current status. Eur J Pediatr Surg 2014;24:127-35. [Crossref] [PubMed]
- Warmann S, Fuchs J, Jesch NK, et al. A prospective study of minimally invasive techniques in pediatric surgical oncology: preliminary report. Med Pediatr Oncol 2003;40:155-7. [Crossref] [PubMed]
- Phelps HM, Lovvorn HN 3rd. Minimally Invasive Surgery in Pediatric Surgical Oncology. Children (Basel) 2018;5:158. [Crossref] [PubMed]
- Christison-Lagay ER, Thomas D. Minimally Invasive Approaches to Pediatric Solid Tumors. Surg Oncol Clin N Am 2019;28:129-46. [Crossref] [PubMed]
- Bouty A, Blanc T, Leclair MD, et al. Minimally invasive surgery for unilateral Wilms tumors: Multicenter retrospective analysis of 50 transperitoneal laparoscopic total nephrectomies. Pediatr Blood Cancer 2020;67:e28212. [Crossref] [PubMed]
- Blanc T, Pio L, Clermidi P, et al. Robotic-assisted laparoscopic management of renal tumors in children: Preliminary results. Pediatr Blood Cancer 2019;66:e27867. [Crossref] [PubMed]
- Mbah N, Agle SC, Philips P, et al. Laparoscopic hepatectomy significantly shortens the time to postoperative chemotherapy in patients undergoing major hepatectomies. Am J Surg 2017;213:1060-4. [Crossref] [PubMed]
- Jung YB, Kang J, Park EJ, et al. Time to Initiation of Adjuvant Chemotherapy in Colon Cancer: Comparison of Open, Laparoscopic, and Robotic Surgery. J Laparoendosc Adv Surg Tech A 2016;26:799-805. [Crossref] [PubMed]
- Qadan M, Kingham TP. Technical Aspects of Gallbladder Cancer Surgery. Surg Clin North Am 2016;96:229-45. [Crossref] [PubMed]
- Mantica G, Smelzo S, Ambrosini F, et al. Port-site metastasis and atypical recurrences after robotic-assisted radical cystectomy (RARC): an updated comprehensive and systematic review of current evidences. J Robot Surg 2020;14:805-12. [Crossref] [PubMed]
- Meehan JJ, Sandler AD. Robotic resection of mediastinal masses in children. J Laparoendosc Adv Surg Tech A 2008;18:114-9. [Crossref] [PubMed]
- Barbosa JA, Barayan G, Gridley CM, et al. Parent and patient perceptions of robotic vs open urological surgery scars in children. J Urol 2013;190:244-50. [Crossref] [PubMed]
- Kinahan KE, Sharp LK, Seidel K, et al. Scarring, disfigurement, and quality of life in long-term survivors of childhood cancer: a report from the Childhood Cancer Survivor study. J Clin Oncol 2012;30:2466-74. [Crossref] [PubMed]
- Varlet F, Petit T, Leclair MD, et al. Laparoscopic treatment of renal cancer in children: a multicentric study and review of oncologic and surgical complications. J Pediatr Urol 2014;10:500-5. [Crossref] [PubMed]
- McKay KG, Abdul Ghani MO, Crane GL, et al. Oncologic Fidelity of Minimally Invasive Surgery to Resect Neoadjuvant-Treated Wilms Tumors. Am Surg 2022;88:943-52. [Crossref] [PubMed]
- Nigri GR, Rosman AS, Petrucciani N, et al. Metaanalysis of trials comparing minimally invasive and open distal pancreatectomies. Surg Endosc 2011;25:1642-51. [Crossref] [PubMed]
- Daouadi M, Zureikat AH, Zenati MS, et al. Robot-assisted minimally invasive distal pancreatectomy is superior to the laparoscopic technique. Ann Surg 2013;257:128-32. [Crossref] [PubMed]
- Vining CC, Kuchta K, Berger Y, et al. Robotic pancreaticoduodenectomy decreases the risk of clinically relevant post-operative pancreatic fistula: a propensity score matched NSQIP analysis. HPB (Oxford) 2021;23:367-78. [Crossref] [PubMed]
