Randomized controlled trial comparing the impacts of Saccharomyces boulardii and Lactobacillus rhamnosus OF44 on intestinal flora in cerebral palsy rats: insights into inflammation biomarkers and depression-like behaviors
Highlight box
Key findings
• Lactobacillus rhamnosus (L. rhamnosus) OF44 intervention reduces the inflammation level of cerebral palsy (CP) rats. The intervention also has the potential to change the gut microbial composition and metabolic functions of CP rats.
What is known and what is new?
• Saccharomyces boulardii (S. boulardii) intervention can alleviate the depressive state, reduce inflammation, and alter the gut microbial of rats with CP.
• L. rhamnosus OF44, a gut microbial strain, demonstrated the same affects as those of S. boulardii in reducing inflammation levels.
What is the implication, and what should change now?
• Our findings indicate that the use of probiotics can alleviate inflammation in CP rats and that these probiotics can potentially be used to treat CP in humans.
Introduction
Cerebral palsy (CP) is a unique type of neurological disorder that degrades an individual’s ability to move and maintain equilibrium and posture (1). CP is commonly triggered via brain injuries or malformation before, during, or after birth (2). Symptoms include impaired motor skills and coordination, muscle tightness or spasticity, difficulty with speech and communication, and impaired balance and coordination. Different gut microbiomes have different effects on general health, as well as diseases. For example, there is evidence of prebiotics being able to reduce the amount of cytokines in humans as well as animals; however, only a few studies suggest that prebiotics can enhance immune system functions (3). As for patients with CP, studies suggest that excessive Prevotella may be a biomarker for CP (4), and Prevotella is related to inflammation (5), suggesting that distinctive gut microbiota of CP patients contribute to their high levels of cytokines. Though CP has negative effects on both the patients and their caregivers (6), there currently exists no curative treatment for CP; however, therapy and treatment have been shown to alleviate the symptoms, including acceptance and commitment therapy, action observations, and bimanual training, among about 20 other treatments (4,7). Although a comprehensive understanding of diseases helps to improve treatment regimen, the etiology and pathophysiology of CP remain largely unknown despite considerable research efforts. Recent evidence suggests that gut microbiota contributes to the progression of CP (8), but the exact mechanisms and pathways remain largely elusive.
Lactobacillus rhamnosus (L. rhamnosus) OF44 is a selected strain of human gut bacteria. Gut microbiota has important effects in the general health of human, and it plays a role in the development of many kinds of diseases, for example, cancer (9) and neurological disorders (10). L. rhamnosus OF44 was originally used for the therapy and prevention of diseases triggered via reproductive tract dysbiosis or bone loss (11). In a study on L. rhamnosus OF44 as an intervention for bone loss, it was found that this strain exerted immunomodulatory effects, similar to many other strains of Lactobacillus (12-17). Since both brain disorders and metabolic immune diseases are related to inflammation (18), we attempted to use L. rhamnosus OF44 to modify the gut microbiota of CP rats and assess its impact on CP.
We hypothesized that the manipulation of the gut microbiota by L. rhamnosus OF44 can improve the general conditions of CP. Differences in cytokine levels and gut microbiota composition have been observed between rats with CP and healthy ones. It has also been reported that the administration of Saccharomyces boulardii (S. boulardii) can improve bowel status, reduce depression-like behavior, lower the hyperactivity of the hypothalamic-pituitary-adrenal (HPA) axis, and modify the gut microbiome of hemiplegic spastic CP rats. However, despite the identified effects of S. boulardii, its unpredictable and less pronounced impact on gut microbiota of CP mice suggests it may not be the ideal microbe for CP treatment (19). Additionally, given the proven efficacy of L. rhamnosus OF44 in treating and preventing osteoporosis and reproductive tract disorders, which both involves inflammation and changes in microbiota (20,21), exploring its role in CP opens avenues for investigating other potentially more effective treatments for CP, as well as the full clinical potential of L. rhamnosus OF44. To this end, we used a rat model of CP and behavioral experiments, enzyme-linked immunosorbent assay (ELISA), and 16S ribosomal RNA (rRNA) sequencing to compare the motor symptoms, depressive states, cytokine levels, and gut microbiomes among groups administered with different interventions. Comparison of the results obtained from the control groups and those treated with S. boulardii could provide a comprehensive evaluation of the efficacy of L. rhamnosus OF44 intervention in CP. The current animal study is crucial in addressing the research question as it delves into the efficacy of L. rhamnosus OF44 in the context of CP by elucidating the distinct impact of L. rhamnosus OF44 on inflammation, behavior, and gut microbiota. The proposed outcomes serve as meaningful indicators of the potential effectiveness of L. rhamnosus OF44 for CP because various factors contributing to the development of CP, for instance, gut microbiota and inflammation, are addressed. Collectively, our findings contribute novel insights into the potential function of the gut microbiota in CP and may further lead to the innovation of therapeutic strategies for the management or prevention of this disorder. We present this article in accordance with the ARRIVE reporting checklist (available at https://tp.amegroups.com/article/view/10.21037/tp-23-566/rc).
Methods
We employed a randomized parallel controlled trial design with simple randomization, using 18 CP model rats and 6 healthy rats as experimental subjects. We estimated the number of rats used with references of other studies in the same area (8,19). Simple randomization ensures that each rat has an equal chance of being assigned to each group. This minimizes the bias of selection in the experiment. By choosing to estimate sample size with accordance to other studies on CP and gut microbiota, our study is aligned with existing research, and the validity of our study is enhanced. Blood and stool samples are collected before the intervention (0 day), 1 week (7 days), 2 weeks (14 days), 3 weeks (21 days), and 4 weeks after the intervention (28 days). On day 0, three CP rats (1 in group A, 1 in group B, and 1 in group C) were euthanized, along with six control rats. The contents in the ceca were gathered and frozen at −80 ℃, while the colon tissues (cleared of contents) and brain tissues were fixed in formalin at room temperature for preservation. On day 14, six CP rats (2 in group A, 2 in group B, and 2 in group C) were euthanized, with the same procedures being performed as those on day 0. Blinding procedures were implemented to minimize potential bias in the experiment. Personnel who were involved in data collection, outcome assessments, and data analysis were kept unaware of the group assignments. A protocol was prepared before the study without registration.
Animals
All animals used in the experiment were acquired from Zhuhai Bestest Biotechnology Co., Ltd. (Zhuhai, China), and kept in a specific pathogen-free animal laboratory by Shenzhen TOP Biotechnology Co., Ltd. (Shenzhen, China). The animal study protocol was approved by the Institutional Review Board of Department of Pediatrics of Longgang District Maternity & Child Healthcare Hospital of Shenzhen City (ethical approval number: LGFYYXLLLQ-2020-002), in compliance with the Chinese national standards GB14925 and GB14922, as well as the standard operating procedures of Shenzhen TOP Biotechnology Co., Ltd., for the care and use of animals. During the study period, all rats were subjected to uniform management measures, such as drug adjustment, nutritional intervention, and standardized lifestyle. At 100 days of age, 12 male and 12 female specific pathogen-free grade Sprague Dawley (SD) rats were assigned to a CP group (n=18) or a control group (n=6) at random. These rats were all kept inside a feeding space at 22±1 ℃ and 50–70% moisture under a single 12-hour light-dark cycle to avoid confounding variables.
