Dynamics of platelet parameters in children with severe community-acquired pneumonia between viral and bacterial infections
Highlight box
Key findings
• Predictors such as plateletcrit (PLT) or PLT combined with C-reactive protein and procalcitonin at 72 h of admission could assist in differentiating bacterial-involved and viral infections, but their sensitivity and specificity are not ideal.
What is known and what is new?
• Platelets are involved in inflammation and anti-infection, and their levels are strongly related to the severity of the disease. However, little is known about their potential role in diagnosing pathogens in children with severe community-acquired pneumonia (CAP).
• PLT of the viral infection group was significantly lower than those of the bacterial infection and bacterial-viral co-infection groups. Additionally, the curve of the absolute value of PLT (ΔPLT) in the virus-infected group was distinctly separated from the other two groups at 72 h.
What is the implication, and what should change now?
• Although the diagnostic value of platelet parameters in bacterial and viral infection in children with severe CAP is limited, they are still expected to be combined with other indicators to provide a reference for timely treatment.
Introduction
Community-acquired pneumonia (CAP) is one of the most common infectious diseases, with high mortality and morbidity among children under 5 years of age, especially in developing countries. In 2019, approximately 740,180 children aged ≤5 years died of pneumonia (1). The incidence of CAP in children under 5 years of age in China is 65.8 per 1,000 person-years, with 12–20% of patients admitted to the intensive care unit (ICU) (2). For children with severe CAP, clinicians urgently need to identify viral or bacterial infections at an early stage to provide corresponding anti-infective treatment in time and improve poor prognosis (3,4).
Several biomarkers in blood routine, including white blood cell (WBC) count, neutrophil percentage (NP), serum C-reactive protein (CRP), and procalcitonin, are commonly used in the diagnostic process for CAP inpatients. However, it is worth noting that the specificity of these changes is not sufficient to accurately predict pathogenic pathogens (5,6). Recent studies have shown that platelets are involved in the inflammation and anti-infection (7). Changes in platelet counts are strongly related to the severity of the disease (8-10).
Changes in platelet parameters may vary according to the different pathogens (11). Many invasive microbial pathogens can target host platelets directly or indirectly, altering platelet count or function (12). Some smaller viruses, such as enteroviruses, adenoviruses, and herpesviruses, can bind to and internalize platelets, thereby increasing the formation of platelet-monocyte aggregates (13). However, little is known about the differences in platelet parameters in viral and bacterial infections in children with severe CAP.
In this study, we retrospectively analyzed the dynamic differences in platelet parameters in viral, bacterial and mixed infections in children with severe CAP to explore whether they can assist distinguishing between viral and bacterial infection. We present this article in accordance with the STROBE reporting checklist (available at https://tp.amegroups.com/article/view/10.21037/tp-23-441/rc).
Methods
Patients
From January 1, 2016 to June 30, 2019, the medical records of children admitted to the Pediatric Intensive Care Unit (PICU) at Beijing Children’s Hospital, Capital Medical University for severe CAP were retrospectively reviewed. This study aimed to examine changes in platelet parameters in children with viral and/or bacterial severe CAP. During the study period, the PICU had a capacity of 27 beds and admitted approximately 1,200 critically ill children annually, excluding newborns and those who had undergone cardiac surgery. Children aged ≥29 days to 18 years were enrolled if they were hospitalized with severe CAP, as defined by the World Health Organization (WHO) (2013 version) (14).
The patients were identified as having at least one of the following risk factors: central cyanosis or hypoxemia (oxygen saturation <90% measured by pulse oximeter), severe respiratory distress (e.g., grunting, very severe chest indrawn breaths), inability to breastfeed or drink, lethargy or unconsciousness, or convulsions (15). The following patients were excluded: (I) concomitant hematologic underlying diseases, such as leukemia, aplastic anemia, immune thrombocytopenia, and Hemophagocytic syndrome; (II) use of antiplatelet drugs, such as dipyridamole 2 weeks prior to or during the course of the disease; (III) history of platelet transfusion before admission; (IV) length of hospitalization <48 hours; (V) lack of platelet profile; and (VI) mycoplasma, fungal, and other pathogenic infections.
