Antimicrobial susceptibility and serotype distribution of Streptococcus pneumoniae isolates among children in Suzhou, China
Highlight box
Key findings
• The main serotypes of Streptococcus pneumoniae (SP) in Suzhou were 19F, 6B, 23F, 6A, and 19A. Strains belonging to different serotypes exhibited distinct antimicrobial resistance patterns and were found to be associated with different diseases. The use of pneumococcal conjugate vaccine (PCV)13 is beneficial to children in Suzhou.
What is known and what is new?
• Most SP isolates showed multidrug-resistant. The most prevalent pneumococcal serotype was 19F.
• PCV13 serotype coverage was 80.9%. Strains belonging to different serotypes exhibited distinct antimicrobial resistance patterns and were found to be associated with different diseases.
What is the implication, and what should change now?
• PCV13 vaccination would be beneficial for children to alleviate suffering from SP-related diseases.
Introduction
Streptococcus pneumoniae (SP)-related diseases have attracted global public health attention due to their high burden of disease and associated mortality. It was the first leading aetiology of lower respiratory infections death among all regions in 2019, accounting for over 50% of deaths (1).
High rates of drug resistance and the spread of multidrug-resistant (MDR) strains of SP constitute serious public health concerns (2,3). Vaccination with pneumococcal conjugate vaccine (PCV) not only reduces the morbidity and mortality of SP infection but also reduces antibiotic resistance and changes the prevalence of SP serotypes (4,5). Angoulvant et al. (4) found that 3 years after the introduction of 13-valent PCV (PCV13; targeting serotypes l, 3, 4, 5, 6A, 6B, 7F, 9V, 14, 18C, 19A, 19F, and 23F), the detection rate of penicillin non-susceptible SP (PNSSP) decreased rapidly from 47.1% to 39% in non-invasive isolates. A recent study demonstrated an increase in non-PCV13 serotypes of isolates from the nasopharynx after the introduction of PCV13 (6).
The 7-valent PCV (PCV7; targeting serotypes 4, 6B, 9V, 14, 18C, 19F, and 23F) was introduced to China in October 2008, but it has not yet been included in the Chinese Expanded Program on Immunizations. PCV13 was licensed for optional use in November 2016, replacing PCV7. The impacts on the serotype prevalence and antimicrobial susceptibility of SP in the Suzhou area are the focus of our attention. This study aimed to determine the SP antimicrobial resistance patterns and serotype distributions of carriage isolates among hospitalized children in the Suzhou area. We present this article in accordance with the STROBE reporting checklist (available at https://tp.amegroups.com/article/view/10.21037/tp-23-547/rc).
Methods
Patients and clinical isolates
The study was conducted at the Children’s Hospital of Soochow University, located in the southeast region of China. We performed a retrospective review of the records of hospitalized patients in the Respiratory Department from 1 January, 2017, to 31 December, 2018. As is shown in Figure 1, a total of 53,890 hospitalized children from 2017 to 2018 were included in the study. SP was detected positive in 3,407 patients and 3,472 SP strains were isolated from these patients. The bacteria quantity was confirmed by semi-quantitative culture, strains with result of + (very small amount) was excluded. A total of 2,446 strains were tested for antimicrobial susceptibility testing. Of the 2,446 isolates, the most common source was deep nasopharyngeal aspiration fluid (N=2,349; 96.03%), followed by bronchoalveolar lavage fluid (N=60; 2.45%), blood (N=24; 0.98%), cerebrospinal fluid (N=12; 0.49%), and pleural fluid (N=1; 0.04%). Strains separated from blood, cerebrospinal fluid, or pleural fluid were regarded as invasive isolates, while those separated from nasopharyngeal aspiration fluid or bronchoalveolar lavage fluid were regarded as non-invasive isolates. Among the isolates, we selected strains with semi-quantitative culture results of +++ (medium amount) to ++++ (large amount). Patients colonized by bacteria but without any clinical evidence of bacterial infection (symptoms: cough, fever (≥38.5 °C); laboratory examination: C-reactive protein >8 mg/L; white blood cell >10.0×109/L) were excluded. A total of 528 strains were selected and serotyped. The 528 SP isolates were collected from the deep nasopharyngeal aspirates, alveolar lavage fluid, or blood of 509 children with SP. Positive results were detected in both the bronchoalveolar lavage fluid and the deep nasopharyngeal aspirate of 19 hospitalized children. The median (interquartile range) age of the 509 children with SP was 24 months (8–41 months). According to the diagnostic criteria of pneumonia and severe pneumonia, the strains were divided into bronchitis pneumonia group and lobar pneumonia group; severe pneumonia group and no-severe pneumonia group. The study was conducted in accordance with the Declaration of Helsinki (as revised in 2013). The study was approved by the Fudan University School of Public Health Institutional Review Board (IRB#2017-11-0646) and individual consent for this retrospective analysis was waived.