Modeling of CP
CP was modeled in neonatal rats through left carotid artery ligation, and 3- to 7-day-old rats were randomly grouped. The surgical procedure involved anesthetizing the 7-day-old neonatal rats with isoflurane, fixing them in a supine position, and disinfecting the skin with 75% alcohol. A midline incision was created in the neck, the left carotid artery was isolated using vascular clamps, double-ligation with No. 7 sterile sutures, and cutting off of the vessels. A gelatin sponge was used for hemostasis during surgery, which was followed by wound closure and re-disinfection of the skin. The entire surgical procedure was completed within 10 minutes.
After surgery, rats were subjected to rewarming inside a water bath at a temperature of 37 ℃. They were allowed to rest in a cage for 2–4 hours before being placed into a sealed hypoxia chamber maintained at a 37 ℃ temperature, which was filled with a gas mixture containing 92% nitrogen and 8% oxygen (flow rate 0.5 L/min; humidity 70%±5%). Rats had reached 100 days of age when the experiment was started. During the 1-hour hypoxic period, observation was made at least every 30 minutes to monitor respiratory distress. If respiratory distress was observed, the rat was immediately transferred to a warming box. After modeling surgery, the rats were kept warm and fed milk.
L. rhamnosus OF44 and S. boulardii preparation
The rats in the model group were split into three groups at random: group A, group B, and group C. To generate data acquired from rats to humans, dosages of gavage are adjusted for rats according to the different surface areas of the two species. Rats in the group A were administered L. rhamnosus [109 colony-forming units (CFU)/day; total 0.2 mL], rats in group B were administered S. boulardii (107 CFU/day; total 0.2 mL), and rats in group C were given normal saline as placebo. In addition, there was a control group of 6 healthy rats that were euthanized on day 0 of the experiment after blood and stool samples were obtained. L. rhamnosus OF44 was prepared by adding the frozen L. rhamnosus OF44 bacterial solution to MRS liquid medium. The frozen L. rhamnosus OF44 solution was cultured at 37 ℃ for 24 hours and then centrifuged at 8,000 g for roughly 3 min, with the subsequent supernatant being discarded. The remaining cell pellet was rinsed with sterile phosphate-buffered saline (PBS) three times. Finally, the remaining L. rhamnosus OF44 was diluted with sterile PBS to obtain a bacterial suspension of 1×109 CFU/mL. After adaptive feeding, the rats were orally gavaged with bacterial solution, at a dose of 1×109 CFU per rat per day, for a total of 4 weeks (28 days). S. boulardii was prepared by adding commercial S. boulardii sachets into a solution at a concentration of 0.05 g/mL (1×107 CFU/mL). After adaptive feeding, the rats were orally gavaged with bacterial solution at a dose of 1×107 CFU per rat per day for a total of 4 weeks (28 days).
Behavioral evaluation of the models
Two behavioral tests were implemented in this study for behavioral evaluation of the rat models: the Y-maze experiment and the tail suspension test. The Y-maze test involves a three-arm maze apparatus in the shape of a “Y”, in which each arm is identical in size and shape. In our study, a nontransparent organic plastic sheet was used to create the shape of the maze, which consisted of three arms (35 cm in length, 10 cm in width, and 20 cm in height) at angles of 120º between each arm. The rat was placed at the end of 1 arm and allowed to freely roam the maze. The sequence by which the rats entered each arm was recorded.
The tail suspension test is a common and rapid means of evaluating the depressive state of model animals. In this study, it was used to determine whether there was a difference in the depressive states between CP rats and healthy rats. In the tail suspension test, the rat is hung by its tail, which prevents it from escaping and causes it to enter a unique state of immobility that indicates a depressive state. The experiment records the time of immobility as indicator of the depressive state. The rat is suspended 20–25 cm away from the working table, with the posterior one-third of its tail fixed on the bracket with adhesive tape. In our study, the rat was considered immobile only when it was completely motionless for 6, and the immobility time was recorded by an experimenter who was unaware of the study’s purpose in 6 minutes.
Measurement of serum inflammatory cytokines
Plastic tubes were used to collect blood samples, from which the serum was obtained through centrifugation at 3,000 g for 10 minutes at a temperature of 4 ℃. The obtained serum was conserved at −80 ℃ for subsequent analysis. To minimize the impact of the circadian rhythm of the HPA axis on the results, all samples within each group were gathered in the same time window (from 10:00 AM to 12:00 AM). Concentrations of interleukin (IL)-1β, IL-6, IL-8, and tumor necrosis factor alpha (TNF-α) inside the serum samples were measured via commercial ELISA kits (product codes: MM-0047R1, MM-0190R1, MM-0175R2, MM-0180R2) by Jiangsu Meimian Industrial Co., Ltd. according to the producer’s instructions. Plates were read by 1 ELISA reader at a 450-nm wavelength. The sensitivity of the kit for IL-1β was 0.1 pg/mL, while that for the IL-6, IL-8, and TNF-α kits was 1.0 pg/mL.
16S rRNA sequencing of the rats
Sample collection
On days 0, 7, 14, and 21, 2 fresh fecal specimens were gathered from each rat using a 1.5-mL Eppendorf (EP) tube and frozen at −80 ℃ instantly until examination.
DNA extraction and polymerase chain reaction (PCR) amplification
Microbial community genomic DNA was extracted from the fecal specimens using the E.Z.N.A. Soil DNA Kit (Omega Bio-tek, Norcross, GA, USA) with TaKaRa Premix Taq Version 2.0 (Takara Bio, Shiga, Japan) according to the manufacturers’ instructions. The extracted DNA was examined on 1% agarose gel. Additionally, the DNA concentration and purity were examined using a NanoDrop 2000 UV-vis spectrophotometer (Thermo Fisher Scientific, Waltham, MA, USA). 16S rRNA sequencing was performed to identify bacterial diversity using the 515F and 806R primers targeting the V4 region. In addition, 528F and 706R primers targeting the V4 region of the 18S rRNA gene were adopted to characterize the diversity of the eukaryotic microbes, while ITS5-1737F and ITS2-2043R primers targeting the ITS1 region were used to characterize the fungal diversity. Furthermore, regions 16S V3–V4/16S V4–V5, archaeal 16S V4–V5, 18S V5, and ITS2 were amplified. Functional gene-specific primers were also implemented in our analysis.