The study protocol was reviewed and approved by the Ethics Committee of Beijing Children’s Hospital (No. 2018-k-103). The study was conducted in accordance with the Declaration of Helsinki (as revised in 2013). Patient informed consent was waived due to the retrospective nature of the study.
Defined etiology
Pathogenic examination results of respiratory secretions (sputum, bronchial lavage fluid, nasopharyngeal swabs), blood, and pleural effusion samples were collected within 24 h after admission of patients with severe CAP. Qualified sputum specimens met the observation of 1–9 squamous cells per field and more than 25 polymorphonuclear leukocytes in the low-magnification field of view. Viruses in nasal aspirate samples were tested by antigen testing, nucleic acid testing, or antibody testing. Bacterial infections were diagnosed by the isolation of a pathogen from bronchoalveolar lavage fluid (BALF) or blood culture.
Classification of patients according to pathogens
Pneumonia was defined as (I) bacterial, if microbiological detection showed one or more bacteria and no virus; (II) viral, if microbiological detection revealed one or more viruses and no bacterium; (III) mixed (virus-bacteria), if microbiological detection revealed at least one virus and one bacterium; and (IV) no etiology, if microbiological investigation showed no virus or no bacteria (16).
Data collection
Clinical data were obtained from the electronic records of patients. Data collection was carried out in the following aspects: (I) general information: demographics, underlying diseases, complications, main treatment measures, length of hospital stay, and mortality at discharge. (II) Laboratory data: microbiological investigations, initial laboratory tests within 24 hours after admission, and changes of in platelet parameters, including platelet count (PLT), mean platelet volume (MPV), platelet distribution width (PDW) and plateletcrit (PCT), were collected at 24 h, 48 h, 72 h, and day 7 of admission and discharge.
The difference in platelet counts between the observation time point and the 1st day of admission [ΔPLT = (PLT at observation time point) – (PLTd1)] was calculated as an indicator of PLT changes. This method was also applied to other platelet parameters (17).
Statistical analysis
Statistical software SPSS 23.0 was used for data analysis in this study. Categorical variables were expressed as the number of cases and percentages, and continuous variables as median ± interquartile range (IQR). Univariate analysis for categorical variables was performed using Fisher’s exact test or Pearson’s Chi squared test (as appropriate). Continuous variables were compared using the Mann Whitney U test or Student’s t-test. The diagnostic performance of the PLT for differentiating severe viral and bacterial CAP was assessed by the area under curve (AUC) (18). The sensitivity, specificity, positive predictive value (PPV), and negative predictive value (NPV) were calculated. Statistical significance was set at P<0.05. Bivariable Cox regression was conducted to determine the influence of potential factors in comorbidities.
Sample size evaluation
The sample size was calculated based on the average area under receiver operating characteristic curve (AUROC) of 0.763, which was obtained from previous studies that investigated the use of serum markers to differentiate bacterial and viral infections in children (19-21). With an 80% power at a significance level of 5%, it was determined that a minimum sample size of 126 would be required, considering an expected mortality rate of 9.3% (22).
Results
Microbial etiology
During the study period, of the 395 patients with severe CAP entered into the database, 156 met our selection criteria. Of the 156 patients, 129 (82.7%) had an identified CAP etiology. Of these patients, 49 (49/156, 31.4%) had viral infections, 38 (38/156, 24.4%) had bacterial infections, and 42 (42/156, 26.9%) had mixed bacterial and viral infections. Twenty-seven patients (27/156, 17.3%) had negative microbiological results (Figure 1).
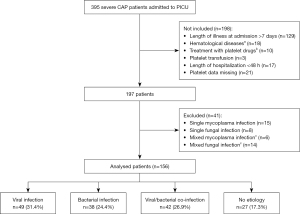
In total 185 pathogens are identified, as shown in Table 1. It was observed that single pathogen infections were predominant in the viral or bacterial infection groups, accounting for 93.9% and 81.6%, respectively. In the virus-bacterial mixed infection group, the highest proportion was seen in cases of single bacterial infection combined with single virus infection, which accounted for 90.5%. The top three viruses were respiratory syncytial virus (RSV) (28.6%, 53/185), influenza A/B virus (13.0%, 24/185), parainfluenza virus type III (7.0%, 13/185). The top three bacteria were S pneumoniae (13.5%, 25/185), S aureus (12.4%, 23/185) and H influenzae (11.4%, 21/185).
