Quantitative computed tomography assessment of bone mineral density in adolescent idiopathic scoliosis: correlations with Cobb angle, vertebral rotation, and Risser sign
Highlight box
Key findings
• In patients with adolescent idiopathic scoliosis (AIS), there is a negative correlation between bone mineral density (BMD) and the severity of the deformity.
• Cobb angle is a risk factor for the presence of low BMD in AIS patients.
What is known and what is new?
• The influence of spinal curvature on BMD has been firmly established.
• This study highlights growth and vertebral rotation’s role, with a novel focus on quantitative computed tomography’s (QCT) utility in patients with AIS.
What is the implication, and what should change now?
• The study proposed a predictive model for identifying low BMD in AIS patients in the absence of QCT.
• The clinical value of QCT for personalized treatment plans is underscored.
Introduction
Scoliosis, particularly adolescent idiopathic scoliosis (AIS), is the most prevalent spinal deformity during adolescence, affecting 0.47% to 5.20% of youth globally (1). It is characterized by a lateral curvature of the spine, typically assessed by the Cobb angle, which serves as a pivotal metric for evaluating the severity of spinal deformity (2). Additionally, vertebral rotation is another crucial parameter providing a three-dimensional perspective on the extent of this deformity (3). The impact of AIS on spinal and thoracic growth can lead to serious complications, including respiratory and cardiac issues in severe cases (4,5). Therefore, a comprehensive understanding of the complex dynamics of spinal growth, along with key maturation markers such as the Risser sign and bone age assessment, is imperative for timely and effective intervention to prevent progression into adulthood in AIS (6).
Bone mineral density (BMD) is an established determinant of bone strength and fracture susceptibility (7). Previous research conducted by Ramos et al. (8). indicated that patients with AIS exhibit lower BMD values compared to healthy individuals, potentially increasing their fragility and fracture risk over time, and reduced quality of life in daily activities (9). In addition, a study by Wu et al. showed an inverse correlation between preoperative BMD and intraoperative blood loss in patients with AIS, which increases the susceptibility to postoperative non-neurological complications such as respiratory problems, incisional infections, and hematomas (10). Additionally, the Risser sign, a scale assessing the ossification of the iliac apophysis and thus indicating the stage of skeletal maturity (11), could potentially correlate with BMD changes, given the close relationship between skeletal maturity and the development of bone density. Therefore, it is of potential interest to conduct bone density and Risser sign measurements in patients with scoliosis. Moreover, In the intricate interplay between bone metabolism and skeletal deformities, calcium (Ca) and phosphate (P) levels emerge as critical biochemical markers. Previous studies have elucidated the perturbations in Ca and P homeostasis associated with bone loss, a condition that predisposes individuals to osteoporosis and fractures, common complications in patients with AIS (12). To explore their associations with changes in BMD among AIS patients, we have incorporated these blood count parameters into our study.
Quantitative computed tomography (QCT) is an accurate measurement offering a sophisticated and precise method for BMD assessment, with cross-sectional imaging providing a detailed volumetric analysis (13). This technology is superior to dual-energy X-ray absorptiometry (DXA) in differentiating between trabecular and cortical bone, offering a three-dimensional perspective that is less likely to be confounded by extraneous factors such as spinal degeneration or aortic calcifications (14). A previous study conducted by Almomen et al. demonstrated that in women diagnosed with AIS, those with more pronounced spinal curvature, as indicated by higher Cobb angle measurements, are more likely to have lower BMD values, as determined through DXA assessments (15). However, the availability and cost of QCT may limit its use in certain clinical institutions. As a result, conventional CT and X-ray remain the primary methods for evaluating AIS in some medical Institutions. Therefore, our study aims to establish correlations between BMD, Cobb Angle, Vertebral Rotation, Risser Sign, and other clinical outcomes to determine if these parameters can be used to predict bone mass loss in AIS patients. This would enable clinics to develop timely strategies for managing patients with AIS and mitigate the progression of osteoporosis in situations where QCT is unavailable. We present this article in accordance with the STARD reporting checklist (available at https://tp.amegroups.com/article/view/10.21037/tp-24-74/rc).
Methods
Study population
This cross-sectional study enrolled patients with scoliosis from The Third People’s Hospital of Chengdu, Sichuan, China, between 2021 and 2023. These patients were prospectively enrolled based on the following considerations: (I) individuals were required to be between the ages of 10 and 18 years and diagnosed with AIS; (II) both male and female patients were actively recruited for the study to ensure a gender-balanced sample; (III) the patients did not undergo any surgical or brace treatment; and (IV) patients’ guardians were required to sign an informed consent form before enrolling in the study.