PCR was completed using a BioRad S1000 thermal cycler (Bio-Rad Laboratories, Hercules, CA, USA). PCR amplification for the 16S rRNA gene was performed as follows: initial denaturation at 94 ℃ for 5 minutes, 30 cycles of denaturation at 94 ℃ for 30 s, annealing at 52 ℃ for 30 s, extension at 72 ℃ for 30 s, and a single extension at 72 ℃ for 10 minutes finishing at 4 ℃. The PCR mixtures comprised 25 µL of 2× Premix Taq, excessive deoxynucleotide triphosphates (dNTPs), 1 µL of forward primer (10 µM), 1 µL of reverse primer (10 µM), 0.4 µL of TransStart FastPfu DNA Polymerase, 50 ng of template DNA, and up to 50 µL of double-distilled water (ddH2O). PCRs were implemented in triplicate. The product of PCR was derived from a 1% agarose gel and purified via the E.Z.N.A. Gel Extraction Kit (Omega Bio-tek) and quantified via Quantus Fluorometer (Promega, Madison, WI, USA).
Illumina Nova 6000 sequencing
Purified amplicons were gathered in equimolar concentrations and paired-end sequenced (2×300) on an Illumina Nova 6000 platform (Illumina, San Diego, CA, USA) according to standard protocols of Majorbio Bio-Pharm Technology Co., Ltd. (Shanghai, China).
Processing of sequencing data
For the analysis of 16S rRNA gene sequencing figures, fastp software (version 0.23.2; https://github.com/OpenGene/fastp) was used to acquire clean reads and to remove primers based on the barcode information. Then, the fastq_mergepairs command in USearch (v.10; http://www.drive5.com/usearch/) was adopted with the objective of merging the paired-end clean reads with a minimal overlap, a length of 16 bp, and a maximal mismatch of 5 bp in the overlap region. Raw tags were filtered using fastp software to generate clean tags. Operational taxonomic units (OTUs) were generated at a 97% similarity cutoff via UPARSE (version 7.1; http://drive5.com/uparse/), and chimeric sequences were deleted. The Majorbio Cloud Platform (www.majorbio.com) was employed for data analysis. Barcodes and primers were used to distinguish samples, and sequence directions were regulated through exact barcode matching and 2-nucleotide mismatch in the primer matching.
Statistical analysis
Analysis of behavioral experiments and serum inflammatory cytokine levels
The original data of the Y-maze test is converted to alteration rates before statistical analysis. Then, the data of both the tail suspension test and the Y-maze test are evaluated using t-tests in Excel. The serum inflammatory cytokine optical density values are first regressed on linear functions for concentration using the equations provided in the instructions of the experimental kits. Then, t-tests are used to compare the concentrations of the cytokines among different treatment groups, and across the timeline.
Analysis of sequencing data
Alpha diversity and Beta diversity are used to analyze the sequencing data. They are two indicators of the diversity of an ecosystem, in this case the gut microbial of rats. Three indicators are used to assess alpha diversity, which are Chao index, Shannon diversity, and Simpson diversity. Chao index assesses diversity based on whether a species exist in the sample. Shannon diversity is based on the uniqueness of a species, and Simpson diversity is based on the total number of individuals of a species. In contrast to alpha diversity, beta diversity assesses the dissimilarities among samples. Heatmap is used to present beta diversity, where different colors represent the similarities and differences among samples.
Results
Establishment of the CP rat model
To establish the CP rat model, we employed right common carotid artery ligation (22). This ligation induced ischemia in the rat, which caused neuronal death and glial cell reaction, mimicking the process of CP in humans. To support the validity of the model, we captured and recorded the surgical procedure and provided clear photos of the ligated right neck region of the rats (Figure 1).
These models exhibited significant motor and cognitive impairments consistent with symptoms of human CP. These data provide strong evidence for our study, indicating that we successfully established a rat model of CP.
Behavioral experiment analysis
Tail suspension experiments have been used to indicate when the emotional state of mice with CP has become depressive (19). We aimed to investigate whether the emotional state of rats is also affected by CP, so we conducted tail suspension experiments on rats before the probiotic intervention was initiated. The tail suspension test is an extensively used method for evaluating anxiety and depression in rodents (23). The tail suspension test was conducted on day 0 of the intervention to assess the emotional states of rats. In this test, the rats were suspended by their tails for a certain period of time, and their immobility time was measured as an indicator of depression. We hypothesized that the CP rats would show more immobility time than would the healthy rats, reflecting their higher level of depression, as in CP mice (19). However, the tail suspension test did not result in a statistically significant between the depressive states of healthy and CP rats. Further studies are needed to explore possible explanations for these results.
After the probiotic intervention concluded, we verified whether the intervention had an impact on the spatial memory and learning of rats, which are among the main functions affected by CP, as reported in children (24,25). We thus conducted the Y-maze test on the last day of the intervention to assess the spatial memory and learning in rats. Both traits are highly correlated with CP. The test provides information on a mouse’s ability to recognize and remember new environments, which is useful in evaluating neurological impairments associated with CP (26). The Y-maze test was chosen for two reasons: (I) to inspect the CP-related movement conditions in intervened rats; and (II) to avoid repeating the tail suspension test since rats have the memory of previously conducted tests, which could affect the results. The test results suggested that after the intervention, the correct conversion rates of all three groups of CP rats were lower than those of healthy rats. The correct conversion rates of the group A rats were the lowest. In addition, the correct conversion rates of group C rats were higher than those of both the group A rats and group B rats. However, no statistical significance was observed (Figure 2).
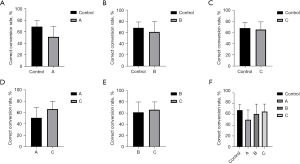
L. rhamnosus OF44 and S. boulardii exerted anti-inflammatory effects in CP rats
A previous study has suggested that neurological diseases such as CP lead to increased inflammation levels (27). Inflammation has been shown to cause increase in oxidative stress and disruption in the blood-brain barrier, both of which are common impairments in CP (28). Given that high inflammation levels can manifest as an increase in cytokines, such as IL (29), we tested whether interventions with L. rhamnosus OF44 and S. boulardii could affect the production of cytokines and thus reflect the inflammation level in rats with CP. We isolated the serum from the blood samples and evaluated the amount of cytokines, specifically that of IL-1β, IL-6, IL-8, and TNF-α, to assess the effects of L. rhamnosus OF44 and S. boulardii on the induction of inflammation. We found there to be a significant increase in the IL-1β level in group C rats on day 28 compared with day 14 (P<0.05). This indicated that the IL-1β level was increased in rats experiencing CP without intervention over time. Furthermore, the levels of IL-1β were not significantly different among different groups or across different time spans of groups A and B (Figure 3).