Table 1
Organisms isolated | All (n=156) | Viral (n=49) | Bacterial (n=38) | Mixed (n=42) |
---|---|---|---|---|
Not detected | 27 (17.3) | – | – | – |
Type of infection | ||||
1 | 77 (49.4) | 46 (93.9) | 31 (81.6) | – |
2 | 47 (30.1) | 3 (6.1) | 6 (15.8) | 38 (90.5) |
>2 | 5 (3.2) | 0 | 1 (2.6) | 4 (9.5) |
Number of pathogens | 185 | 52 | 44 | 89 |
Virus | ||||
RSV | 53 (28.6) | 27 (51.9) | – | 26 (29.2) |
Influenzae A/B virus | 24 (13.0) | 18 (34.6) | – | 6 (6.7) |
Parainfluenza | 13 (7.0) | 5 (9.6) | – | 8 (9.0) |
Adenovirus | 4 (2.2) | 2 (3.8) | – | 2 (2.2) |
Bacterium | ||||
S pneumoniae | 25 (13.5) | – | 11 (25.0) | 14 (15.7) |
S aureus | 23 (12.4) | – | 13 (29.5) | 10 (11.2) |
H influenzae | 21 (11.4) | – | 9 (20.5) | 12 (13.5) |
K pneumoniae | 14 (7.6) | – | 4 (9.1) | 10 (11.2) |
P aeruginosa | 2 (1.1) | – | 2 (4.5) | 0 |
Other* | 6 (3.2) | – | 5 (11.4) | 1 (1.1) |
*, E coli, E cloacae, Stenotrophomonas maltophilia. Data are presented as number, or n (%). CAP, community acquired pneumonia; RSV, respiratory syncytial virus.
Demographic characteristics and clinical information
The median age of the children was 10.2 months (IQR: 3.6, 24 months), and 88 children (56.4%) were male. Among them, 70.5% (110/156) had underlying disease, with congenital heart disease being the most common disease. The complication rate in these patients was 94.2% (147/156), and respiratory failure was the most common complication. Invasive mechanical ventilation was used in 39.1% (61/156) of these children. The median ICU and hospital length of stay was 7 days (IQR: 3.2, 10.6 days) and 11 days (IQR: 6.9, 24.7 days), respectively.
The highest percentage of congenital heart disease (23/49, 46.9%) and structural abnormalities of the airway (12/49, 24.5%) were found in children with viral infections, and the incidence of heart failure (7/38, 18.4%) and pneumothorax (4/38, 10.5%) was higher in children with bacterial infections (Table 2). Moreover, the length of ICU stay and total length of hospital stay were significantly lower in those with viral infections than in those with bacterial or mixed infections.
Table 2
Characteristics | Viral (n=49) | Bacterial (n=38) | Mixed (n=42) |
---|---|---|---|
Male (%) | 28 (57.1) | 17 (44.7) | 26 (61.9) |
Age (month), median [IQR] | 10 [4–24] | 12 [6–36] | 11 [7–24] |
Co-morbidities, n (%) | |||
Congestive heart failure | 23 (46.9) | 8 (21.1) | 18 (42.9) |
Abnormal respiratory structure | 12 (24.5) | 5 (13.2) | 2 (4.8) |
Abnormal birth history | 5 (10.2) | 3 (7.9) | 2 (4.8) |
Neurological disorders | 5 (10.2) | 5 (13.2) | 4 (9.5) |
Malnutrition | 4 (8.2) | 2 (5.3) | 2 (4.8) |
Bronchopulmonary dysplasia | 4 (8.2) | 3 (7.9) | 2 (4.8) |
Asthma | 5 (10.2) | 2 (5.3) | 0 |
Chronic liver/kidney disease | 1 (2.0) | 1 (2.6) | 2 (4.8) |
Inherited metabolic disease | 2 (4.1) | 1 (2.6) | 0 |
Complications, n (%) | |||
Respiratory failure | 45 (91.8) | 33 (86.8) | 42 (100.0) |
ARDS | 5 (10.2) | 8 (21.1) | 4 (9.5) |
Pleural fluid | 5 (10.2) | 6 (15.8) | 8 (19.0) |
Heart failure | 2 (4.1) | 7 (18.4) | 4 (9.5) |
Septic shock | 5 (10.2) | 1 (2.6) | 2 (4.8) |
Acute renal failure | 3 (6.1) | 3 (7.9) | 4 (9.5) |
Pneumothorax | 0 | 4 (10.5) | 4 (9.5) |
Respiratory failure | 2 (4.1) | 3 (7.9) | 0 |
PIM2 (%), median [IQR] | 11 [3–20] | 12 [2–24] | 13 [2–26] |
Support treatment, n (%) | |||