The exclusion criteria were the following: (I) patients with secondary scoliosis resulting from neurological or muscular disorders, congenital abnormalities, fractures, infections, metabolic diseases, or other non-idiopathic causes; (II) patients with congenital scoliosis caused by abnormal spinal development during the fetal period (e.g., spina bifida and congenital vertebral anomalies); (III) individuals with severe osteoporosis or other bone metabolic diseases and those who have undergone spinal or orthopedic surgeries; and (IV) non-Asian patients (to eliminate any potential influence of race on the results) (Figure 1). A total of 150 patients diagnosed with AIS were included in this study, while 21 patients with incomplete laboratory examination records, incomplete CT images, or other exclusion criteria were excluded. Ultimately, a cohort of 129 patients were analyzed. The study was conducted in accordance with the Declaration of Helsinki (as revised in 2013). A protocol was prepared before the study without registration, and the study was approved by the Medical Ethics Committee of The Third People’s Hospital of Chengdu (No. 2021-S-13) and informed consent was taken from all the patients’ guardians.
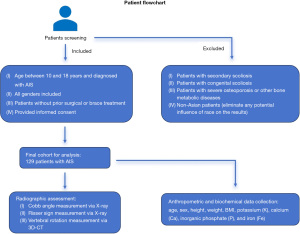
Anthropometric and biochemical data collection
Age at the time of consultation and sex were recorded. Height (in cm) and weight (in kg) were measured using a standardized procedure, while body mass index (BMI) was calculated using the following formula: BMI = weight/height2. Blood samples were collected from each participant following an overnight fast. The samples were processed and analyzed for the following: potassium (K), calcium (Ca), inorganic phosphate (P), and iron (Fe).
Radiographic assessment
The patients underwent posteroanterior spinal radiographs using a DigitalDiagnost DR-TH system (Philips), and the Cobb angle was measured to assess the severity of the spinal curve and to distinguish between thoracic and lumbar curve type based on the location of the apex. The radiographic assessments were conducted by J.G. and Z.Z., two highly experienced radiologists with over 15 years of experience. Any conflicting opinions among the reviewers were resolved through discussion.
The Risser sign was documented from the radiographs for evaluating skeletal maturation and subsequently classified into six groups (Risser 0–5) based on the ossification and fusion of the iliac crest apophysis, with the higher numbers denoting advancement toward skeletal maturity. Stage 0 refers to an X-ray image where the absence of an ossification center is observed in the apophysis, while stage 5 indicates complete ossification and fusion of the iliac apophysis (16). The CT scans were performed using a 64-multidetector CT system (SOMATOM Definition AS, Siemens Healthineers, Germany). The assessment of vertebral rotation was conducted using 3D CT images, following the established methodology described by Ho et al. (3). In this approach, the pedicles were identified on an axial CT slice of the vertebra. Subsequently, two lines were drawn: one passing through the center of the vertebral body and another bisecting the pedicles. The angle between these two lines represented the rotation angle (Figure 2). QCT Pro (Mindways Software) was used to measure BMD in the lumbar spine of all study patients. The region of interest (ROI) was meticulously drawn on the trabecular bone of the vertebral body, with cortical bone and the basivertebral venous plexus being avoided to obtain a representative measure of the true bone density (Figure 3, Table 1). According to the American College of Radiology Practice Parameter for the performance of Musculoskeletal Quantitative Computed Tomography (revised 2018 version), A BMD value exceeding 120 g/cm2 is defined as normal BMD, while a range of 80–120 g/cm2 indicates osteopenia, and values below 80 g/cm2 indicate osteoporosis (17). In this study, we used a BMD threshold of 120 g/cm2 since values below this threshold indicate low BMD levels, which may lead to clinically significant complications.
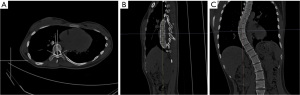
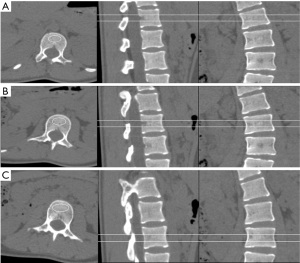
Table 1
ROI | BMD (g/cm2) | Z value |
---|---|---|
L1 | 220.4 | 1.8 |
L2 | 219.1 | 1.8 |
L3 | 233.6 | 2.3 |
Average | 224.4 | 1.94 |
The Z value is the difference between the patient’s BMD and the average BMD for healthy people of the same age, ethnicity, and sex. BMD, bone mineral density; ROI, region of interest.
Statistical analysis
Statistical analysis was conducted with SPSS 26 (IBM Corp). Continuous variables are presented as the mean ± standard deviation (SD), while categorical variables are presented as percentages. The normality of distribution for continuous variables was assessed using the Shapiro-Wilk test. Pearson correlation coefficient was calculated to determine the relationship between the normally distributed continuous variables of BMD. Spearman rank correlation was performed for continuous variables that were nonnormally distributed. An independent samples t-test was conducted to investigate the statistically significant difference in BMD between males and females. One-way analysis of variance (ANOVA) tests were used to compare BMD values between thoracic and lumbar curve types. The Kruskal-Wallis test was conducted across different Risser sign groups to assess the statistical significance of variations in BMD.
Multivariate logistic regression was employed to assess the independent predictors of low BMD (BMD ≤120 g/cm2) (17). The statistically significant predictors identified through univariate logistic regression analysis were further examined in a binary logistic regression model. Receiver operating characteristic (ROC) curve analysis was employed to assess the accuracy of predicting low BMD (BMD ≤120 g/cm2) in patients with AIS.