Bacteria identification and serotyping
Samples were inoculated on blood agar plates and incubated at 35 °C in 5% CO2 incubators for 24 hours. SP isolates were identified by colony morphology on blood agar and optochin tests. The bacteria quantity was confirmed by semi-quantitative culture, expressed as + (very small amount), ++ (small amount), +++ (medium amount), and ++++ (large amount). Serogroups were tested using the Quellung reaction with Pneumotest kits, and serotypes were determined with factor antisera (Statens Serum Institute, Copenhagen, Denmark). The interpretation of the serotyping depended on the capsular swelling under phase contrast microscopy with an oil immersion lens (magnification, ×100).
Antimicrobial susceptibility testing
A total of 37 invasive isolates and 2,409 non-invasive isolates were tested. The minimum inhibitory concentrations (MICs) for erythromycin, clindamycin, penicillin G, tetracycline, linezolid, moxifloxacin, sulfamethoxazole-trimethoprim (SXT), amoxicillin, cefotaxime, chloramphenicol, vancomycin, rifampicin, and levofloxacin were determined by E-test strips (AB Biodisk, Solna, Sweden) in accordance with the guidelines established by the Clinical and Laboratory Standards Institute (CLSI) (7). In the CLSI 2017 criteria, the non-meningitis breakpoint was used to classify isolates as penicillin-susceptible (MIC ≤2 µg/mL), penicillin-intermediate (MIC 4–8 µg/mL), or penicillin-resistant (MIC ≥8 µg/mL), while meningitis strains with penicillin MIC ≤0.06 µg/mL are considered sensitive. MDR was defined as nonsusceptibility to at least one agent in three or more antimicrobial categories. SP ATCC 49619 was used as the standard strain for quality control.
Diagnostic criteria
Pneumonia: (I) symptoms: fever, cough, tachypnea, dyspnea, inspiratory depression of the chest wall, fixed wet rale in the lungs; (II) abnormal changes could be seen on chest X-ray. By chest imaging examination, community-acquired pneumonia was divided into a bronchitis group and a lobar pneumonia group. Severe pneumonia was defined according to the diagnostic criteria for pneumonia with at least one of the following seven criteria: (I) fever >38.5 °C, severe systemic poisoning symptoms; (II) tachypnea (infants >70 minutes, older children >50/min); (III) dyspnea; (IV) cyanosis; (V) involvement of multiple lobes or ≥2 or 3 lobes; (VI) complicated with obvious pleural effusion; (VII) percutaneous oxygen saturation ≤92%.
Statistical analysis
Statistical analyses were completed with IBM SPSS Statics (Version 22.0). We used n (%) for categorical variables and the median (quartiles) for continuous variables with non-normal distributions or the mean and standard deviation (SD) for those with normal distributions. Comparisons were performed with the Chi-square test. A cutoff P value <0.05 was considered statistically significant.
Results
Antimicrobial susceptibility
The antimicrobial susceptibility of the SP isolates is shown in Tables 1,2. The non-susceptible rates of erythromycin, clindamycin, tetracycline, and sulfamethoxazole were 99.9%, 98.1%, 89.7%, and 87.9%, respectively. The non-susceptible rate of penicillin was 9.5% (232/2,446), among them invasive isolates to penicillin was 48.6% (18/37), while non-invasive isolates was 8.9% (214/2,409). To other β-lactam antibiotics, such as amoxicillin and cefotaxime, the non-susceptible rate were 27.7% and 27.2%, respectively. About 99% of the isolates were susceptible to rifampicin, levofloxacin, and moxifloxacin, and all were susceptible to vancomycin and linezolid.