- Waters JA, Canal DF, Wiebke EA, et al. Robotic distal pancreatectomy: cost effective? Surgery 2010;148:814-23. [Crossref] [PubMed]
- Ojima T, Nakamura M, Hayata K, et al. Short-term Outcomes of Robotic Gastrectomy vs Laparoscopic Gastrectomy for Patients With Gastric Cancer: A Randomized Clinical Trial. JAMA Surg 2021;156:954-63. [Crossref] [PubMed]
- Luzzi L, Corzani R, Ghisalberti M, et al. Robotic surgery vs. open surgery for thymectomy, a retrospective case-match study. J Robot Surg 2021;15:375-9. [Crossref] [PubMed]
- Brown CT, Sebastião YV, Zann A, et al. Utilization of robotics for retroperitoneal lymph-node dissection in pediatric and non-pediatric hospitals. J Robot Surg 2020;14:865-70. [Crossref] [PubMed]
- Kenney PA, Tuerk IA. Complications of laparoscopic retroperitoneal lymph node dissection in testicular cancer. World J Urol 2008;26:561-9. [Crossref] [PubMed]
- Brisse HJ, McCarville MB, Granata C, et al. Guidelines for imaging and staging of neuroblastic tumors: consensus report from the International Neuroblastoma Risk Group Project. Radiology 2011;261:243-57. [Crossref] [PubMed]
- Irtan S, Brisse HJ, Minard-Colin V, et al. Minimally invasive surgery of neuroblastic tumors in children: Indications depend on anatomical location and image-defined risk factors. Pediatr Blood Cancer 2015;62:257-61. [Crossref] [PubMed]
- Privitera L, Paraboschi I, Cross K, et al. Above and Beyond Robotic Surgery and 3D Modelling in Paediatric Cancer Surgery. Front Pediatr 2021;9:777840. [Crossref] [PubMed]
- Schulte Am Esch J, Krüger M, Barthlen W, et al. Technical aspects of paediatric robotic pancreatic enucleation based on a case of an insulinoma. Int J Med Robot 2021;17:e2317. [Crossref] [PubMed]
- Virzì A, Muller CO, Marret JB, et al. Comprehensive Review of 3D Segmentation Software Tools for MRI Usable for Pelvic Surgery Planning. J Digit Imaging 2020;33:99-110. [Crossref] [PubMed]
- Michiels C, Khene ZE, Prudhomme T, et al. 3D-Image guided robotic-assisted partial nephrectomy: a multi-institutional propensity score-matched analysis (UroCCR study 51). World J Urol 2023;41:303-13. [Crossref] [PubMed]
- Esposito C, Settimi A, Del Conte F, et al. Image-Guided Pediatric Surgery Using Indocyanine Green (ICG) Fluorescence in Laparoscopic and Robotic Surgery. Front Pediatr 2020;8:314. [Crossref] [PubMed]
- Esposito C, Autorino G, Castagnetti M, et al. Robotics and future technical developments in pediatric urology. Semin Pediatr Surg 2021;30:151082. [Crossref] [PubMed]
- Mehdorn AS, Beckmann JH, Braun F, et al. Usability of Indocyanine Green in Robot-Assisted Hepatic Surgery. J Clin Med 2021;10:456. [Crossref] [PubMed]
- Martelli H, Ricard M, Larroquet M, et al. Intraoperative localization of neuroblastoma in children with 123I- or 125I-radiolabeled metaiodobenzylguanidine. Surgery 1998;123:51-7.
- Andras I, Mazzone E, van Leeuwen FWB, et al. Artificial intelligence and robotics: a combination that is changing the operating room. World J Urol 2020;38:2359-66. [Crossref] [PubMed]
- O’Sullivan S, Leonard S, Holzinger A, et al. Anatomy 101 for AI-driven robotics: Explanatory, ethical and legal frameworks for development of cadaveric skills training standards in autonomous robotic surgery/autopsy. Int J Med Robot Comput Assist Surg 2020;16:e2020.
- Krebs TF, Egberts JH, Lorenzen U, et al. Robotic infant surgery with 3 mm instruments: a study in piglets of less than 10 kg body weight. J Robot Surg 2022;16:215-28. [Crossref] [PubMed]