Results
Clinical characteristics
For the 129 patients with AIS included in this study, the demographic data, including age, sex, BMI, BMD, Cobb angle, vertebral rotation angle, curve type, Risser sign, and biochemical information, are shown in Table 2. The mean age of the patients was 14.98±1.85 years, the mean height was 152.50±12.45 cm, and the mean BMI was 18.40±2.61 kg/m2. The Shapiro-Wilk test for normality revealed that BMD and K exhibited a normal distribution (P>0.05 both); conversely, age, Cobb angle, rotation angle, Ca, P, Fe, height, and BMI did not follow a normal distribution (P<0.01 all).
Table 2
Characteristics | Values (n=129) | P value |
---|---|---|
Age (years) | 14.98±1.85 [11–18] | <0.001 |
Sex | ||
Female | 85 (65.9) | |
Male | 44 (34.1) | |
Height (cm) | 152.50±12.45 [115.00–177.00] | 0.003 |
BMI (kg/m2) | 18.40±2.61 [12.03–27.55] | 0.001 |
BMD (g/cm2) | 138.32±44.47 [21.9–252.3] | 0.29 |
Cobb angle (°) | 68.52±29.74 [12.3–171.0] | <0.001 |
Vertebral rotation angle (°) |
22.47±15.29 [1.0–79.8] | <0.001 |
Curve type | ||
Thoracic | 102 (79.1) | |
Lumbar | 27 (20.9) | |
Risser sign | ||
0 | 7 (5.4) | |
1 | 10 (7.8) | |
2 | 10 (7.8) | |
3 | 22 (17.0) | |
4 | 66 (51.1) | |
5 | 14 (10.9) | |
Potassium (mEq/L) | 4.14±0.34 [3.38–5.33] | 0.09 |
Calcium (mmol/L) | 2.28±0.12 [1.92–2.52] | 0.001 |
Inorganic phosphate (mmol/L) | 1.54±0.25 [0.67–2.10] | 0.001 |
Iron (µmol/L) | 15.66±8.22 [2.43–36.58] | <0.001 |
Values are presented as mean ± standard deviation [range] or numbers (comparative percentages). The P value indicates the Shapiro-Wilk test result for the assessment of the normality of the distribution of continuous variables. BMD, bone mineral density; BMI, body mass index.
Correlation analyses
The correlation analyses between BMD and other continuous variables in patients diagnosed with AIS are presented in Table 3. The results revealed a significant negative correlation between BMD and Cobb angle (coefficient =−0.663; P<0.001), suggesting that an increase in Cobb angle was associated with a decrease in BMD. Beside, a significant negative correlation (coefficient =−0.442; P<0.001) was observed between BMD and vertebral rotation angle. Additionally, a significant positive correlation was observed between BMD and height (coefficient =0.355; P<0.001) and between BMD and BMI (coefficient =0.199; P=0.02), indicating that higher values of height and BMI are related to higher BMD measurements. The scatter plots of the correlation of BMD versus Cobb angle and rotation angle in patients with AIS are shown in Figure 4, which shows an inverse relationship between BMD and the Cobb angle: as the Cobb angle increases, a corresponding decrease in BMD is observed, which is consistent with our findings of a negative correlation. Furthermore, a similar pattern was observed in terms of BMD versus vertebral rotation angle, with a decrease in BMD as the rotation angle increased. Furthermore, no significant correlations were observed between BMD age, and levels of K, Ca, P, and Fe (P>0.05 all).
Table 3
Variables | Coefficient | P value |
---|---|---|
Age (years) | 0.009 | 0.92 |
Height (cm) | 0.355 | <0.001 |
BMI (kg/m2) | 0.199 | 0.02 |
Cobb angle (°) | −0.663 | <0.001 |
Vertebral rotation angle (°) | −0.442 | <0.001 |
Potassium (mEq/L) | −0.084 | 0.34 |
Calcium (mmol/L) | −0.059 | 0.51 |
Inorganic phosphate (mmol/L) | −0.018 | 0.84 |
Iron (µmol/L) | 0.061 | 0.49 |
P values indicate statistical significance for the correlations, with Pearson’s test used for normally distributed variables (BMD and potassium) and Spearman’s rank correlation for non-normally distributed variables (age, Cobb angle, vertebral rotation angle, calcium, inorganic phosphate, iron, height, and BMI). A P value less than 0.05 is considered statistically significant. BMI, body mass index; BMD, bone mineral density.