Table 1
Antibiotics | S (%) | I (%) | R (%) | Non-susceptiblea (%) |
---|---|---|---|---|
ERY | 3 (0.1) | 0 (0.0) | 2,443 (99.9) | 99.9 |
CLI | 46 (1.9) | 0 (0.0) | 2,400 (98.1) | 98.1 |
TCY | 251 (10.3) | 0 (0.0) | 2,195 (89.7) | 89.7 |
SXT | 295 (12.1) | 244 (10.0) | 1,907 (78.0) | 87.9 |
AMX | 1,763 (72.3) | 574 (23.6) | 100 (4.1) | 27.7 |
CTX | 1,780 (72.8) | 329 (13.5) | 337 (13.8) | 27.2 |
PEN | 2,214 (90.5) | 209 (8.5) | 23 (0.9) | 9.5 |
CHL | 2,223 (90.9) | 0 (0.0) | 223 (9.1) | 9.1 |
TEL | 1,748 (92.5) | 0 (0.0) | 142 (7.5) | 7.5 |
RD | 2,435 (99.6) | 1 (0.0) | 10 (0.4) | 0.4 |
LUV | 2,444 (99.9) | 1 (0.0) | 1 (0.0) | 0.1 |
MFX | 1,890 (99.9) | 0 (0.0) | 2 (0.1) | 0.1 |
VAN | 2,446 (100.0) | 0 (0.0) | 0 (0.0) | 0 |
LZD | 2,446 (100.0) | 0 (0.0) | 0 (0.0) | 0 |
a, non-susceptible: includes intermediate and resistance. SP, Streptococcus pneumoniae; S, susceptible; I, intermediate; R, resistant; ERY, erythromycin; CLI, clindamycin; SXT, sulfamethoxazole; AMX, amoxicillin; CTX, cefotaxime; PEN, penicillin; TCY, tetracycline; CHL, chloramphenicol; TEL, telithromycin; RD, rifampicin; LUV, levofloxacin; MFX, moxifloxacin; VAN, vancomycin; LZD, linezolid.
Table 2
Antibiotics | Non-invasive (n=2,409) | Invasive (n=37) | P | |||
---|---|---|---|---|---|---|
S (%) | Non-susceptible (%)a | S (%) | Non-susceptible (%)a | |||
ERY | 3 (0.1) | 2,046 (99.9) | 0 (0.0) | 37 (100.0) | – | |
CLI | 46 (1.9) | 2,363 (98.1) | 0 (0.0) | 37 (100.0) | – | |
TCY | 244 (10.1) | 2,165 (89.9) | 7 (18.9) | 30 (81.1) | 0.095 | |
SXT | 282 (11.7) | 2,127 (88.3) | 13 (35.1) | 24 (64.9) | <0.001 | |
AMX | 1,740 (72.2) | 669 (27.8) | 23 (82.1) | 5 (17.9) | 0.293 | |
CTX | 1,758 (73.0) | 651 (27.0) | 22 (59.5) | 15 (40.5) | 0.092 | |
PEN | 2,195 (91.1) | 214 (8.9) | 19 (51.4) | 18 (48.6) | <0.001 | |
CHL | 2,189 (90.9) | 220 (9.1) | 34 (91.9) | 3 (8.1) | >0.999 | |
TEL | 1,723 (92.5) | 139 (7.5) | 25 (89.3) | 3 (19.7) | 0.463 | |
RD | 2,398 (99.5) | 11 (0.5) | 37 (100.0) | 0 (0.0) | – | |
LUV | 2,407 (99.9) | 2 (0.1) | 37 (100.0) | 0 (0.0) | – | |
MFX | 1,865 (99.9) | 1(0.1) | 27 (96.4) | 1 (3.6) | 0.029 | |
VAN | 2,409 (100.0) | 0 (0.0) | 37 (100.0) | 0 (0.0) | – | |
LZD | 2,409 (100.0) | 0 (0.0) | 37 (100.0) | 0 (0.0) | – |
a, non-susceptible rate includes intermediate and resistance. S, susceptible, ERY, erythromycin; CLI, clindamycin; SXT, sulfamethoxazole; AMX, amoxicillin; CTX, cefotaxime; PEN, penicillin; TCY, tetracycline; CHL, chloramphenicol; TEL, telithromycin; RD, rifampicin; LUV, levofloxacin; MFX, moxifloxacin; VAN, vancomycin; LZD, linezolid.
