Enhanced diagnosis of severe bacterial and fungal respiratory infection in children using a rapid syndromic array—case report
Highlight box
Key findings
• Molecular diagnostic arrays can incorporate targets for atypical and rare pathogens as ‘safety netting’ for clinicians. They can also include targets for pathogens that commonly cause bacterial co-infection, which can help clinicians rationalise antimicrobial therapy earlier.
What is known and what is new?
• A microbiological cause of infection is infrequently identified in critically unwell children with a respiratory infection.
• Molecular diagnostic arrays can provide a pathogen-specific diagnosis more rapidly than routine microbiological cultures, and in some instances detect pathogens that would not be identified on routinely performed cultures.
What is the implication, and what should change now?
• Molecular diagnostic arrays are highly sensitive tests, therefore require careful interpretation to differentiate pathogens from commensals and contaminants. When implemented, a multidisciplinary approach should be taken when interpreting results.
Introduction
Lower respiratory tract infection (LRTI) is a leading cause of admissions to paediatric intensive care units (PICUs) (1). Between 2–7% of children hospitalised for community acquired pneumonia (CAP) require mechanical ventilation in high-income countries (2,3). Children requiring prolonged mechanical ventilation for other problems can develop ventilator-associated pneumonia (VAP). Although there are consensus-based definitions of pneumonia (4,5), adherence to these definitions may vary and pathogen-specific diagnoses may be dependent on laboratory methodology and whether microorganism/s detected are considered the underlying cause of infection. National audits in the UK have found that a pathogen is identified in 9–16% of cases of paediatric pneumonia (3). Microbiological cultures are limited by previously administered antimicrobials, particularly in the PICU where broad-spectrum treatment is common (6). Molecular syndromic diagnostic tests are an alternative method for obtaining a pathogen-specific diagnosis in critically ill children. Whilst there are several studies describing use of these tests in paediatric samples, descriptions of real-world application and interpretation of these tests to influence clinical decision making are limited (7-11). Here we describe three cases which demonstrate ways in which molecular diagnostics can impact on prescribing decisions in the PICU.
PICU patients with suspected respiratory infections had samples obtained via endotracheal tube aspiration (ETA) or non-bronchoscopic bronchoalveolar lavage (BAL) (mini-BAL). In this procedure, saline is instilled and re-aspirated using an in-line suction catheter at the level of the carina. Nucleic acid was extracted from the sample and underwent a 52-respiratory pathogen TaqMan array card (TAC) (Table S1). This array was custom designed for use in this institution using a combination of commercially available targets in addition to targets that were sequenced in-house (12). Each target was reported according to cycle threshold (Ct), which represents the number of cycles of polymerase chain reaction (PCR) amplification that occurred before the exponential increase in the fluorescent reporter signal. A Ct result of ≤32 was generally considered significant, given this corresponded with clinically significant growth on microbiological culture during validation (12). Importantly, the result was taken in the context of the patient regardless of Ct value. We present this article in accordance with the CARE reporting checklist (available at https://tp.amegroups.com/article/view/10.21037/tp-23-525/rc).
Case presentation
Consent and procedures
All procedures performed in the study were in accordance with the ethical standards of the institutional and/or national research committee(s) and with the Helsinki Declaration (as revised in 2013). Written informed consent was obtained from all patients’ legal guardians for publication of this case report. A copy of the written consent is available for review by the editorial office of this journal.
Case one: Candida pneumonia in a trauma patient
A 17-year-old male went for an emergency laparotomy in his local hospital after a motorcycle accident in which he sustained a grade V liver laceration, a right-sided pneumothorax, rib, and limb fractures. He was intubated, his abdomen was packed, and post-operatively he was transferred to PICU. Haemostasis was achieved on re-exploration of the abdomen and hepatic and coeliac artery embolisation. During stabilisation, >10 L of blood products were required. He initially received amoxicillin-clavulanic acid, gentamicin, and fluconazole prophylaxis peri-operatively, which was rationalised to piperacillin-tazobactam in PICU. He returned to theatre on day 3 to manage bile leak and underwent endoscopic retrograde cholangiopancreatography (ERCP) with biliary stenting, peritoneal drain insertion and removal of intra-abdominal packs. On day 5 of his admission, his fraction of inspired oxygen (FiO2) needed to be progressively increased from 21% to 80% despite incremental increases in peak end-expiratory pressure (PEEP). He also began to develop fevers. Chest radiograph demonstrated consolidation of the left lower lobe of the lung. Following mini-BAL culture and TAC, treatment gentamicin was added, and piperacillin-tazobactam was continued. TAC results returned on day 6, after 25 hours, suggestive of Candida albicans (C. albicans) VAP (Figure 1A). The microbiology team thought that the TAC result was ‘colonising microbiota’ and not necessarily contributing to current state. Pulmonary embolism was found on computerised tomography pulmonary angiogram (CTPA), and anti-coagulation was commenced. By day 7, the patient required 100% oxygen, and PEEP on conventional mechanical ventilation was set at 10 cmH2O. Antimicrobials were changed to meropenem, gentamicin and amphotericin-B, as part of an escalation plan based on TAC results and clinical information. On day 8, 70 hours after collection, C. albicans was identified on the mini-BAL culture. The same organism was isolated on blood culture on day 11. He received a total of 7 days of gentamicin, 12 days of meropenem and 6 days of amphotericin-B. By day 17, the patient was recovering well and was discharged from the PICU.