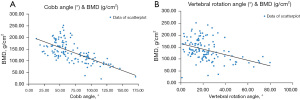
The correlation analysis presented in Table 4 illustrates the correlation analysis of BMD with categorical variables including sex, curve type, and Risser sign in patients with AIS. The results of the independent samples t-test indicated that the mean BMD values were higher in female patients (143.78±45.46 g/cm2) compared to their male (127.76±40.95 g/cm2) counterparts; however, this difference did not achieve statistical significance (P=0.052). Similarly, the one-way ANOVA test reveals no significant variance in BMD among different curve types (P=0.13). Conversely, the Kruskal-Wallis test revealed a significant variation in BMD across different Risser sign categories (P=0.002). Notably, the mean BMD generally increased with higher Risser sign values although a slight decrease was observed at Risser sign 5 in comparison to Risser sign 4. Figure 5 presents two box plots that visualize the distribution of BMD by sex and Risser sign levels. The box plot representing BMD segregated by sex indicates that females exhibited a slightly higher median BMD than did males, along with greater variability, as denoted by the larger interquartile range. The second box plot illustrates the BMD distribution across different Risser sign levels, demonstrating a general trend of increasing median BMD with higher Risser sign values, except for a slight drop at Risser sign 5. This trend is represented by the median line within each box. Furthermore, there was a wider range of BMD values observed in the groups with Risser sign levels of 0 and 3, indicating greater variability within these groups.
Table 4
Parameters | Number of patients |
BMD (g/cm2), mean ± SD |
P value |
---|---|---|---|
Sex | 0.052 | ||
Female | 85 | 143.78±45.46 | |
Male | 44 | 127.76±40.95 | |
Curve type | 0.13 | ||
Thoracic | 102 | 135.26±41.12 | |
Lumbar | 27 | 149.87±54.71 | |
Risser sign | 0.002 | ||
0 | 7 | 85.14±30.33 | |
1 | 10 | 108.43±28.69 | |
2 | 10 | 128.19±41.32 | |
3 | 22 | 140.59±51.25 | |
4 | 66 | 148.42±43.02 | |
5 | 14 | 142.30±31.09 |
The independent samples t-test was used to compare the mean BMD between sexes, one-way analysis of variance was employed for curve types, and the Kruskal-Wallis test was used for different Risser sign categories. BMD, bone mineral density; SD, standard deviation.
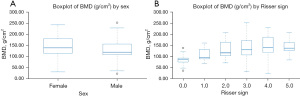
Assessment of risk factors for low BMD in patients with AIS
The results of the univariate analysis, presented in Table 5, revealed several statistically significant associations between AIS patients’ characteristics and low BMD (BMD ≤120 g/cm2). The AIS patients with low BMD exhibited a significantly higher mean Cobb angle of 94.37±27.07 degrees, in comparison to the normal bone mass group with a mean Cobb angle of 52.68±17.86 degrees (P<0.001). Similarly, vertebral rotation angle was significantly greater in the low BMD group (32.00±18.52 degrees) than compared group (16.64±8.92 degrees) (P<0.001). In contrast, Risser sign, which indicates the stage of pelvic bone ossification, showed a significant inverse relationship with low BMD, and higher Risser sign values were less common in the low BMD group (P=0.007). Height was also significantly associated with BMD, with patients with a low BMD were, on average (147.71±13.72 cm), tending to be shorter than those with a higher BMD (155.44±10.66 cm) (P=0.001). However, no significant differences were observed in age or BMI between the two groups (P>0.05 both). Among categorical variables, sex showed a significant distributional difference; specifically, the male group had a greater likelihood of having low BMD than did the female group (P=0.04). Biochemical indices such as K, Ca, P, and Fe did not show significant differences between the low BMD and normal bone mass groups (P>0.05 all). Similarly, the categories for curve type did not exhibit a statistically significant association with BMD level (P=0.58).
Table 5
Variables | Low BMD (BMD ≤120 g/cm2) (n=49) | Normal bone mass (BMD >120 g/cm2) (n=80) | P value |
---|---|---|---|
Age (years) | 14.94±1.88 | 15.00±1.85 | 0.86 |
Height (cm) | 147.71±13.72 | 155.44±10.66 | 0.001 |
Sex | |||
Female | 27 (55.1) | 58 (72.5) | 0.04 |
Male | 22 (44.9) | 22 (27.5) | |
BMI (kg/m2) | 18.14±3.02 | 18.56±2.33 | 0.37 |
Cobb angle (°) | 94.37±27.07 | 52.68±17.86 | <0.001 |
Rotation angle (°) | 32.00±18.52 | 16.64±8.92 | <0.001 |
Potassium (mEq/L) | 4.16±0.34 | 4.12±0.34 | 0.49 |
Calcium (mmol/L) | 2.28±0.13 | 2.28±0.11 | 0.85 |
Inorganic phosphate (mmol/L) | 1.52±0.25 | 1.55±0.25 | 0.54 |
Iron (µmol/L) | 14.77±7.95 | 16.21±8.39 | 0.34 |
Curve type | 0.58 | ||
Thoracic | 40 (81.63) | 62 (77.5) | |
Lumbar | 9 (18.37) | 18 (22.5) | |
Risser sign | 0.007 | ||
0 | 6 (12.24) | 1 (1.25) | |
1 | 6 (12.24) | 4 (5.00) | |
2 | 6 (12.24) | 4 (5.00) | |
3 | 9 (18.37) | 13 (16.25) | |
4 | 20 (40.82) | 46 (57.50) | |
5 | 20 (40.82) | 12 (15.00) |
The continuous variables are presented as the mean ± standard deviation, and categorical variables are were presented as counts and percentages. For continuous variables, the P values were obtained using independent samples t-tests or Mann-Whitney tests if the normality assumptions were unmet. For categorical variables, chi-squared tests were used to compare the proportions between groups. BMD, bone mineral density; BMI, body mass index.