The non-invasive isolates were completely sensitive to rifampicin, linezolid, levofloxacin, and vancomycin. The non-susceptible rate of invasive isolates to moxifloxacin was higher than that of non-invasive isolates (1/1,866, 0.1% vs. 1/28, 3.6%, P=0.029).
Resistance pattern and multidrug resistance of SP in Suzhou
The resistance patterns are shown in Table 3. We found that SP was resistant to eight kinds of antibiotics: macrolides (ERY), β-lactam (PEN, AMX, CTX), lincosamide (CLI), tetracycline (TCY), sulfonamides (SXT), amide alcohols (CHL), rifamycin (RD), and quinolones (LUV, MFX).
Table 3
Multi-resistance pattern | Non-invasive (n=2,326), n (%) | Invasive (n=35), n (%) | Major serotypes |
---|---|---|---|
MLS + SXT + CLI + TCY | 1,066 (45.8) | 0 (0.0) | 6A, 23F, 6B, 19F, 15B |
MLS + β + SXT + CLI + TCY | 688 (29.6) | 13 (37.1) | 19F, 19A, 6B, 23F, 6A |
MLS + SXT + CLI + TCY + CHL | 168 (7.2) | 2 (5.7) | 6B, 6A, 15B, 23F, 3 |
MLS + CLI + TCY | 153 (6.6) | 5 (14.3) | 23A, 14, 6A, NT, 23F |
MLS + SXT + CLI | 107 (4.6) | 0 (0.0) | 6A, 6B, 14, 19F, 23F |
MLS + β + SXT + CLI | 29 (1.2) | 1 (2.9) | 19F, 23F |
MLS + β + CLI + TCY | 20 (0.9) | 2 (5.7) | 14 |
MLS + β + SXT + CLI + TCY + CHL | 17 (0.7) | 1 (2.9) | 15B, 23A, 6B |
MLS + β + SXT + TCY | 15 (0.6) | 0 (0.0) | 19F |
MLS + CLI + TCY + CHL | 13 (0.6) | 0 (0.0) | 33A, 14 |
MLS + SXT + CLI + CHL | 10 (0.4) | 0 (0.0) | NT, 6B |
MLS + β + CLI | 10 (0.4) | 2 (5.7) | 14 |
MLS + SXT + TCY | 7 (0.3) | 0 (0.0) | 35F, 19F, 11A |
MLS + β + SXT + CLI + TCY + RD | 5 (0.2) | 0 (0.0) | – |
MLS + SXT + CLI + TCY + RD | 4 (0.2) | 7 (20.0) | – |
MLS + CLI + CHL | 2 (0.1) | 0 (0.0) | – |
MLS + TCY + CHL | 2 (0.1) | 0 (0.0) | – |
MLS + β + SXT + CLI + CHL | 2 (0.1) | 0 (0.0) | – |
MLS + CLI + RD | 1 (0.0) | 0 (0.0) | – |
MLS + SXT + TCY + CHL | 1 (0.0) | 0 (0.0) | – |
MLS + FLQs + SXT + CLI | 1 (0.0) | 0 (0.0) | – |
MLS + FLQs + SXT + CLI + TCY + CHL | 1 (0.0) | 0 (0.0) | – |
MLS + FLQs + SXT + CLI + TCY | 1 (0.0) | 0 (0.0) | – |
MLS + β + CLI + CHL | 1 (0.0) | 0 (0.0) | – |
MLS + β + CLI + TCY + CHL | 1 (0.0) | 1 (2.9) | – |
MLS + β + SXT + CLI + TCY + CHL + RD | 1 (0.0) | 0 (0.0) | – |
MLS + FLQs + CLI | 0 (0.0) | 1 (2.9) | – |
MLS, macrolides; SXT, sulfamethoxazole; CLI, clindamycin; TCY, tetracycline; β, β-lactams; FLQs, fluoroquinolones; CHL, chloramphenicol; RD, rifampicin.
Non-invasive isolates with MDR accounted for 96.6% (2,326/2,409) of all strains. Invasive isolates with MDR accounted for 94.6% (35/37) of all strains. The MDR strains showed 27 patterns of resistance. The most extensive resistance pattern of non-invasive isolates was MLS + SXT + CLI + TCY (1,066/2,326, 45.8%). The major serotypes of this resistance pattern were 6A, 23F, 6B, 19F, 15B. The most extensive resistance pattern of invasive isolates was MLS + β + SXT + CLI + TCY (13/35, 37.1%). The most common serotypes of the pattern were 19F, 19A, 6B, 23F, 6A.