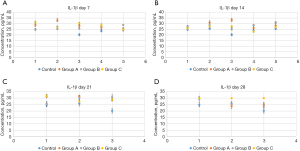
Compared with those in group C, IL-6 concentrations were reduced significantly in group A (P<0.01) on day 21 of the intervention. In addition, on day 28 of the intervention, there was a significant rise in the IL-6 level in rats from group A in comparison with those of days 0, 7, and 14. We observed similar results in the group B rats: on day 28 of the intervention, there was also a significant rise in the IL-6 level in rats from group B in comparison with the levels on days 0, 7, and 14. No other significant changes were found. This suggested that interventions with L. rhamnosus OF44 had some effect in reducing the level of IL-6. In comparison, S. boulardii showed less impact on reducing the amount of IL-6 in CP rats. Furthermore, the IL-6 level in group C rats showed a significant increase during the course of the intervention, which points to an increase in the IL-6 level over time in rats with CP. The results also thus imply that the interventions with both L. rhamnosus OF44 and S. boulardii may potentially slow the increase of IL-6 levels (Figure 4).
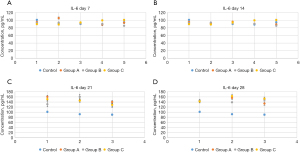
There was a significant difference in the IL-8 level between the control group and CP groups before the intervention (P<0.05). This indicated that CP rats had higher IL-8 levels than did healthy rats, supporting the idea of an increased inflammation level in rats with CP. In addition, a significant difference was observed in the IL-8 levels between group A and group B rats on day 21 of intervention (P<0.05). The level of IL-8 in group B rats was obviously lower than that in group A rats, suggesting that S. boulardii intervention was more effective in lowering IL-8 levels than was L. rhamnosus OF44 intervention. Moreover, for group A rats, on day 21 and day 28 of the intervention, the IL-8 levels were significantly different from those of days 0, 7, and 14 (P<0.05), decreasing as time elapsed. This suggested that intervention with L. rhamnosus OF44 was also effective in lowering IL-8 levels, and its effects were more stable and long lasting. Additionally, the IL-8 level in group B rats was significantly different between day 0 and day 7; between day 21 and days 0, 7, and 14; between day 0 and day 28; and between day 28 and day 21. These results suggested that overall, the intervention of S. boulardii reduced the IL-8 levels in rats, but the intervention seemed to be less stable than that of the L. rhamnosus OF44 intervention (Figure 5).
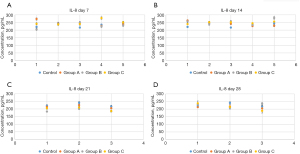
On day 14 of the intervention, group B rats exhibited an evident decline in TNF-α concentration compared to group C rats (P<0.05). This suggests that the S. boulardii intervention had the ability to decrease the TNF-α level in CP rats. In addition, on day 21 of the intervention, the TNF-α level in the group A rats was obviously less than that of the group B rats (P<0.05). This implies that on day 21, L. rhamnosus OF44 exhibited a stronger ability to alleviate inflammation than did S. boulardii. In group A, the TNF-α levels on day 21 and day 28 were markedly reduced compared with those on day 0 (P<0.01). This indicates that L. rhamnosus OF44 intervention had some effect in reducing the amount of TNF-α. Furthermore, in group B, the TNF-α levels on day 14 and day 28 were obviously reduced compared to those on day 0 (P<0.01). This suggested that S. boulardii also has the ability to reduce TNF-α levels. Finally, compared with days 0, 7, and 14 of the intervention, the TNF-α level in group C rats reduced significantly on day 28 (P<0.05). This was an unexpected result, which might have occurred due to the limited number of rat models that we used in this study (Figure 6).
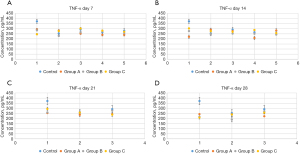
16S rRNA sequencing analysis
Alpha diversity
Chao index: there was no obvious distinction in the Chao indicator among the three groups. However, intervention was associated with a significant increase in the Chao index of gut microbiota of all three groups of CP rats (Figure 7A).
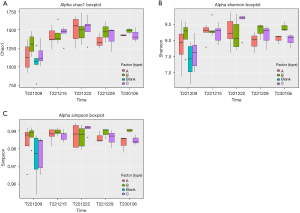
The Shannon and Simpson diversity indices exhibited similar trends. At baseline, the healthy rats had a lower Shannon diversity index score than did the CP rats. After 1 week, the CP rats had a significant increase in Shannon diversity index score. However, no obvious distinction was observed in the three CP groups. After 2 weeks, the group C rats exhibited a rising trend in Shannon diversity index score, while the scores of the group A and B rats remained relatively stable. After 3 weeks, the group C rats experienced a significant drop in Shannon diversity index score, but no obvious difference was detected across the three groups. After 4 weeks, the group C rats further declined in Shannon diversity index score. Additionally, no obvious difference was found between the three groups (Figure 7B).
Simpson diversity: the healthy rats had a lower Simpson diversity index score than did the CP rats at baseline. No obvious differences in Simpson diversity index score were noted across the three groups of CP rats at any time point (Figure 7C).
In contrast to our previous study, the alpha diversity results did not show that healthy rats had a more favorable gut microbiota or that CP rats received improved gut microbiota diversity following intervention (8).
Beta diversity
UniFrac: the dissection on the weighted UniFrac heatmap revealed the differences in gut microbiota composition between healthy rats and rats in group C at 2 and 3 weeks’ intervention. Additionally, after 1 week’s intervention, there was a noticeable difference in gut microbiota composition between groups A and B compared to the control group. Moreover, the gut microbiota composition of the group C rats at 1 week of intervention differed from that of the group B rats at 2 weeks of intervention. After 2 weeks of intervention, the difference in gut microbiota composition between groups A or B and the control group decreased but was still significant, and there was also a considerable difference between group A or B rats and group C rats in this regard. These findings indicate the following: (I) the gut microbiota composition between healthy rats and untreated CP rats is different, as observed in the UniFrac heatmap analysis; (II) the intervention with L. rhamnosus OF44 and S. boulardii showed a tendency to alter the gut microbiota composition in rats, but more pronounced results may require a longer duration of intervention (Figure 8).
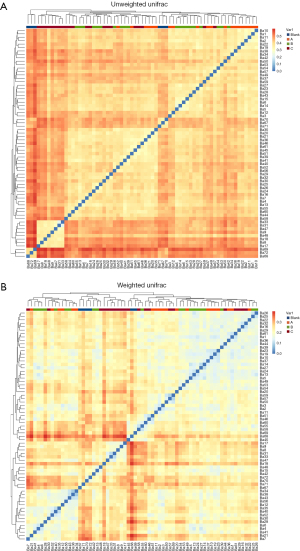
Principal co-ordinates analysis (PCoA) indicated that there existed no significant difference among the three CP groups while there is significant difference between the three CP groups and the control group. Meanwhile, there were both similarities and differences between the microbiota composition of the healthy rats and that of the CP rats. In terms of intervention time, there was no noticeable difference in the PCoA images of the four groups within 4 weeks’ intervention (Figure 9).