Mechanical ventilation | 18 (36.7) | 21 (55.3) | 20 (47.6) |
CRRT | 1 (2.0) | 1 (2.6) | 2 (4.8) |
ECMO | 1 (2.0) | 0 | 0 |
Length of stay (days), median [IQR] | |||
PICU | 6 [5–9] | 8 [5–14] | 8 [5–12] |
Hospital | 11 [8–14] | 13 [9–21] | 13 [8–16] |
Mortality, n (%) | 6 (12.2) | 5 (13.2) | 5 (11.9) |
Comorbidities are underlying diseases that occur simultaneously with severe CAP. Abnormal birth history is defined as premature, low-birth weight, or small for gestational age. Malnutrition refers to deficiencies, excesses or imbalances in a person’s intake of energy and/or nutrients. IQR, interquartile range; ARDS, acute respiratory distress syndrome; PIM2, Pediatric Mortality Index 2; CRRT, continuous renal replacement therapy; ECMO, extracorporeal membrane oxygenation; PICU, pediatric intensive care unit; CAP, community acquired pneumonia.
Dynamic differences of platelet parameters in viral, bacterial and mixed infections
Table 3 presents a comparison of platelet parameters among patients with viral, bacterial and mixed infections at different periods during hospitalization (at 24 h, 48 h, 72 h, and day 7 of admission, and discharge). It was observed that only at 72 h of admission, PLT was significantly lower in the viral infection group than in the bacterial and mixed infection groups (P=0.025, P=0.001). Furthermore, we analyzed the absolute changes in platelet parameters at different observation times using the values of platelet parameters upon admission as a reference. The resulting dynamic curve is shown in Figure 2. It can be observed that after 72 hours of admission, the bacterial and mixed infection groups had a significant increase in their ΔPLT levels, while the virus infected group maintained its PLT level during hospitalization without any upward trend. However, ΔMPV, ΔPDW, and ΔPCT did not differ significantly among the three groups.
Table 3
Parameters | Viral | Bacterial | Mixed | P |
---|---|---|---|---|
24 h | ||||
N | 49 | 38 | 42 | |
PLT (109/L) | 339±27 | 333±30 | 300±31 | 0.664 |
MPV (fL) | 9.7±0.2 | 9.5±0.3 | 9.7±0.2 | 0.377 |
PDW (fL) | 10.7±0.3 | 10.6±0.4 | 10.6±0.3 | 0.182 |
PCT | 0.32±0.02 | 0.33±0.03 | 0.29±0.03 | 0.772 |
48 h | ||||
N | 44 | 30 | 39 | |
PLT (109/L) | 327±25 | 304±31 | 304±34 | 0.771 |
MPV (fL) | 9.9±0.2 | 9.5±0.4 | 9.6±0.2 | 0.353 |
PDW (fL) | 10.9±0.3 | 11.5±0.3 | 11.8±0.6 | 0.588 |
PCT | 0.32±0.02 | 0.31±0.03 | 0.30±0.03 | 0.815 |
72 h | ||||
N | 44 | 34 | 39 | |
PLT (109/L) | 330±25b | 417±27a | 409±30a | 0.073 |
MPV (fL) | 9.8±0.2 | 9.7±0.1 | 9.8±0.2 | 0.836 |
PDW (fL) | 11.2±0.3 | 11.0±0.5 | 11.5±0.5 | 0.604 |
PCT | 0.33±0.02 | 0.37±0.03 | 0.39±0.04 | 0.292 |
Day 7 | ||||
N | 42 | 33 | 37 | |
PLT (109/L) | 441±36 | 470±36 | 507±48 | 0.535 |
MPV (fL) | 9.7±0.1 | 9.9±0.2 | 9.5±0.2 | 0.669 |
PDW (fL) | 11.2±0.3 | 11.5±0.5 | 10.6±0.3 | 0.853 |
PCT | 0.44±0.03 | 0.42±0.03 | 0.48±0.05 | 0.461 |
Discharge | ||||
N | 34 | 32 | 27 | |
PLT (109/L) | 532±38 | 491±34 | 452±58 | 0.552 |
MPV (fL) | 9.3±0.1 | 9.2±0.2 | 9.2±0.2 | 0.795 |
PDW (fL) | 10.4±0.3 | 10.1±0.3 | 9.9±0.3 | 0.807 |
PCT | 0.49±0.04 | 0.44±0.03 | 0.41±0.05 | 0.451 |
Data are presented as mean ± SD. a and b indicate P<0.05 versus viral and bacterial, respectively. CAP, community acquired pneumonia; PLT, platelet count; MPV, mean platelet volume; PDW, platelet distribution width; PCT, plateletcrit; SD, standard deviation.