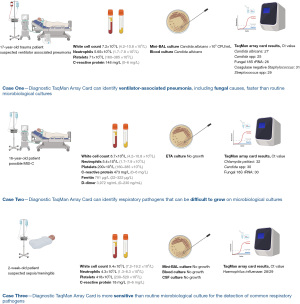
Case two: Chlamydia psittaci (C. psittaci) pneumonia in an undifferentiated adolescent
A 16-year-old female presented to her local hospital with a 3-day history of fever, cough, and increased work of breathing. She required FiO2 70% to maintain SaO2 >90% on high-flow nasal cannulae running at 60 L/min. Her initial inflammatory markers were significantly elevated (Figure 1B). Severe acute respiratory syndrome coronavirus 2 (SARS-CoV-2) combined nasopharyngeal-oropharyngeal swab quantitative PCR (qPCR) was negative. On chest radiograph, she had bilateral basal consolidation and parapneumonic effusion. Given worsening respiratory distress, she was intubated and transferred to PICU. The leading differential diagnosis was multisystem inflammatory syndrome in children (MIS-C), a latent response to coronavirus disease 2019 (COVID-19). MIS-C was suspected given the history of fever, inflammatory presentation of the patient, and a high incidence of this problem in UK PICUs at the time (13).
TAC, undertaken on a mini-BAL sample obtained at the time of admission to PICU, identified C. psittaci, which was reported back the following day. On further questioning, the family said they had purchased two pet cockatiels, one of which had recently died. The patient initially received meropenem and clindamycin, later rationalised to a 10-day course of doxycycline. She required 3 days of mechanical ventilation and was discharged to the ward. The C. psittaci result was confirmed on C. psittaci/abortus serology undertaken in the national reference laboratory, with results available after 10 days.
Case three: Haemophilus influenzae (H. influenzae) pneumonia in a neonate with suspected sepsis and meningitis
A 2-week-old male, born at 35+5 weeks gestation, was taken to his local hospital with increased work of breathing and apnoeas. He had received 5 days of antimicrobial therapy after birth for suspected sepsis with no organism identified. In the present admission, he had a short history of cough and nasal congestion. Despite treatment with high-flow nasal cannulae, intubation was required due to the persistence of apnoeas. He then needed 40 mL/kg in fluid boluses for hypotension and tachycardia. Ceftriaxone, amoxicillin and acyclovir were given to treat possible sepsis and meningitis. He was transferred to PICU overnight. The patient was producing moderate, thick yellow secretions and there were scattered crackles on chest auscultation. Chest radiograph demonstrated diffuse interstitial change. Mini-BAL was undertaken the following morning given suspicion of a primary respiratory source of infection. H. influenzae was reported on TAC 5 hours after sample collection (Figure 1C). Amoxicillin and acyclovir were accordingly ceased. Ceftriaxone was rationalised to a 10-day course of amoxicillin-clavulanate, and he was extubated and discharged to the ward on day 3 of PICU admission. There were no organisms reported on microbiological cultures when results became available later.
Discussion
These clinical cases demonstrate that TAC can diagnose bacterial and fungal infections in critically ill children faster than routine investigations, and in some instances, identify pathogens that would not be identified using first-line tests. Diagnostic syndromic arrays for pneumonia have been widely commercially available since 2018, but their potential role in children has not been described broadly (14,15).