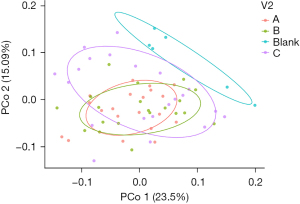
Nonmetric multidimensional scaling (NMDS) analysis revealed a significant difference in composition of gut microbiota between healthy rats and rats with CP. However, no apparent difference was observed among the other groups. After the rat CP model was created, the gut microbiota of these rats was found to be completely different from that the control group. However, the groups treated with S. boulardii and physiological saline were not significantly different in this regard (Figure 10A). In addition, the gut microbiota of rats treated with L. rhamnosus OF44 gradually changed from the early stage to the later stage (Figure 10B).
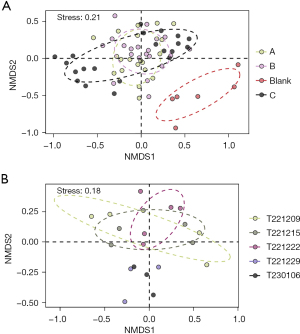
The statistical analysis of significant differences in gut microbiota
Linear discriminant analysis effect size (LEfSe) of species population characterized the genus abundance of each specimen at the level of genus. At that genus level, the feature taxa in the group A rats [linear discriminant analysis (LDA) >2] were Lachnospiraceae NK4A136 group, Desulfovibrio, and Lachnoclostridium. The feature taxa in the group B rats (LDA >2) were Roseburia, Lachnospiraceae UCG-006, and Anaerovoracaceae. The feature taxa in the group C rats (LDA >2) were Helicobacteraceae, Campylobacteria, Helicobacter, Campilobacterota, and GCA 900066575. Feature taxa in the control group rats (LDA >2) were Erysipelotrichales, Dubosiella, Coriobacteria, Actinobacteriota, Enterorhabdus, and Coriobacteriaceae UCG-002 (Figure 11).
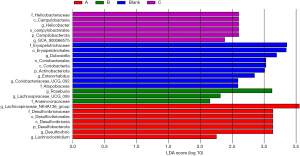
The rank-sum test was used to test hypotheses concerning the differences in microbial composition. Table 1 shows the genera that differed significantly across the samples from the different groups (P<0.05, Kruskal-Wallis test).
Table 1
Taxon name | Mean | P value | FDR | |||
---|---|---|---|---|---|---|
A | B | Blank | C | |||
g_Lachnospiraceae_NK4A136_group | 0.058498 | 0.029340 | 0.010203 | 0.028502 | 0.001871 | 0.019747 |
g_Desulfovibrio | 0.020066 | 0.014579 | 0 | 0.012477 | 0.006124 | 0.039159 |
g_Roseburia | 0.022248 | 0.023682 | 0.004398 | 0.012702 | 0.000812 | 0.012860 |
g_Treponema | 0.012635 | 0.027199 | 0 | 0.007472 | 0.001399 | 0.017375 |
g_Clostridia_UCG-014 | 0.093741 | 0.108704 | 0.163258 | 0.114673 | 0.034540 | 0.112123 |
g_(Eubacterium)_xylanophilum_group | 0.017205 | 0.013593 | 0.006758 | 0.009861 | 0.002359 | 0.023704 |
g_Alistipes | 0.011792 | 0.010228 | 0.016478 | 0.005330 | 0.006724 | 0.039409 |
g_Prevotellaceae_NK3B31_group | 0.003611 | 0.004154 | 0 | 0.005193 | 0.006545 | 0.039409 |
g_Bacteroides | 0.007134 | 0.011833 | 0.029125 | 0.022835 | 0.030505 | 0.102168 |
g_Alloprevotella | 0 | 2.59e−07 | 0 | 0.010590 | 0.000867 | 0.012861 |
Group A, Lactobacillus rhamnosus OF44 treatment group; group B, Saccharomyces boulardii treatment group; group C, physiological saline treatment group; Blank, healthy control group. FDR, false discovery rate.
At the level of phylum, the groups showed differences in Lachnospiraceae NK4A136 group, Desulfovibrio, Roseburia, Treponema, Clostridia UCG-014, (Eubacterium) xylanophilum group, Alistipes, Prevotellaceae NK3B31 group, Bacteroides, Alloprevotella, Dubosiella, Helicobacter, Prevotella, Lachnospiraceae UCG-006, Escherichia-Shigella, (Eubacterium) siraeum group, Monoglobus, (Eubacterium) ruminantium group, Candidatus Saccharimonas, Turicibacter UCG-009, Romboutsia, Colidextribacter, Elusimicrobium, Lachnoclostridium, Enterorhabdus UCG-007, Rikenellaceae RC9 gut group, Coriobacteriaceae UCG-002, GCA-900066575, (Eubacterium) nodatum group, Lachnospiraceae ND3007 group, Paludicola, Intestinimonas, Papillibacter, Lactococcus, Muribaculum, Fournierella, Family XIII UCG-001, Peptococcus, Mycoplasma, Butyricimonas, Lachnospiraceae NK4B4 group, Tyzzerella, Marvinbryantia, Rothia, Harryflintia, Acetitomaculum, Anaerofustis, (Eubacterium) brachy group, Collinsella, Parvibacter, Sporobacter, Clostridium sensu stricto 1 UCG-008, Subdoligranulum, Proteus, Saccharimonadales, Paraprevotella, Mucispirillum, Oligella, Faecalitalea, Fusicatenibacter UCG-004, Aureimonas, Eubacterium hallii group, Shinella, Devosia, and Comamonas (Table 1).
Functional predictions
The PICRUSt (phylogenetic detection of communities through reconstructing unobserved states) technique was used to predict the functional composition of the microbiome according to the 16S rRNA sequencing results. This involved mapping the species composition information from 16S sequencing to a prebuilt table of species functional gene composition, allowing for the prediction of functional outcomes. LEfSe and the Mann-Whitney test were used to estimate the effect sizes of each component metabolic pathway (MetaCyc_pathway), Kyoto Encyclopedia of Genes and Genomes (KEGG) metabolic pathway, and KEGG orthology (KO) function on the observed gut microbial differences. This approach aimed to identify metabolic pathways that significantly contributed to the differentiation between sample groups (with a default filtering condition of LDA >2). In the LefSe analysis conducted, no differential metabolic pathways were found in the predicted enzyme commission number (EC) and KO functional databases. However, in the KEGG metabolic pathway analysis, three differential pathways were identified for the different time points of intervention in the group A rats. These pathways were P441-PWY, GLYCOLYSIS-E-D, and PWY-6588. The detailed results are as follows:
During the 1-week intervention in group A rats with CP, the P441-PWY pathway showed a significant downregulation compared to other time points. However, after 4 weeks of intervention, both the Entner-Doudoroff (ED) glycolysis pathway (GLYCOLYSIS-E-D) pathway and PWY-6588 pathway were significantly upregulated. This suggests that the intervention effects of L. rhamnosus OF44 are dependent on intervention time. The P441-PWY pathway is involved in the metabolism of neuroamino acids (30), and thus might have been inhibited or damaged. This indicated that during the 4-week intervention, the condition of CP in rats might have still been worsening. The GLYCOLYSIS-E-D pathway is involved in intracellular glycolysis and the alanine pathway, and glycolysis is a metabolic pathway that breaks down glucose into pyruvate and lactate while producing a certain amount of adenosine triphosphate (ATP). The alanine pathway facilitates the further metabolism of pyruvate into ethanol and acetic acid (31), while the PWY-6588 facilitates the fermentation of pyruvate into acetone (32) and serves as an alternative pathway to glycolysis (Figure 12).