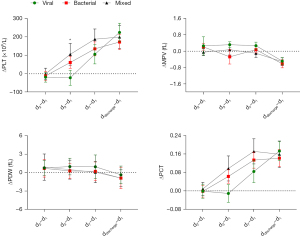
Evaluation of diagnostic performance in platelet parameters to differentiate bacterial and viral infections
Receiver operating characteristic (ROC) analysis for PLT to discriminate children with severe CAP with bacterial or mixed from viral infection showed an AUC for discriminating between bacterial involved (bacterial and mixed) and viral infection were 0.683 [P=0.007, 95% confidence interval (CI): 0.561–0.805], and there were differences between bacterial and viral infections (AUC =0.638, P=0.044, 95% CI: 0.509–0.766), and mixed and viral infections (AUC =0.695, P=0.018, 95% CI: 0.552–0.839) (Figure 3A). A cut off of PLT at 339×109/L in bacterial-involved (bacterial and mixed) vs. viral infection yielded a sensitivity of 68% and a specificity of 65%.
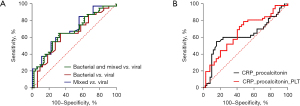
Meanwhile, levels of serum procalcitonin and CRP on day 3 after admission were also collected to assess whether PLT could be incorporated into a composite scoring tool. The AUC value for diagnosis of bacterial involved infection using procalcitonin and CRP was 0.626 (95% CI: 0.503–0.748, P=0.040, sensitivity 55%, specificity 85%). However, when PLT levels were added to the combination model of procalcitonin and CRP, the AUC value increased to 0.663 (95% CI: 0.549–0.774, P=0.008), with a sensitivity of 79%, a specificity of 53% (Figure 3B). This suggested that these predictors together were more effective in identifying bacterial infections in severe CAP.
To eliminate the impact of confounding factors on platelet parameter prediction models, bivariate Cox regression was conducted to assess the effects of nine potential comorbidities, such as congestive heart failure, respiratory structural abnormalities, birth history abnormalities, neurological diseases, malnutrition, bronchopulmonary dysplasia, asthma, chronic liver and kidney disease, and hereditary metabolic disease. These comorbidities did not affect the ability of platelet parameters to identify virus or bacterial infections at a P value of ≥0.25 level.
Discussion
Viruses and bacteria are the main infectious pathogens causing severe CAP in children. Recently, the proportion of bacterial infections has decreased in children with severe CAP, and viral infections have become more common (1). In this study, viral infections were found to be more prevalent than bacterial infections in 156 children with severe CAP, at a rate of 36.3% and 28.1%, respectively. Single pathogen infections were the most common type observed, with RSV being the most frequently identified viral pathogen. A prospective cross-sectional study on the etiology of hospitalized children with CAP under 5 years of age also found that 51.6% were single viral infections, with the causative agent mostly being RSV or human rhinovirus (HRV) (23). The rise in the proportion of viral CAP may be attributed to the introduction of pneumococcal conjugate vaccines, which have led to a decline in CAP caused by Streptococcus pneumoniae (24). Simultaneously, the clinical application of polymerase chain reaction technology has enhanced the detection rate of viral pathogens and facilitated the identification of new virus (25). Moreover, individuals with congenital heart disease, congenital airway dysplasia, history of previous major surgery, or malnutrition are at high risk for viral infection and are more likely to develop severe pneumonia (26). The combination of underlying diseases and viral infections has emerged as a significant risk factor for severe CAP in children (27).