Serotype distribution and PCV coverage
A total of 35 different serotypes were identified among 513 pneumococcal isolates. It is important to note that 15 isolates (2.8%) remained non-typeable (NT).
The most common serotypes were 19F (28.6%), 6B (11.9), 23F (11.2%), 6A (10.6%), and 19A (9.1%), which altogether accounted for 71.4% of all isolates. The serotype distributions and the PCV coverage rates are shown in Figure 2. The percentage of vaccine serotypes in total serotypes indicates the coverage rate of serotypes. The coverage rates of PCV7 and PCV13 in all isolates reached 60.4% (310/513) and 80.9% (415/513), respectively. The coverage rate of non-vaccine serotypes (NVT) was 19.1% (98/513).
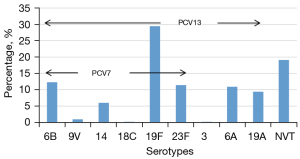
Serotype distribution of SP with different antimicrobial susceptibility
Analysis of the serotype distribution showed antimicrobial-susceptibility-dependent variations. The percentage of serotypes according to the antimicrobial resistance of SP is shown in Figure 3. Among the isolates susceptible to penicillin, the major serotypes were NVT, 19F, 6B, 6A, 23F, while serotypes 19F, 19A, 23F, 6B, and 14 were the prominent serotypes among the non-susceptible cases. Serotypes NVT, 6B, 6A, 23F, and 19F were the most common in strains sensitive to β-lactam, while among the non-susceptible cases, the most common serotypes were 19F, 19A, NVT, 6B, 23F. Among the non-MDR SP isolates, the most prevalent serotypes were 14, 23F, 19F, NVT, and 6A. In the isolates with MDR, the first five most common serotypes were 19F, NVT, 6B, 6A and 23F.
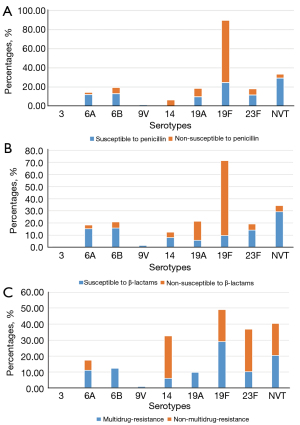
Serotype distribution agents by disease
The top five serotypes in the age group of ≤6 months were 19F, 6B, 23F, 6A, and 19A. The most common serotypes in the age group of 6 to 12 months were 19F, 23F, 6B, 6A, and 19A. The top five serotypes in the age group of 1 to 3 years (excluding 1 year old) were 19F, 23F, 6A, 19A, and 6B. The top five serotypes in the age group of 3 to 5 years (excluding 3 year old) were 19F, 6B, 19A, 23F, and 6A. The top five serotypes in the age group of >5 years were 19F, 6A, 6B, 14, and 23F.
Among the 509 children infected with SP, 475 cases were diagnosed as pneumonia (429 cases of bronchopneumonia and 46 cases of lobar pneumonia). Among the cases of pneumonia, 56 cases were diagnosed as severe pneumonia (11.8%), and 419 cases were non-severe pneumonia (88.2%). The distribution of SP serotype agents by disease is shown in Figure 4. Among the bronchitis group, the major serotypes were 19F, NVT, 6B, 6A, and 23F, while serotypes 19F, 23F, NVT, 6B and 14 were the prominent serotypes among the lobar pneumonia group. Serotypes 19F, NVT, 23F, 6B, and 6A were the most common in non-severe pneumonia, while serotypes 19F, 6B, NVT, 23F, and 6A were the prevalent serotypes among severe cases.
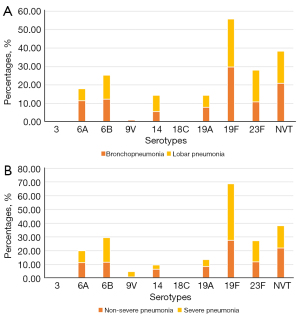
Discussion
In this study, the non-susceptible rates of SP to erythromycin and clindamycin were more than 95%. This is consistent with a six-year multicenter study, which illustrated that this kind of antibiotic was not recommended in clinical treatment (8). The abuse of macrolide antibiotics may be the cause of the high resistance prevalence (9). Rifampicin, levofloxacin, and moxifloxacin showed high susceptible rates, and no isolates were resistant to vancomycin and linezolid, which is comparable to the results from other reports (10). This can be explained by the fact that these antibiotics are not commonly used in children.