Based on the results of binary logistic regression analysis (Table 6), a statistically significant positive association was observed between the Cobb angle and the risk of low BMD, with a regression coefficient of 0.072 (P<0.001). The odds ratio (OR) of 1.075 suggests that for each degree increase in Cobb angle, there is a corresponding 7.5% higher likelihood of developing low BMD. Conversely, height, sex, vertebral rotation Angle, and Risser sign had regression coefficients of 0.016, −0.023, 0.018, and −0.302 respectively; however, none showed significant differences (P>0.05 all). The combined model equation can be derived from the data presented in Table 6 as follows: ln(p/1−p) =−7.414 + 0.016× height (cm) − 0.023× sex + 0.072× Cobb angle (°) + 0.0018× vertebral rotation angle (°) − 0.302× Risser sign (where p represents probability when low BMD equals one while l−p represents probability when it equals zero. For sex, female is labeled as 1 and male is labeled as 2).
Table 6
Variables | Coefficient | Standard error | OR (95% CI) | P value |
---|---|---|---|---|
Height (cm) | 0.016 | 0.025 | 1.016 (0.968–1.067) | 0.52 |
Sex | −0.023 | 0.563 | 0.978 (0.324–2.949) | 0.97 |
Cobb angle (°) | 0.072 | 0.016 | 1.075 (1.041–1.109) | <0.001 |
Vertebral rotation angle (°) | 0.018 | 0.029 | 1.019 (0.963–1.078) | 0.52 |
Risser sign | −0.302 | 0.241 | 0.739 (0.461–1.185) | 0.21 |
Constant | −7.414 | 4.046 | 0.001 (0.000–1.675) | 0.07 |
McFadden R2: 0.451. The OR reflects the odds change for low BMD per unit increase in continuous predictors or across categorical predictor groups. P values less than 0.05 indicate statistical significance. OR, odds ratio; CI, confidence interval; BMD, bone mineral density.
The predictive ability of height, sex, Cobb angle, vertebral rotation angle, Risser sign, and the combined model for low BMD was evaluated by calculating the area under the curve (AUC) for each factor (Figure 6) and comparative sensitivity and specificity are presented in Table 7. The results demonstrated that the Cobb angle exhibited a high diagnostic value for low BMD with an AUC value of 0.899. The corresponding optimal threshold was determined as 69.00 (with a sensitivity of 0.837, specificity of 0.863, and Youden index of 0.699). Similarly, vertebral rotation angle also displayed a relatively high diagnostic value for low BMD with an AUC value of 0.784 and an optimal threshold of 18.10 (sensitivity: 0.837; specificity: 0.662; Youden index 0.499). Conversely, sex showed a lower diagnostic value for low BMD with an AUC value of only 0.587. Additionally, height individually demonstrated negligible diagnostic utility for low BMD, with an AUC value of merely 0.319. Regarding the combined model, it yielded the highest AUC value among all prediction factors at 0.900, indicating its superior diagnostic potential compared to individual factors alone. The optimal threshold corresponding to this combined model was determined as 0.398 (with a sensitivity of 0.816, specificity of 0.900, and Youden index of 0.716).
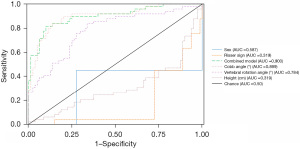
Table 7
Items | Youden index | Sensitivity | Specificity | Cut-off |
---|---|---|---|---|
Sex | 0.174 | 0.449 | 0.725 | 1.00 |
Risser sign | 0.000 | 0.00 | 1.00 | 5.00 |
Combined model | 0.716 | 0.816 | 0.90 | 0.398 |
Cobb angle (°) | 0.699 | 0.837 | 0.863 | 69.00 |
Vertebral rotation angle (°) | 0.499 | 0.837 | 0.662 | 18.10 |
Height (cm) | 0.020 | 0.02 | 1.00 | 175.00 |
Discussion
Although previous studies have examined the relationship between BMD and Cobb angle (8,10,15,18), the use of QCT for BMD measurement in studying the association with Cobb angle is still relatively novel. In our study, we observed a significant inverse correlation (coefficient =−0.663; P<0.001) between BMD and severity of Cobb angle in AIS patients. This inverse correlation suggests that as the spinal deformity, as characterized by the Cobb angle, intensifies, BMD tends to decline. This finding is consistent with previous studies conducted by Almomen et al. and Nishida et al. (15,18), in which patients with AIS with more severe Cobb angles had a significantly higher prevalence of low BMD, indicating that the extent of spinal deformity may adversely affect bone quality in patients with AIS. Additionally, a significant negative correlation (coefficient =−0.442; P<0.001) was observed between BMD and vertebral rotation angle. Our finding is consistent with the study conducted by Girardi et al., where they also found a significant negative correlation between BMD and the degree of rotation. Specifically, they reported an approximate 20% decrease in measured BMD when the lumbar spine was rotated from neutral to 60 degrees (19).