Several studies have shown that blood inflammatory biomarkers, such as procalcitonin and CRP, can assist in differentiating between bacterial and viral CAP (28,29). In recent years, platelets have been found to detect endothelial cell damage and pathogenic microorganism invasion, forming the first line of defense against pathogenic infections (30). In addition, platelets can sense and capture pathogens and release a variety of bioactive substances after activation, such as growth factors, cytokines and chemokines, which are important immune modulators and inflammatory mediators (31). Currently, an increasing number of studies are focusing on the role of platelet parameters in the diagnosis of infectious diseases and the prediction of disease progression (12,32).
In this study, we further explored the role of platelet parameters in the diagnosis of viral and bacterial infections in children with severe CAP, and found that PLT levels at 72 h of admission were significantly higher in the bacterial and virus-bacterial mixed group than in the viral group. Accordingly, the dynamic curve of ΔPLT at 72 h of admission was significantly elevated in the bacterial and virus-bacterial mixed groups, but not in the viral group. Bacteria and viruses can affect platelet function in different ways. Bacterial endotoxins like lipopolysaccharide (LPS) can activate platelets and promote a prothrombin phenotype (33), while RNA viruses can enhance platelet adhesion to infected endothelial cells (34), interfere with the immune system (35), and decrease thrombopoietin (TPO) production in the liver (36), leading to a decrease in circulating platelet count.
Due to factors such as early antibiotic use, sample collection, and transportation, the positive rate of culture is only about 60% (37). In the absence of culture results, doctors usually make decisions based on clinical manifestations, imaging examinations, and other laboratory test results. The AUC of PLT for distinguishing bacterial from viral infections was found to be 0.6–0.7, indicating a lack of accuracy. Although the 72-h predictive indicators may not be satisfactory, they are still expected to be combined with other indicators to provide a reference for timely adjustment of treatment.
The shortcomings of this study are as follows: (I) a single-center retrospective study with a small number of cases, which may have led to data bias. The findings of this study need to be confirmed in prospective, large sample, multicenter studies. (II) Due to the scarcity of severe mycoplasma pneumonia cases admitted to PICU during the study period, they were not included in this research. (III) The inclusion of platelet activation biomarkers may further improve the application of platelets in the differential diagnosis and prognostic assessment of pathogens.
Conclusions
Dynamic monitoring of platelet parameters in severe CAP children showed that PLT after 72 h of admission was significantly lower in the viral infection group than in the bacterial-involved infection group (including bacterial and viral-bacterial mixed infection). Although predictors such as PLT or PLT combined with CRP and procalcitonin at this time could assist in differentiating bacterial-involved and viral infections, their sensitivity and specificity are not ideal. Further research is needed to verify its accuracy and clinical application value.
Acknowledgments
The authors of this paper would like to thank all nurses and doctors in PICU of Beijing Children’s Hospital.
Funding: This study was supported by pediatric special project from
Footnote
Reporting Checklist: The authors have completed the STROBE reporting checklist. Available at https://tp.amegroups.com/article/view/10.21037/tp-23-441/rc
Data Sharing Statement: Available at https://tp.amegroups.com/article/view/10.21037/tp-23-441/dss
Peer Review File: Available at https://tp.amegroups.com/article/view/10.21037/tp-23-441/prf
Conflicts of Interest: All authors have completed the ICMJE uniform disclosure form (available at https://tp.amegroups.com/article/view/10.21037/tp-23-441/coif). The authors have no conflicts of interest to declare.
Ethical Statement: The authors are accountable for all aspects of the work in ensuring that questions related to the accuracy or integrity of any part of the work are appropriately investigated and resolved. The study protocol was reviewed and approved by the Ethics Committee of Beijing Children’s Hospital (No. 2018-k-103). The study was conducted in accordance with the Declaration of Helsinki (as revised in 2013). Patient informed consent was waived due to the retrospective nature of the study.