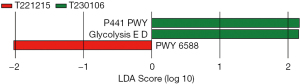
Furthermore, group A and group C showed differences in certain metabolic pathways, including P441-PWY, GLYCOLYSIS-E-D, and PWY-5505. The detailed results are as follows:
In addition to the upregulation of the P441-PWY pathway and GLYCOLYSIS-E-D pathway in group C rats, the PWY-5505 pathway was downregulated in group A rats. The PWY-5505 pathway, also known as the methylmalate pathway, is involved in the synthesis and breakdown of methylmalate in organisms (33). Methylmalate is an important intermediate metabolite that participates in various biochemical reactions, such as the tricarboxylic acid (TCA) cycle, glucose metabolism, and fatty acid metabolism (34) (Figure 13).
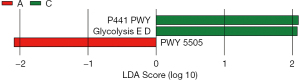
In addition, at week 4 of intervention, group C rats exhibited two differential pathways, PWY0_162 and PWY_7196 (Figure 14).
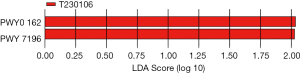
Discussion
Despite another study suggesting that CP affects the emotional states and tail suspension test results of rats (19), our study found there to revealed no obvious difference between the depressive states of rats with and without CP. This may be due to the limited number of rats used in this study. At the end of the intervention, we employed another type of behavioral experiment—the Y-maze test—to evaluate the spatial motion ability of rats. Our hypothesis was that the correct conversion rate of CP rats would be lower than that of healthy rats since due to the reduced spatial memory and learning capacity in CP rats (24,25). Additionally, we expected that intervention with probiotics would increase the correct conversion rate to a level between that of CP rats without intervention and that of healthy rats. The results suggested that the correct conversion rate of CP rats was indeed lower than that of healthy rats. However, contrary to our expectations, intervention with probiotics seemed to lower the correct conversion rate to a level lower than that of CP rats with no intervention. This may suggest that intervention with L. rhamnosus OF44 and S. boulardii lower the rats’ spatial motion ability. However, no exact conclusion can be drawn since no significant difference was observed.
Through data analysis of ELISA experiments, we found that intervention with L. rhamnosus OF44 and S. boulardii had a significant impact upon the levels of cytokines from rat serum. (I) The levels of IL-1β and IL-6 increased among rats with CP over time. (II) Interventions with both L. rhamnosus and S. boulardii had no significant effect in reducing the amount of IL-1β and IL-6 in CP rats. (III) L. rhamnosus intervention had some effect on slowing the increase of IL-6 levels. (IV) Intervention with L. rhamnosus had an effect on the IL-8 levels in rats, but a relatively long intervention time was needed. (V) Intervention of S. boulardii appeared to reduce the IL-8 levels in rats, but the intervention seemed to be unstable. (VI) L. rhamnosus OF44 intervention had some overall effect in reducing the amount of TNF-α.
Our observations regarding cytokine levels suggests that as CP progresses, cytokine levels increase in rats, supporting the notion that CP is associated with inflammation (35). In previous research, intervention with L. rhamnosus OF44 had a similar effect to that of S. boulardii in reducing cytokine levels in the serum of CP mice (19), and in our study, L. rhamnosus OF44 demonstrated a better effect compared to S. boulardii. This supports the hypothesis the intervention with L. rhamnosus OF44 can reduce cytokine levels in CP rats, alleviating their inflammatory response and relieving their CP symptoms. However, to obtain more definitive results, longer intervention periods are needed.
We further found that though another study suggests that interventions with dietary fiber, lactic acid-producing bacteria, and butyric acid-producing bacteria can significantly alter gut microbiota diversity for children with CP (8), intervention with L. rhamnosus OF44 changed the gut microbial composition of CP rats to some extent, but the overall composition and diversity of intestinal flora were not significantly changed. Analysis of 16S rRNA sequencing data revealed some differential bacterial taxa among the different groups of rats. Metabolism-related bacteria were mainly affected after both L. rhamnosus OF44 treatment and S. boulardii treatment. Prevotella was a notable example, as it had a mean relative abundance of 0 in the gut of healthy rats but a mean relative abundance of about 0.0117 in the gut of CP rats. This result was consistent with a previous report that Prevotella is a signature taxon of CP (36). Lachnoclostridium UCG 006 is a strain of bacteria that is usually found in the gut microbiome and is highly abundant in the human intestine. It has been shown to promote the function of the intestinal mucosal barrier, thereby helping to protect intestinal health. Moreover, it produces beneficial metabolites, such as propionic acid, which help maintain the stability of the intestinal environment (37). Roseburia bacteria, as a type of probiotic, is a constituent of the intestinal microbiota in the human body and is primarily found in the colon. These bacteria have the ability to produce short-chain fatty acids (SCFAs), such as acetic acid, butyric acid, and propionic acid on (38). These SCFAs contribute to maintaining gut health, regulating the immune system, and reducing inflammation (39). Research indicates that a decrease in the abundance of Roseburia bacteria is linked to several illnesses, including inflammatory bowel disease, enteritis, metabolic syndrome, obesity, and type 2 diabetes (40). Desulfovibrio bacteria are a Gram-negative sulfate-reducing bacterium primarily found inside the human digestive tract. They have the ability to use organic compounds and hydrogen gas to reduce sulfates, producing organic acids such as propionate, isobutyrate, and acetone, while also generating hydrogen sulfide (41). The presence of these bacteria suggests that treatment with L. rhamnosus OF44 and S. boulardii may improve constipation in rats with CP by influencing the metabolism of the gut microbiota.