Penicillin is still widely used in clinical practices. There are differences in the sensitivity of SP detected in different regions to penicillin. We reported the resistance rate against penicillin was 9.5%, with an insensitivity rate of 48.6% for invasive isolates and 8.9% for non-invasive isolates, which differs from reports from other countries (11,12). The difference may be related to various sources of SP isolates and different indications for the use of antibiotics. Our study showed that the non-susceptible rates to other β-lactam antibiotics, such as amoxicillin and cefotaxime, were 27.7% and 27.2%, suggesting that the choice of first-line antibiotics for patients with suspected invasive pneumococcal disease was more potent β-lactams, such as amoxicillin.
According to a study from the Asian Network for Surveillance of Resistant Pathogens (ANSORP), MDR was observed in 49.5% and in 50.8% of isolates in 2008–2009 and 2012–2017, respectively, with the highest rates observed in China (58.4% and 76.0%, respectively) (13). In our study, 96.6% of non-invasive isolates and 94.6% of invasive isolates showed MDR. MDR isolates are widespread in clinical infections and greatly threaten children’s health. Therefore standardizing the use of antibiotics and dynamically monitoring the antimicrobial resistance of SP is recommended. In this study, we found that macrolides + sulfamethoxazole + clindamycin + tetracycline was the most extensive resistance pattern of non-invasive isolates in Suzhou, while macrolides + β-lactams + sulfamethoxazole + clindamycin + tetracycline was the most extensive resistance pattern of invasive isolates. In addition, we found that different resistance patterns are related to different serotypes, which point out susceptibility is highly related to clones. These findings provide some guidance for clinical treatment.
Serotype distribution among different countries is remarkably different. In this study, the most prevalent pneumococcal serotype was 19F, and similar findings have been observed in most domestic reports in recent years (14,15). This finding differs from previous studies conducted in other countries, including the USA (35B, 3, 23A) and Japan (12F, 3, 23A, 19A) (16,17). Usually, vaccine introduction was followed by a decrease in the rate of vaccine serotype accompanied by an increase in the diversity of non-vaccine serotypes (13), which is called serotype replacement. For children, PCV7 became commercially available in China in 2008 and was replaced by the PCV13 in 2017. Unlike the vaccination in Chinese EPI schedule, the PCV7 vaccine was not free to the public and the coverage was estimated as 10% (18). Such low vaccination may not produce satisfactory results for reducing the burden of pneumococcal diseases. Which may explain that serotypes included in the PCV7 are still among the most frequently identified serotypes. Compared with the isolates from the same hospital before the introduction of PCV13 from July 2012 to July 2013 (19), the serotype coverage rates decreased from 89.9% to 80.9%. Serotype replacement was not observed in this study, which related to the low PCV13 vaccination rate at present (12.4%). Hence, long-term surveillance of SP is required to monitor the dynamic changing of serotypes and antimicrobial resistance.
Most resistant strains show a relatively concentrated serotype distribution. In this study, extensive β-lactam antibiotic resistance was observed more in 19F, 19A, which were highly similar to serotypes noted as commonly non-susceptible in a Canada study (20). In this study, there were differences in the distribution of serotypes with different antimicrobial susceptibility. Among non-susceptible SP isolates, the common serogroups could be covered by PCV13. It is suggested that popularizing vaccination is positive in controlling the prevalence of antibiotic-resistant isolates. Several studies have reported that the most prevalent serotypes of MDR isolates were 6B, 19A, 19F, 23F, and 14 (13,21), differed from our research results. The increase of non-vaccine serotypes in MDR bacteria brings new challenges to medical care. McCollum et al. reported that the incidence rate and mortality of children under 5 years were reduced by 13.3–87.7% after universal inoculation of PCV (2). This study found that the distribution of serotypes was significantly different for different severities of pneumonia. For lobar pneumonia and severe pneumonia, the percentage of NVT is lower, suggesting PCV13 is of protective effectiveness against pneumonia.