Open Access Statement: This is an Open Access article distributed in accordance with the Creative Commons Attribution-NonCommercial-NoDerivs 4.0 International License (CC BY-NC-ND 4.0), which permits the non-commercial replication and distribution of the article with the strict proviso that no changes or edits are made and the original work is properly cited (including links to both the formal publication through the relevant DOI and the license). See: https://creativecommons.org/licenses/by-nc-nd/4.0/.
References
- Nascimento-Carvalho CM. Community-acquired pneumonia among children: the latest evidence for an updated management. J Pediatr (Rio J) 2020;96:29-38. [Crossref] [PubMed]
- Li S, Xu Y, Wu Y, et al. Heparin-Binding Protein: A Prognostic Biomarker Associated with Severe or Complicated Community-Acquired Pneumonia in Children. J Inflamm Res 2023;16:321-31. [Crossref] [PubMed]
- Rueda ZV, Aguilar Y, Maya MA, et al. Etiology and the challenge of diagnostic testing of community-acquired pneumonia in children and adolescents. BMC Pediatr 2022;22:169. [Crossref] [PubMed]
- Ruuskanen O, Lahti E, Jennings LC, et al. Viral pneumonia. Lancet 2011;377:1264-75. [Crossref] [PubMed]
- Principi N, Esposito S. Biomarkers in Pediatric Community-Acquired Pneumonia. Int J Mol Sci 2017;18:447. [Crossref] [PubMed]
- Thomas J, Pociute A, Kevalas R, et al. Blood biomarkers differentiating viral versus bacterial pneumonia aetiology: a literature review. Ital J Pediatr 2020;46:4. [Crossref] [PubMed]
- Bakogiannis C, Sachse M, Stamatelopoulos K, et al. Platelet-derived chemokines in inflammation and atherosclerosis. Cytokine 2019;122:154157. [Crossref] [PubMed]
- Wei Y, Feng J, Ma J, et al. Characteristics of platelet-associated parameters and their predictive values in Chinese patients with affective disorders. BMC Psychiatry 2022;22:150. [Crossref] [PubMed]
- Ozcelik N, Ozyurt S, Yilmaz Kara B, et al. The value of the platelet count and platelet indices in differentiation of COVID-19 and influenza pneumonia. J Med Virol 2021;93:2221-6. [Crossref] [PubMed]
- Zhu Q, Zhou J, Li F, et al. Nasopharyngeal aspirates in children with severe community-acquired pneumonia collected within 3 days before bronchoscopy can partially reflect the pathogens in bronchoalveolar lavage fluids. BMC Infect Dis 2022;22:814. [Crossref] [PubMed]
- Khodadi E. Platelet Function in Cardiovascular Disease: Activation of Molecules and Activation by Molecules. Cardiovasc Toxicol 2020;20:1-10. [Crossref] [PubMed]
- Li C, Li J, Ni H. Crosstalk Between Platelets and Microbial Pathogens. Front Immunol 2020;11:1962. [Crossref] [PubMed]
- Arbesu I, Bucsaiova M, Fischer MB, et al. Platelet-borne complement proteins and their role in platelet-bacteria interactions. J Thromb Haemost 2016;14:2241-52. [Crossref] [PubMed]
- Pocket Book of Hospital Care for Children: Guidelines for the Management of Common Childhood Illnesses. 2nd ed. Geneva: World Health Organization; 2013.