In our first case study, C. albicans pneumonia was identified earlier by TAC than routine microbiological cultures. Candida causes between 2.4–11.9% of paediatric VAP (16). There are no targets for Candida on commercial pneumonia syndromic arrays. Whilst C. albicans can be a lung commensal, pulmonary candidiasis can occur in severely unwell and immunocompromised patients—a group that frequently requires PICU admission. This pneumonia likely occurred secondary to disseminated candidiasis. The presence of candidaemia can increase mortality by 10.0% (95% confidence interval: 6.2–13.8%) in children; hence its prompt identification is essential (17). If found on a respiratory sample alone, clinicians must decide whether it requires treatment (18). In this case, whilst anti-fungal treatment was not given immediately, identification of Candida using TAC allowed treatment to be escalated promptly when the patient demonstrated signs of deterioration.
In the second case study, an unexpected diagnosis of C. psittaci was made due to the detection on the TAC, which would have been unlikely to have been identified with routine tests. Psittacosis occurs due to contact with aerosols generated from the urine or faeces of birds, particularly those belonging to the Psittaciformes—parrots, parakeets, budgerigars and cockatiels (19,20). It is an intracellular, Gram-negative infection that is rare in children; however, is expected to be identified more frequently due to the increasing availability of molecular diagnostic tests (19,20). In this case, TAC significantly impacted treatment as the patient initially had poor Gram-negative antimicrobial cover. A benefit of TAC is that it contains a wide range of pathogens that may help detect unusual respiratory pathogens, potentially offering a diagnosis in situations of uncertainty.
In the last case, H. influenzae pneumonia was identified as the primary cause of infection, resulting in neonatal apnoeas. In this case, early identification of the organism with TAC allowed antimicrobial cover for presumed central nervous system infection to be rationalised sooner than if decision making was based on microbiological culture. The prevalence of invasive paediatric Haemophilus has reduced significantly since the introduction of the H. influenzae type B (Hib) vaccine, which is administered in repeated doses from eight weeks of life.
The three cases highlight the role and potential limitations of syndromic diagnostic arrays in children. The TAC identified the cause of pneumonia faster than routine cultures and provided a cause where it may not have been otherwise identified. Respiratory cultures in the PICU are typically obtained after children have received broad-spectrum antimicrobial therapy, when initial signs of bacterial LRTI, or deterioration is identified. This reduces the viable number of organisms in specimens, reducing the yield of microbiological culture. Molecular diagnostic tests are advantageous in that they do not require a live organism, but instead detect nucleic acid to assist clinicians in making a pathogen-specific diagnosis. Molecular diagnostics tests are capable of identifying respiratory pathogens within 1–5 hours (21-24), offering the potential for clinicians to make earlier antimicrobial decisions.
As is the case for microbiological cultures, it is possible to identify organisms that may be commensals/contaminants with rapid molecular diagnostic arrays. This was initially suspected in case one, which is why the clinical oversight by the multidisciplinary team is essential. Without this input there is a risk of unnecessary escalation of antimicrobial therapy should low consequence microorganisms be detected. Diagnostic arrays, such as TAC, are limited to detection of pathogens pre-specified by design. Therefore, it is important for clinicians to be aware that a negative test result does not indicate absence of an off-panel pathogen. This is critical to consider where tests are used in international settings, where there may be different patterns of epidemiology. Finally, there is still progress to be made in implementing molecular diagnostic tests into clinical practice. Institutions must have the laboratory infrastructure and personnel, and familiarity of clinicians in interpreting the tests for their routine use to be effective (25).
In comparison to commercially available molecular diagnostic arrays, TAC has a longer turnaround time given the need for laboratory staff to perform extraction of nucleic acid manually. However, the test is capable of a broader range of pathogens that other tests and is customisable. Currently, use of the respiratory pathogen TAC is limited to this centre, but in future may become available if validated through a larger scale study. We demonstrate through these cases how this syndromic diagnostic test could influence the management of PICU patients.
Conclusions
Respiratory infection is frequently encountered in the PICU, but a bacterial or fungal cause is often not identified due to the limitations of microbiological cultures. Use of a custom TAC can deliver rapid and sensitive results in mechanically ventilated children with suspected LRTI. If demonstrated to be reliable and cost-effective in a dedicated paediatric randomised control trial, TAC could become a routine adjunct to microbiological cultures in the PICU.
Acknowledgments
We wish to thank the families, children and young people who kindly agreed to be involved in this case report.