Our findings also demonstrated a positive correlation between BMD and height (coefficient =0.355; P<0.001) and between BMD and BMI (coefficient =0.199; P=0.02), which is consistent with the study by Hahn et al. (20). These correlations suggest that greater body size, indicated by increased height and BMI, may contribute to enhanced bone mass accrual during adolescence through increased mechanical loading on the bones. This aligns with the understanding that elevated mechanical stress resulting from higher body weight can stimulate bone formation and mineralization (21). However, the nonnormal distribution of height and BMI in our cohort necessitates a cautious interpretation of these results, considering the potential confounding factors such as genetic predispositions, nutritional status, physical activity levels, and hormonal variations, which were not accounted for in this study. Although we specifically selected AIS patients who had not undergone prior surgical or orthotic treatment to minimize potential treatment-related changes in BMD, these variables could potentially affect height, BMI, and BMD, thereby influencing the observed correlations.
In our study, we observed that females (143.78±45.46 g/cm2) exhibited a marginally higher average BMD compared to males (127.76±40.95 g/cm2) although though this difference was not statistically significant (P=0.052). This finding does not align with the broader trend reported by Wu et al. (10), in which males with severe AIS often exhibit more prevalent and severe low BMD compared to females. This discrepancy may suggest a complex interplay of hormonal, genetic, and potentially biomechanical factors, which warrants further investigation in elucidating the underlying mechanisms. In terms of skeletal maturity, our study revealed a significant variation in BMD across different Risser sign categories (P=0.002), which supports the established correlation between skeletal maturity and bone density. Specifically, as skeletal maturity increases, marked by advancing Risser sign values, a corresponding increase in bone density is typically expected due to the concurrent processes of bone growth and mineralization during adolescence (22). Interestingly, a slight decrease in BMD was observed at Risser sign 5 underscores the potential limitations of the Risser classification in capturing the full spectrum of maturational factors affecting bone density. This observation suggests that while Risser sign stages 1–4 correlate with increasing BMD, the transition to Risser sign 5 may involve additional physiological changes not fully accounted for by skeletal maturity alone (4,6,23). Besides, considering that Risser 0 encompasses the period from birth until the completion of the pubertal growth spurt, and Risser 5 indicates no further changes at the iliac crest (23), these stages encompass significantly broader developmental phases compared to the transitional Risser 1–4 stages (4,6). However, this broad categorization may obscure finer variations in BMD occurring over these extensive periods. Therefore, future studies should aim to dissect these broader categories further by implementing longitudinal designs capable of precisely tracking BMD changes throughout the entire spectrum of skeletal maturity. Besides, our study did not identify any statistically significant differences in BMD between thoracic and lumbar curve type (P=0.13). This finding is intriguing as it suggests that the specific spinal curvature may not exert a substantial impact on BMD in patients with AIS. This observation diverges from the findings of Chen et al. (24), who reported a significant influence of curve location on the structural characteristics of AIS curves, thereby highlighting the complexity of the disease’s impact on bone density, regardless of curve location. This finding underscores the necessity for further research to explore the nuanced variations in the BMD associations with different curve characteristics in AIS.
In the univariate analysis of predictors for low BMD, we found that height, Cobb angle, vertebral rotation angle, and Risser sign showed significant differences between the low BMD and normal bone mass groups (P<0.01 all). Furthermore, patients with low BMD tended to have lower height, higher Cobb angle and vertebral rotation angle, while higher Risser sign values were less common in the low BMD group, which is consistent with the findings reported by Schell et al. (25). Furthermore, our findings demonstrate that the Cobb angle is a significant risk factor for low BMD in patients with AIS, exhibiting a regression coefficient of 0.072 (P<0.001). The calculated OR of 1.075 indicates that for each degree increase in Cobb angle, there is a corresponding 7.5% higher likelihood of developing low BMD. This finding aligns with the study conducted by Almomen et al., where AIS patients with higher Cobb angles showed a statistically significant increased frequency of low BMD (15). Besides, our study revealed no significant correlations between BMD and biochemical indices of mineral metabolism, including K, Ca, P, and Fe (all P values >0.05). This finding is particularly noteworthy considering the intricate interplay of Ca and P metabolism in AIS, as explored in the study by Brzęczek et al. (26), who observed elevated levels of calcium and 25-hydroxy vitamin D and reduced parathyroid hormone (PTH) levels in patients with AIS. Furthermore, in a study conducted by Bala et al. on Turkish children with AIS, the authors collected multiple parameters including Ca, P, 25-hydroxyvitamin D (25-OH-D3), alkaline phosphatase (ALP), PTH, neutrophil-to-lymphocyte ratio (NLR), monocyte-to-lymphocyte ratio (MLR), platelet-to-lymphocyte ratio (PLR), complete blood counts, and hormone parameters. The findings revealed a significant correlation between PLR and the Cobb angle, as well as an association between vitamin D deficiency and low BMD. Additionally, the authors reported that complete blood count parameters can serve as predictors for BMD in adolescents (27). In contrast to their findings, our study did not identify a significant relationship between these biochemical markers and BMD. This discrepancy suggests that biomechanical factors might have exerted a more pronounced influence on BMD within our study population than did the biochemical aspects of mineral metabolism. Additionally, considering our study population consisted solely of Chinese AIS patients, ethnicity might also impact our findings. Our results underscore the multifaceted nature of determinants for BMD in AIS, emphasizing the necessity for further research to unravel the intricate balance between biomechanical and biochemical influences. Furthermore, future investigations could incorporate additional parameters pertaining to blood cell count in order to enhance the comprehensiveness of the study.