Our sequencing analysis showed after 1 week of intervention, the PWY-6588 pathway was downregulated in the group A rats. This may indicate that the intervention with L. rhamnosus OF44 led to a downregulation of energy metabolism since PWY-6588 is the pyruvate fermentation to acetone pathway (32). After 4 weeks, the GLYCOLYSIS-E-D pathway was upregulated in the group A rats, possibly indicating that the rats gradually adapted to the intervention of L. rhamnosus, and their energy metabolism returned to a normal state. In comparison to the rats in the other groups, the group A rats showed a downregulation of the PWY-5505 pathway. PWY-5505 is also a pathway associated with energy metabolism, and its downregulation suggests a decrease in metabolic levels (33). This could be due to the impact of L. rhamnosus OF44 intervention on energy metabolism in rats with CP. Confirmation of this speculation requires further experimental validation is. In comparison to the other groups, group C showed an upregulation of both the P441-PWY pathway and the GLYCOLYSIS-E-D pathway, indicating an increase in metabolic levels (30,31). This could potentially be due to inflammation caused by CP, but additional research is needed to confirm this hypothesis.
Overall, the findings of this study support the use of treating CP with probiotics and suggest that intervention with probiotics can be an effective and feasible means to reducing the amount of cytokines, altering gut microbiota, and changing the behaviors of CP rats, especially when the intervention is long lasting. Therefore, pharmaceutical companies should consider to investing more resources and support in developing and implementing probiotics therapy in CP treatment.
However, some limitations to this study should be mentioned. First, the sample was relatively small, which limits the generalizability of results. Second, we only measured cytokines levels and conducted 16S rRNA sequencing, while only two behavioral experiments were performed; this may not capture the full range of the behaviors and symptoms of CP rats. Third, the data of the healthy control group was only measured on the first day, which might have had an impact on data analysis and comparison. Finally, the study began when the rats are 100 days old, which might have influenced the outcomes of the intervention.
To address these limitations, subsequent studies should be conducted that include larger and more representative samples, measure a more extensive array of metrics representing the health state of rats with CP, establish more complete and comprehensive control groups, and begin when the rats are younger. Moreover, future research could explore other aspects of probiotics treatment, for example, the specific mechanisms by which probiotics affect physiological activity, and examine the possibility treating CP with probiotic pharmaceuticals.
Conclusions
The CP rats in our study exhibited a higher level of inflammation than did healthy rats before intervention. After intervention, rats treated with both L. rhamnosus OF44 and S. boulardii had lower levels of cytokines in their serum. Moreover, L. rhamnosus OF44 appeared to be more efficient in lowering the levels of cytokines. In addition, L. rhamnosus OF44 and S. boulardii altered the gut microbial composition and metabolic rate of CP rats. Some altered bacteria taxa were the same as those in our previous studies concerning the link between CP and the gut microbiota. However, in contrast to other studies, the CP rats in our study did not exhibit depression-like behaviour. Overall, our findings indicate that L. rhamnosus OF44, similar to S. boulardii, has potential as a treatment for CP.
Acknowledgments
Funding: This work was supported by
Footnote
Reporting Checklist: The authors have completed the ARRIVE reporting checklist. Available at https://tp.amegroups.com/article/view/10.21037/tp-23-566/rc
Data Sharing Statement: Available at https://tp.amegroups.com/article/view/10.21037/tp-23-566/dss
Peer Review File: Available at https://tp.amegroups.com/article/view/10.21037/tp-23-566/prf
Conflicts of Interest: All authors have completed the ICMJE uniform disclosure form (available at https://tp.amegroups.com/article/view/10.21037/tp-23-566/coif). Y.Z. and Z.M. are from BGI Nutrition Precision Co., Ltd., which provided L. rhamnosus OF44 for this study. The other authors have no conflicts of interest to declare.
Ethical Statement: The authors are accountable for all aspects of the work in ensuring that questions related to the accuracy or integrity of any part of the work are appropriately investigated and resolved. The animal study protocol was approved by the Institutional Review Board of Department of Pediatrics of Longgang District Maternity & Child Healthcare Hospital of Shenzhen City (ethical approval number: LGFYYXLLLQ-2020-002), in compliance with the Chinese national standards GB14925 and GB14922, as well as the standard operating procedures of Shenzhen TOP Biotechnology Co., Ltd., for the care and use of animals.
Open Access Statement: This is an Open Access article distributed in accordance with the Creative Commons Attribution-NonCommercial-NoDerivs 4.0 International License (CC BY-NC-ND 4.0), which permits the non-commercial replication and distribution of the article with the strict proviso that no changes or edits are made and the original work is properly cited (including links to both the formal publication through the relevant DOI and the license). See: https://creativecommons.org/licenses/by-nc-nd/4.0/.
References
- Malgorzata M, Wojciech K, Alina BŁ, et al. Botulinum toxin injection as primary treatment for esotropia in patients with cerebral palsy. Klin Oczna 2013;115:13-4.
- Chen D, Huang M, Yin Y, et al. Risk factors of cerebral palsy in children: a systematic review and meta-analysis. Transl Pediatr 2022;11:556-64. [Crossref] [PubMed]
- Shokryazdan P, Faseleh Jahromi M, Navidshad B, et al. Effects of prebiotics on immune system and cytokine expression. Med Microbiol Immunol 2017;206:1-9. [Crossref] [PubMed]
- Novak I, Morgan C, Fahey M, et al. State of the Evidence Traffic Lights 2019: Systematic Review of Interventions for Preventing and Treating Children with Cerebral Palsy. Curr Neurol Neurosci Rep 2020;20:3. [Crossref] [PubMed]
- Larsen JM. The immune response to Prevotella bacteria in chronic inflammatory disease. Immunology 2017;151:363-74. [Crossref] [PubMed]
- Kamal S, Hamzaid NH, Kamaralzaman S, et al. Nutritional status as predictors for quality of life among caregivers of children with severe cerebral palsy. Transl Pediatr 2023;12:1601-18. [Crossref] [PubMed]
- Xue Y, Shi S, Zheng S, et al. Therapeutic effect of scalp-based acupuncture and moxibustion as an adjunctive treatment on children with cerebral palsy comparing to conventional rehabilitation therapy: a systematic review and meta-analysis of randomized controlled trials. Transl Pediatr 2022;11:631-41. [Crossref] [PubMed]
- Huang C, Lyu J, Chu C, et al. Dietary fiber and probiotics based on gut microbiota targeting for functional constipation in children with cerebral palsy. Front Pediatr 2022;10:1001789. [Crossref] [PubMed]
- Ohtani N, Hara E. Gut-liver axis-mediated mechanism of liver cancer: A special focus on the role of gut microbiota. Cancer Sci 2021;112:4433-43. [Crossref] [PubMed]
- Sorboni SG, Moghaddam HS, Jafarzadeh-Esfehani R, et al. A Comprehensive Review on the Role of the Gut Microbiome in Human Neurological Disorders. Clin Microbiol Rev 2022;35:e0033820. [Crossref] [PubMed]
- Zhang X, Lyu J, Zhao S, et al., inventors; Shenzhen BGI Research Center, assignee; Lactobacillus rhamnosus used to prevent and/or treat diseases caused by disturbance of reproductive tract microbiota and/or bone loss. China patent ZL202011248842.0. 2021 May 7.