- Nasrin S, Tariqujjaman M, Sultana M, et al. Factors associated with community acquired severe pneumonia among under five children in Dhaka, Bangladesh: A case control analysis. PLoS One 2022;17:e0265871. [Crossref] [PubMed]
- Voiriot G, Visseaux B, Cohen J, et al. Viral-bacterial coinfection affects the presentation and alters the prognosis of severe community-acquired pneumonia. Crit Care 2016;20:375. [Crossref] [PubMed]
- Mangalesh S, Dudani S, Malik A. Platelet Indices and Their Kinetics Predict Mortality in Patients of Sepsis. Indian J Hematol Blood Transfus 2021;37:600-8. [Crossref] [PubMed]
- Carter JV, Pan J, Rai SN, et al. ROC-ing along: Evaluation and interpretation of receiver operating characteristic curves. Surgery 2016;159:1638-45. [Crossref] [PubMed]
- Ng WW, Lam SM, Yan WW, et al. NLR, MLR, PLR and RDW to predict outcome and differentiate between viral and bacterial pneumonia in the intensive care unit. Sci Rep 2022;12:15974. [Crossref] [PubMed]
- Lubell Y, Blacksell SD, Dunachie S, et al. Performance of C-reactive protein and procalcitonin to distinguish viral from bacterial and malarial causes of fever in Southeast Asia. BMC Infect Dis 2015;15:511. [Crossref] [PubMed]
- Safarika A, Wacker JW, Katsaros K, et al. A 29-mRNA host response test from blood accurately distinguishes bacterial and viral infections among emergency department patients. Intensive Care Med Exp 2021;9:31. [Crossref] [PubMed]
- Cao L, Ji Z, Zhang P, et al. Epidemiology and mortality predictors for severe childhood community-acquired pneumonia in ICUs: A retrospective observational study. Front Pediatr 2023;11:1031423. [Crossref] [PubMed]
- Tramper-Stranders GA. Childhood community-acquired pneumonia: A review of etiology- and antimicrobial treatment studies. Paediatr Respir Rev 2018;26:41-8. [Crossref] [PubMed]
- Jansen KU, Gruber WC, Simon R, et al. The impact of human vaccines on bacterial antimicrobial resistance. A review. Environ Chem Lett 2021;19:4031-62. [Crossref] [PubMed]
- Berastegui-Cabrera J, Aguilar-Guisado M, Crespo-Rivas JC, et al. Prepandemic viral community-acquired pneumonia: Diagnostic sensitivity and specificity of nasopharyngeal swabs and performance of clinical severity scores. J Med Virol 2023;95:e28317. [Crossref] [PubMed]
- Ding XF, Zhang B, Zhong LL, et al. Viral etiology and risk factors for severe community-acquired pneumonia in children. Zhongguo Dang Dai Er Ke Za Zhi 2012;14:449-53.
- Dean P, Florin TA. Factors Associated With Pneumonia Severity in Children: A Systematic Review. J Pediatric Infect Dis Soc 2018;7:323-34. [Crossref] [PubMed]
- Wrotek A, Robakiewicz J, Pawlik K, et al. The Etiology of Community-Acquired Pneumonia Correlates with Serum Inflammatory Markers in Children. J Clin Med 2022;11:5506. [Crossref] [PubMed]
- Li F, Kong S, Xie K, et al. High ratio of C-reactive protein/procalcitonin predicts Mycoplasma pneumoniae infection among adults hospitalized with community acquired pneumonia. Scand J Clin Lab Invest 2021;81:65-71. [Crossref] [PubMed]
- van der Meijden PEJ, Heemskerk JWM. Platelet biology and functions: new concepts and clinical perspectives. Nat Rev Cardiol 2019;16:166-79. [Crossref] [PubMed]
- Maouia A, Rebetz J, Kapur R, et al. The Immune Nature of Platelets Revisited. Transfus Med Rev 2020;34:209-20. [Crossref] [PubMed]
- Pogorzelska K, Krętowska A, Krawczuk-Rybak M, et al. Characteristics of platelet indices and their prognostic significance in selected medical condition - a systematic review. Adv Med Sci 2020;65:310-5. [Crossref] [PubMed]
- Lê VB, Schneider JG, Boergeling Y, et al. Platelet activation and aggregation promote lung inflammation and influenza virus pathogenesis. Am J Respir Crit Care Med 2015;191:804-19. [Crossref] [PubMed]
- Martyanov AA, Maiorov AS, Filkova AA, et al. Effects of bacterial lipopolysaccharides on platelet function: inhibition of weak platelet activation. Sci Rep 2020;10:12296. [Crossref] [PubMed]
- Ojha A, Bhasym A, Mukherjee S, et al. Platelet factor 4 promotes rapid replication and propagation of Dengue and Japanese encephalitis viruses. EBioMedicine 2019;39:332-47. [Crossref] [PubMed]
- Antoniak S, Mackman N. Platelets and viruses. Platelets 2021;32:325-30. [Crossref] [PubMed]
- Miethke M, Pieroni M, Weber T, et al. Towards the sustainable discovery and development of new antibiotics. Nat Rev Chem 2021;5:726-49. [Crossref] [PubMed]