Our integrated predictive model, achieving an AUC of 0.900, demonstrated superior capability in identifying patients with AIS at risk for low BMD compared to individual parameters. This model’s efficacy highlights the necessity of a multifaceted approach that integrates various clinical and demographic factors for a comprehensive assessment of BMD in AIS. Importantly, the Cobb angle emerged as a significant individual predictor, with an AUC of 0.899, reflecting the direct influence of spinal curvature severity on BMD. In contrast, sex exhibited lower discriminatory power, with an AUC of 0.587, but underscores the gender-specific influences on BMD. Vertebral rotation angle and Risser sign both contributed to the accuracy of our model and emphasized the complexity of BMD variation in AIS beyond skeletal maturity, including aspects related to spinal deformity. By integrating these uniquely insightful parameters, our predictive tool surpasses the limited scope offered by single-factor analysis. Our model provides a foundation for clinical prediction of low BMD in patients with AIS, enabling clinicians to evaluate the presence of low BMD using easily accessible clinical data such as height and sex, in conjunction with X-ray and conventional CT images. This model proves particularly advantageous for medical institutions that do not extensively employ quantitative CT examinations when formulating clinic strategies for AIS patients, which could serve as a valuable reference tool.
Our research derives its robustness from employing QCT, a reliable technique for measuring BMD, in a well-characterized cohort of patients with AIS. However, the cross-sectional design of our study imposes certain limitations. While this design allowed for the analysis of data at a specific time point, it restricted our ability to establish causal relationships. Moreover, although our sample size is adequate for initial analysis, it may not fully represent the broader AIS population, potentially limiting the generalizability of our findings. Furthermore, the absence of a control group represents another limitation, which precludes direct comparisons with non-AIS individuals and potentially compromises the interpretation of BMD influences specific to AIS. Therefore, caution should be exercised when extrapolating our results to all patients with AIS due to the uneven distribution of certain variables, lack of longitudinal data, and a controlled healthy population.
To build upon our findings and address these limitations, future research should focus on longitudinal studies to monitor BMD and scoliosis progression over an extended period of time, particularly in the context of therapeutic interventions. Moreover, exploring molecular and genetic factors that contribute to BMD variations in AIS could reveal potential targets for pharmacological intervention. Further investigation into the role of nutrition, physical activity, and lifestyle factors in BMD among patients with AIS is also essential for developing comprehensive management strategies.
Our study confirms the negative association between spinal deformity severity and BMD in AIS, highlighting the significance of stature and gender in this relationship. The efficacy of our multifactor model in predicting low BMD in AIS patients underscores its potential in guiding clinical decisions. This model enables clinicians to better stratify patients based on their risk factors, facilitating personalized treatment strategies. By incorporating a comprehensive BMD assessment, clinicians can make more informed choices regarding therapeutic interventions, ultimately enhancing patient management and outcomes in AIS.
Conclusions
The results of our study demonstrated a significant inverse correlation between BMD and Cobb angle (coefficient =−0.663; P<0.001), as well as between BMD and vertebral rotation angle (coefficient =−0.442; P<0.001) in patients with AIS. Additionally, we observed positive correlations between BMD and height (coefficient =0.355; P<0.001), as well as between BMD and BMI (coefficient =0.199; P=0.02). Moreover, BMD exhibited a significant variation across different categories of Risser sign (P<0.01), with a general increase in BMD observed as the Risser sign values increased. Furthermore, we identified Cobb angle as a substantial risk factor for low BMD in patients with AIS, exhibiting a regression coefficient of 0.072 (P<0.001) and an odds ratio of 1.075, indicating that each degree increase in Cobb angle corresponds to a 7.5% higher likelihood of developing low BMD. Moreover, we developed a prediction model including height, sex, Cobb angle, vertebral rotation angle, and Risser sign to predict the presence of low BMD in AIS patients. The optimal threshold for this combined model was determined as 0.398 with high sensitivity (0.816) and specificity (0.900). This predictive tool can be valuable when QCT is not available.
Acknowledgments
Funding: This study was supported by
Footnote
Reporting Checklist: The authors have completed the STARD reporting checklist. Available at https://tp.amegroups.com/article/view/10.21037/tp-24-74/rc
Data Sharing Statement: Available at https://tp.amegroups.com/article/view/10.21037/tp-24-74/dss
Peer Review File: Available at https://tp.amegroups.com/article/view/10.21037/tp-24-74/prf
Conflicts of Interest: All authors have completed the ICMJE uniform disclosure form (available at https://tp.amegroups.com/article/view/10.21037/tp-24-74/coif). F.C. serves as General Secretary of European Paediatric Orthopaedic Society (EPOS), Deputy Editor at the Orthopaedics & Traumatology: Surgery & Research (OTSR), and Specialty Editor at the Journal of Pediatric Orthopaedics B (JPOB). The other authors have no conflicts of interest to declare.