Our study has several limitations. This study a single-center study, which may not reflect the full distribution of serotypes across Suzhou. In addition, it only collects SP isolates recovered from patients hospitalized in general ward. The lack of data on ICU patients may lead to some selection bias. Finally, we did not have access to the PCV vaccination status of pneumonia patients from whom isolates were obtained; therefore, we could not compare isolates from vaccinated and unvaccinated individuals.
Conclusions
In conclusion, this study reported the antimicrobial susceptibility and serotype distribution of SP isolates from children in Suzhou, China, between 2017 and 2018. The most common resistance pattern of non-invasive isolates was macrolides + sulfamethoxazole + clindamycin + tetracycline. The most extensive resistance pattern of invasive isolates was macrolides + β-lactams + sulfamethoxazole + clindamycin + tetracycline. Serotypes 19F, 6B, 23F, 6A, and 19A were identified as predominant in Suzhou. PCV13 serotype coverage was 80.9%. Strains belonging to different serotypes exhibited distinct antimicrobial resistance patterns and were found to be associated with different diseases. PCV13 vaccination would be beneficial for children to alleviate suffering from SP-related diseases.
Acknowledgments
Funding: This work was supported by
Footnote
Reporting Checklist: The authors have completed the STROBE reporting checklist. Available at https://tp.amegroups.com/article/view/10.21037/tp-23-547/rc
Data Sharing Statement: Available at https://tp.amegroups.com/article/view/10.21037/tp-23-547/dss
Peer Review File: Available at https://tp.amegroups.com/article/view/10.21037/tp-23-547/prf
Conflicts of Interest: All authors have completed the ICMJE uniform disclosure form (available at https://tp.amegroups.com/article/view/10.21037/tp-23-547/coif). The authors have no conflicts of interest to declare.
Ethical Statement: The authors are accountable for all aspects of the work in ensuring that questions related to the accuracy or integrity of any part of the work are appropriately investigated and resolved. The study was conducted in accordance with the Declaration of Helsinki (as revised in 2013). The study was approved by the Fudan University School of Public Health Institutional Review Board (IRB#2017-11-0646) and individual consent for this retrospective analysis was waived.
Open Access Statement: This is an Open Access article distributed in accordance with the Creative Commons Attribution-NonCommercial-NoDerivs 4.0 International License (CC BY-NC-ND 4.0), which permits the non-commercial replication and distribution of the article with the strict proviso that no changes or edits are made and the original work is properly cited (including links to both the formal publication through the relevant DOI and the license). See: https://creativecommons.org/licenses/by-nc-nd/4.0/.
References
- Kang L, Jing W, Liu J, et al. Trends of global and regional aetiologies, risk factors and mortality of lower respiratory infections from 1990 to 2019: An analysis for the Global Burden of Disease Study 2019. Respirology 2023;28:166-75. [Crossref] [PubMed]
- McCollum ED, Nambiar B, Deula R, et al. Impact of the 13-Valent Pneumococcal Conjugate Vaccine on Clinical and Hypoxemic Childhood Pneumonia over Three Years in Central Malawi: An Observational Study. PLoS One 2017;12:e0168209. [Crossref] [PubMed]
- Kim GR, Kim EY, Kim SH, et al. Serotype Distribution and Antimicrobial Resistance of Streptococcus pneumoniae Causing Invasive Pneumococcal Disease in Korea Between 2017 and 2019 After Introduction of the 13-Valent Pneumococcal Conjugate Vaccine. Ann Lab Med 2023;43:45-54. [Crossref] [PubMed]
- Angoulvant F, Cohen R, Doit C, et al. Trends in antibiotic resistance of Streptococcus pneumoniae and Haemophilus influenzae isolated from nasopharyngeal flora in children with acute otitis media in France before and after 13 valent pneumococcal conjugate vaccine introduction. BMC Infect Dis 2015;15:236. [Crossref] [PubMed]
- Amin-Chowdhury Z, Groves N, Sheppard CL, et al. Invasive pneumococcal disease due to 22F and 33F in England: A tail of two serotypes. Vaccine 2021;39:1997-2004. [Crossref] [PubMed]
- Nakano S, Fujisawa T, Ito Y, et al. Nationwide surveillance of paediatric invasive and non-invasive pneumococcal disease in Japan after the introduction of the 13-valent conjugated vaccine, 2015-2017. Vaccine 2020;38:1818-24. [Crossref] [PubMed]
- Clinical and Laboratory Standard Institute. Performance standards for antimicrobial susceptibility testing; twenty-seventh informational supplement. M100-S27. Wayne, Pennsylvania. CLSI, 2017.