Funding: This project was funded by
Footnote
Reporting Checklist: The authors have completed the CARE reporting checklist. Available at https://tp.amegroups.com/article/view/10.21037/tp-23-525/rc
Peer Review File: Available at https://tp.amegroups.com/article/view/10.21037/tp-23-525/prf
Conflicts of Interest: All authors have completed the ICMJE uniform disclosure form (available at https://tp.amegroups.com/article/view/10.21037/tp-23-525/coif). J.A.C. received PhD personal funding through the Gates Cambridge Trust and funding for the consumables for the research were provided by the Addenbrooke’s Charitable Trust, Cambridge University Hospitals. T.G. receives a salary from the NIHR Cambridge Biomedical Research Campus and participates in monitoring the PROMISE study. A.C.M. receives support from the Medical Research Council Clinician Scientist Fellowship and Wellcome Trust Clinical Research Career Development Fellowship, and has received speaker fees from BioMérieux, Boston Scientific and Fisher and Paykel. M.D.C. is the inventor of a patent held by the Secretary of State for Health (UK government) EP2788503, which covers some of the genetic sequences used in this study. V.N. is a founder, director, and shareholder in Cambridge Infection Diagnostics (CID), which is a commercial company aimed at developing molecular diagnostics in infection and antimicrobial and AMR stewardship. A.C.M. and S.B. are advisory board members of the CID. S.B. has received funding from Addenbrooke’s Charitable Trust, Cambridge University Hospitals, Wellcome Trust and Action Medical Research. N.P. has received funding from Action Medical Research, the Addenbrooke’s Charitable Trust, Cambridge University Hospitals and the Cambridge NIHR Biomedical Research Centre, and has received speaker fees from BioMérieux. The other authors have no conflicts of interest to declare.
Ethical Statement: The authors are accountable for all aspects of the work in ensuring that questions related to the accuracy or integrity of any part of the work are appropriately investigated and resolved. All procedures performed in the study were in accordance with the ethical standards of the institutional and/or national research committee(s) and with the Helsinki Declaration (as revised in 2013). Written informed consent was obtained from the patients’ legal guardians for publication of this case report. A copy of the written consent is available for review by the editorial office of this journal.
Open Access Statement: This is an Open Access article distributed in accordance with the Creative Commons Attribution-NonCommercial-NoDerivs 4.0 International License (CC BY-NC-ND 4.0), which permits the non-commercial replication and distribution of the article with the strict proviso that no changes or edits are made and the original work is properly cited (including links to both the formal publication through the relevant DOI and the license). See: https://creativecommons.org/licenses/by-nc-nd/4.0/.
References
- Paediatric Intensive Care Audit Network. Paediatric Intensive Care Audit Network Annual Report (2021) [Internet]. 2022 Apr [cited 2023 Feb 13]. Available online: https://www.picanet.org.uk/wp-content/uploads/sites/25/2022/04/PICANet-2021-Annual-Report_v1.1-22Apr2022.pdf
- Jain S, Williams DJ, Arnold SR, et al. Community-acquired pneumonia requiring hospitalization among U.S. children. N Engl J Med 2015;372:835-45. [Crossref] [PubMed]
- Legg J, Rampton C. Paediatric Pneumonia Audit. National Audit Period: 1 November 2016 - 31 January 2017. London; 2018 Jan.