- Gao D, Liu Z, Liu F, et al. Study of the immunoregulatory effect of Lactobacillus rhamnosus 1.0320 in immunosuppressed mice. Journal of Functional Foods 2021;79:104423.
- Martínez-Abad B, Garrote JA, Bernardo D, et al. Differential immunomodulatory effects of Lactobacillus rhamnosus DR20, Lactobacillus fermentum CECT 5716 and Bifidobacterium animalis subsp. lactis on monocyte-derived dendritic cells. Journal of Functional Foods 2016;22:300-12.
- Salva S, Villena J, Alvarez S. Immunomodulatory activity of Lactobacillus rhamnosus strains isolated from goat milk: impact on intestinal and respiratory infections. Int J Food Microbiol 2010;141:82-9. [Crossref] [PubMed]
- Schultz M, Linde HJ, Lehn N, et al. Immunomodulatory consequences of oral administration of Lactobacillus rhamnosus strain GG in healthy volunteers. J Dairy Res 2003;70:165-73. [Crossref] [PubMed]
- Kolling Y, Salva S, Villena J, et al. Are the immunomodulatory properties of Lactobacillus rhamnosus CRL1505 peptidoglycan common for all Lactobacilli during respiratory infection in malnourished mice? PLoS One 2018;13:e0194034. [Crossref] [PubMed]
- Mahnet S, Kirin S, Kos B, et al. Immunomodulatory effect of Lactobacillus rhamnosus GG from low fat fresh cheese “BioAktiv LGG.” Mljekarstvo 2004;54:5-18.
- Hajishengallis G, Chavakis T. Local and systemic mechanisms linking periodontal disease and inflammatory comorbidities. Nat Rev Immunol 2021;21:426-40. [Crossref] [PubMed]
- Tao D, Zhong T, Pang W, et al. Saccharomyces boulardii improves the behaviour and emotions of spastic cerebral palsy rats through the gut-brain axis pathway. BMC Neurosci 2021;22:76. [Crossref] [PubMed]
- Gholiof M, Adamson-De Luca E, Wessels JM. The female reproductive tract microbiotas, inflammation, and gynecological conditions. Front Reprod Health 2022;4:963752. [Crossref] [PubMed]
- Seely KD, Kotelko CA, Douglas H, et al. The Human Gut Microbiota: A Key Mediator of Osteoporosis and Osteogenesis. Int J Mol Sci 2021;22:9452. [Crossref] [PubMed]
- Rice JE 3rd, Vannucci RC, Brierley JB. The influence of immaturity on hypoxic-ischemic brain damage in the rat. Ann Neurol 1981;9:131-41. [Crossref] [PubMed]
- Vuralli D, Wattiez AS, Russo AF, et al. Behavioral and cognitive animal models in headache research. J Headache Pain 2019;20:11. [Crossref] [PubMed]
- Critten V, Campbell E, Farran E, et al. Visual perception, visual-spatial cognition and mathematics: Associations and predictions in children with cerebral palsy. Res Dev Disabil 2018;80:180-91. [Crossref] [PubMed]
- Bartonek Å, Piccardi L, Guariglia C. Topographical Working Memory in Children with Cerebral Palsy. J Mot Behav 2021;53:200-8. [Crossref] [PubMed]
- Kraeuter AK, Guest PC, Sarnyai Z. The Y-Maze for Assessment of Spatial Working and Reference Memory in Mice. Methods Mol Biol 2019;1916:105-11. [Crossref] [PubMed]
- Deleidi M, Jäggle M, Rubino G. Immune aging, dysmetabolism, and inflammation in neurological diseases. Front Neurosci 2015;9:172. [Crossref] [PubMed]
- Gustafson DR, Karlsson C, Skoog I, et al. Mid-life adiposity factors relate to blood-brain barrier integrity in late life. J Intern Med 2007;262:643-50. [Crossref] [PubMed]
- Zhang JM, An J. Cytokines, inflammation, and pain. Int Anesthesiol Clin 2007;45:27-37. [Crossref] [PubMed]
- PubChem. Superpathway of N-acetylneuraminate degradation (Internet). pubchem.ncbi.nlm.nih.gov. (cited 2023 Sep 5). Available online: https://pubchem.ncbi.nlm.nih.gov/pathway/BioCyc:META_P441-PWY
- PubChem. Superpathway of glycolysis and the Entner-Doudoroff pathway (Internet). pubchem.ncbi.nlm.nih.gov. (cited 2023 Sep 5). Available online: https://pubchem.ncbi.nlm.nih.gov/pathway/BioCyc:META_GLYCOLYSIS-E-D
- PubChem. pyruvate fermentation to acetone (Internet). pubchem.ncbi.nlm.nih.gov. Available online: https://pubchem.ncbi.nlm.nih.gov/pathway/BioCyc:META_PWY-6588
- PubChem. L-glutamate and L-glutamine biosynthesis (Internet). pubchem.ncbi.nlm.nih.gov. (cited 2023 Sep 5). Available online: https://pubchem.ncbi.nlm.nih.gov/pathway/BioCyc:META_PWY-5505
- Tomita S, Nemoto T, Matsuo Y, et al. A NMR-based, non-targeted multistep metabolic profiling revealed L-rhamnitol as a metabolite that characterised apples from different geographic origins. Food Chem 2015;174:163-72. [Crossref] [PubMed]
- Yates N, Gunn AJ, Bennet L, et al. Preventing Brain Injury in the Preterm Infant-Current Controversies and Potential Therapies. Int J Mol Sci 2021;22:1671. [Crossref] [PubMed]
- Huang C, Li X, Wu L, et al. The effect of different dietary structure on gastrointestinal dysfunction in children with cerebral palsy and epilepsy based on gut microbiota. Brain Dev 2021;43:192-9. [Crossref] [PubMed]
- Li Q, Xu T, Shao C, et al. Obstructive sleep apnea is related to alterations in fecal microbiome and impaired intestinal barrier function. Sci Rep 2023;13:778. [Crossref] [PubMed]
- Nie K, Ma K, Luo W, et al. Roseburia intestinalis: A Beneficial Gut Organism From the Discoveries in Genus and Species. Front Cell Infect Microbiol 2021;11:757718. [Crossref] [PubMed]
- Dalile B, Van Oudenhove L, Vervliet B, et al. The role of short-chain fatty acids in microbiota-gut-brain communication. Nat Rev Gastroenterol Hepatol 2019;16:461-78. [Crossref] [PubMed]
- Tamanai-Shacoori Z, Smida I, Bousarghin L, et al. Roseburia spp.: a marker of health? Future Microbiol 2017;12:157-70. [Crossref] [PubMed]
- Dordević D, Jančíková S, Vítězová M, et al. Hydrogen sulfide toxicity in the gut environment: Meta-analysis of sulfate-reducing and lactic acid bacteria in inflammatory processes. J Adv Res 2021;27:55-69. [Crossref] [PubMed]