- Zhou M, Wang Z, Zhang L, et al. Serotype Distribution, Antimicrobial Susceptibility, Multilocus Sequencing Type and Virulence of Invasive Streptococcus pneumoniae in China: A Six-Year Multicenter Study. Front Microbiol 2021;12:798750. [Crossref] [PubMed]
- He X, Xie M, Li S, et al. Antimicrobial resistance in bacterial pathogens among hospitalized children with community acquired lower respiratory tract infections in Dongguan, China (2011-2016). BMC Infect Dis 2017;17:614. [Crossref] [PubMed]
- Zhao W, Pan F, Wang B, et al. Epidemiology Characteristics of Streptococcus pneumoniae From Children With Pneumonia in Shanghai: A Retrospective Study. Front Cell Infect Microbiol 2019;9:258. [Crossref] [PubMed]
- Li XX, Xiao SZ, Gu FF, et al. Serotype Distribution, Antimicrobial Susceptibility, and Multilocus Sequencing Type (MLST) of Streptococcus pneumoniae From Adults of Three Hospitals in Shanghai, China. Front Cell Infect Microbiol 2019;9:407. [Crossref] [PubMed]
- Al-Jardani A, Al Rashdi A, Al Jaaidi A, et al. Serotype distribution and antibiotic resistance among invasive Streptococcus pneumoniae from Oman post 13-valent vaccine introduction. Int J Infect Dis 2019;85:135-40. [Crossref] [PubMed]
- Kim SH, Chung DR, Song JH, et al. Changes in serotype distribution and antimicrobial resistance of Streptococcus pneumoniae isolates from adult patients in Asia: Emergence of drug-resistant non-vaccine serotypes. Vaccine 2020;38:6065-73. [Crossref] [PubMed]
- Huang LD, Yang MJ, Huang YY, et al. Molecular Characterization of Predominant Serotypes, Drug Resistance, and Virulence Genes of Streptococcus pneumoniae Isolates From East China. Front Microbiol 2022;13:892364. [Crossref] [PubMed]
- Huang X, Tan H, Lu F, et al. Molecular characterization of invasive Streptococcus pneumoniae clinical isolates from a tertiary children's hospital in eastern China. Microbiol Spectr 2023; Epub ahead of print. [Crossref]
- Suaya JA, Mendes RE, Sings HL, et al. Streptococcus pneumoniae serotype distribution and antimicrobial nonsusceptibility trends among adults with pneumonia in the United States, 2009-2017. J Infect 2020;81:557-66. [Crossref] [PubMed]
- Yanagihara K, Kosai K, Mikamo H, et al. Serotype distribution and antimicrobial susceptibility of Streptococcus pneumoniae associated with invasive pneumococcal disease among adults in Japan. Int J Infect Dis 2021;102:260-8. [Crossref] [PubMed]
- Lyu S, Hu HL, Yang YH, et al. A systematic review about Streptococcus Pneumoniae serotype distribution in children in mainland of China before the PCV13 was licensed. Expert Rev Vaccines 2017;16:997-1006. [Crossref] [PubMed]
- Geng Q, Zhang T, Ding Y, et al. Molecular characterization and antimicrobial susceptibility of Streptococcus pneumoniae isolated from children hospitalized with respiratory infections in Suzhou, China. PLoS One 2014;9:e93752. [Crossref] [PubMed]
- Golden AR, Baxter M, Adam HJ, et al. PCV-15 and PPSV-23 coverage of invasive and respiratory tract Streptococcus pneumoniae, including MDR and XDR isolates: CANWARD 2007-20. J Antimicrob Chemother 2022;77:1444-51. [Crossref] [PubMed]
- Shi W, Li J, Dong F, et al. Serotype distribution, antibiotic resistance pattern, and multilocus sequence types of invasive Streptococcus pneumoniae isolates in two tertiary pediatric hospitals in Beijing prior to PCV13 availability. Expert Rev Vaccines 2019;18:89-94. [Crossref] [PubMed]
(English Language Editor: D. Fitzgerald)