- National Health Safety Network. Pneumonia (Ventilator-associated [VAP] and non-ventilator- associated Pneumonia [PNEU]) Event [Internet]. Atlanta, USA; 2023 [cited 2023 Feb 13]. Available online: https://www.cdc.gov/nhsn/pdfs/pscmanual/6pscvapcurrent.pdf
- Harris M, Clark J, Coote N, et al. British Thoracic Society guidelines for the management of community acquired pneumonia in children: update 2011. Thorax 2011;66:ii1-23. [Crossref] [PubMed]
- Clark J, White D, Daubney E, et al. Low diagnostic yield and time to diagnostic confirmation results in prolonged use of antimicrobials in critically ill children. Wellcome Open Res 2021;6:119. [Crossref] [PubMed]
- Fireizen Y, Babbitt CJ, Adams S, Morphew T, Ferro ET, Randhawa I. The Impact of Pneumonia PCR Panel Testing in the PICU: A Quality Improvement Study. J Pediatr Intensive Care 2022; [Crossref]
- Yen TY, Chen JF, Lu CY, et al. Application of nested multiplex polymerase chain reaction respiratory and pneumonia panels in children with severe community-acquired pneumonia. J Med Virol 2023;95:e28334. [Crossref] [PubMed]
- Papan C, Meyer-Buehn M, Laniado G, et al. Assessment of the multiplex PCR-based assay Unyvero pneumonia application for detection of bacterial pathogens and antibiotic resistance genes in children and neonates. Infection 2018;46:189-96. [Crossref] [PubMed]
- Moleleki M, du Plessis M, Ndlangisa K, et al. Pathogens detected using a syndromic molecular diagnostic platform in patients hospitalized with severe respiratory illness in South Africa in 2017. Int J Infect Dis 2022;122:389-97. [Crossref] [PubMed]
- Pandolfo AM, Horne R, Jani Y, et al. Intensivists' beliefs about rapid multiplex molecular diagnostic testing and its potential role in improving prescribing decisions and antimicrobial stewardship: a qualitative study. Antimicrob Resist Infect Control 2021;10:95. [Crossref] [PubMed]
- Navapurkar V, Bartholdson Scott J, Maes M, et al. Development and implementation of a customised rapid syndromic diagnostic test for severe pneumonia. Wellcome Open Res 2022;6:256. [Crossref] [PubMed]
- Whittaker E, Bamford A, Kenny J, et al. Clinical Characteristics of 58 Children With a Pediatric Inflammatory Multisystem Syndrome Temporally Associated With SARS-CoV-2. JAMA 2020;324:259-69. [Crossref] [PubMed]
- Darie AM, Khanna N, Jahn K, et al. Fast multiplex bacterial PCR of bronchoalveolar lavage for antibiotic stewardship in hospitalised patients with pneumonia at risk of Gram-negative bacterial infection (Flagship II): a multicentre, randomised controlled trial. Lancet Respir Med 2022;10:877-87. [Crossref] [PubMed]
- Poole S, Tanner AR, Naidu VV, et al. Molecular point-of-care testing for lower respiratory tract pathogens improves safe antibiotic de-escalation in patients with pneumonia in the ICU: Results of a randomised controlled trial. J Infect 2022;85:625-33. [Crossref] [PubMed]
- Venkatachalam V, Hendley JO, Willson DF. The diagnostic dilemma of ventilator-associated pneumonia in critically ill children. Pediatr Crit Care Med 2011;12:286-96. [Crossref] [PubMed]
- Zaoutis TE, Argon J, Chu J, et al. The epidemiology and attributable outcomes of candidemia in adults and children hospitalized in the United States: a propensity analysis. Clin Infect Dis 2005;41:1232-9. [Crossref] [PubMed]
- Pendleton KM, Huffnagle GB, Dickson RP. The significance of Candida in the human respiratory tract: our evolving understanding. Pathog Dis 2017;75:ftx029. [Crossref] [PubMed]
- Public Health England. Guidance: Psittacosis. 2017. Available online: https://www.gov.uk/guidance/psittacosis
- Qu J, Zhang J, Chen Y, et al. Aetiology of severe community acquired pneumonia in adults identified by combined detection methods: a multi-centre prospective study in China. Emerg Microbes Infect 2022;11:556-66. [Crossref] [PubMed]
- Gadsby NJ, Russell CD, McHugh MP, et al. Comprehensive Molecular Testing for Respiratory Pathogens in Community-Acquired Pneumonia. Clin Infect Dis 2016;62:817-23. [Crossref] [PubMed]
- Personne Y, Ozongwu C, Platt G, et al. 'Sample-in, answer-out'? Evaluation and comprehensive analysis of the Unyvero P50 pneumonia assay. Diagn Microbiol Infect Dis 2016;86:5-10. [Crossref] [PubMed]
- Li J, Tao Y, Tang M, et al. Rapid detection of respiratory organisms with the FilmArray respiratory panel in a large children's hospital in China. BMC Infect Dis 2018;18:510. [Crossref] [PubMed]
- Leber AL, Everhart K, Daly JA, et al. Multicenter Evaluation of BioFire FilmArray Respiratory Panel 2 for Detection of Viruses and Bacteria in Nasopharyngeal Swab Samples. J Clin Microbiol 2018;56:e01945-17. [Crossref] [PubMed]
- Clark JA, Conway Morris A, Kanaris C, et al. A qualitative investigation of paediatric intensive care staff attitudes towards the diagnosis of lower respiratory tract infection in the molecular diagnostics era. Intensive Care Med Paediatr Neonatal 2023;1:10. [Crossref] [PubMed]