Ethical Statement: The authors are accountable for all aspects of the work in ensuring that questions related to the accuracy or integrity of any part of the work are appropriately investigated and resolved. The study was conducted in accordance with the Declaration of Helsinki (as revised in 2013). The study was approved by the Medical Ethics Committee of The Third People’s Hospital of Chengdu (No. 2021-S-13) and informed consent was taken from all the patients’ guardians.
Open Access Statement: This is an Open Access article distributed in accordance with the Creative Commons Attribution-NonCommercial-NoDerivs 4.0 International License (CC BY-NC-ND 4.0), which permits the non-commercial replication and distribution of the article with the strict proviso that no changes or edits are made and the original work is properly cited (including links to both the formal publication through the relevant DOI and the license). See: https://creativecommons.org/licenses/by-nc-nd/4.0/.
References
- Dimitrijević V, Šćepanović T, Jevtić N, et al. Application of the Schroth Method in the Treatment of Idiopathic Scoliosis: A Systematic Review and Meta-Analysis. Int J Environ Res Public Health 2022;19:16730. [Crossref] [PubMed]
- Bottino L, Settino M, Promenzio L, et al. Scoliosis Management through Apps and Software Tools. Int J Environ Res Public Health 2023;20:5520. [Crossref] [PubMed]
- Ho EK, Upadhyay SS, Chan FL, et al. New methods of measuring vertebral rotation from computed tomographic scans. An intraobserver and interobserver study on girls with scoliosis. Spine (Phila Pa 1976) 1993;18:1173-7. [Crossref] [PubMed]
- Dimeglio A, Canavese F. The growing spine: how spinal deformities influence normal spine and thoracic cage growth. Eur Spine J 2012;21:64-70. [Crossref] [PubMed]
- Liu L, Xiu P, Li Q, et al. Prevalence of cardiac dysfunction and abnormalities in patients with adolescent idiopathic scoliosis requiring surgery. Orthopedics 2010;33:882. [Crossref] [PubMed]
- Dimeglio A, Canavese F. Progression or not progression? How to deal with adolescent idiopathic scoliosis during puberty. J Child Orthop 2013;7:43-9.
- Gullberg J, Sundh D, Johansson L, et al. The outcome of an automated assessment of trabecular pattern in intraoral radiographs as a fracture risk predictor. Dentomaxillofac Radiol 2022;51:20210483. [Crossref] [PubMed]
- Ramos O, Razzouk J, Chung JH, et al. Opportunistic assessment of bone density in patients with adolescent idiopathic scoliosis using MRI-based vertebral bone quality. J Clin Neurosci 2022;103:41-3. [Crossref] [PubMed]
- Bianchi ML. Osteoporosis in children and adolescents. Bone 2007;41:486-95. [Crossref] [PubMed]
- Wu Z, Zhu X, Xu L, et al. More Prevalent and Severe Low Bone-Mineral Density in Boys with Severe Adolescent Idiopathic Scoliosis Than Girls: A Retrospective Study of 798 Surgical Patients. J Clin Med 2023;12:2991. [Crossref] [PubMed]
- Gilmore A, Thompson GH. Radiographic evaluation of children and adolescents with a spinal deformity. Semin Musculoskelet Radiol 2000;4:349-59. [Crossref] [PubMed]
- Kellar K, Pandillapalli NR, Moreira AG. Calcium and Phosphorus: All You Need to Know but Were Afraid to Ask. Clin Perinatol 2023;50:591-606. [Crossref] [PubMed]
- Xu XM, Li N, Li K, et al. Discordance in diagnosis of osteoporosis by quantitative computed tomography and dual-energy X-ray absorptiometry in Chinese elderly men. J Orthop Translat 2018;18:59-64. [Crossref] [PubMed]
- Wang J, Zhou S, Chen S, et al. Prediction of osteoporosis using radiomics analysis derived from single source dual energy CT. BMC Musculoskelet Disord 2023;24:100. [Crossref] [PubMed]
- Almomen FA, Altaweel AM, Abunadi AK, et al. Determining the correlation between Cobb angle severity and bone mineral density in women with adolescent idiopathic scoliosis. J Taibah Univ Med Sci 2021;16:365-8. [Crossref] [PubMed]
- Chopra S, Larson AN, Kaufman KR, et al. Accelerometer based assessment of daily physical activity and sedentary time in adolescents with idiopathic scoliosis. PLoS One 2020;15:e0238181. [Crossref] [PubMed]
- Link TM, Kazakia G. Update on Imaging-Based Measurement of Bone Mineral Density and Quality. Curr Rheumatol Rep 2020;22:13. [Crossref] [PubMed]
- Nishida M, Yagi M, Suzuki S, et al. Persistent low bone mineral density in adolescent idiopathic scoliosis: A longitudinal study. J Orthop Sci 2023;28:1099-104. [Crossref] [PubMed]
- Girardi FP, Parvataneni HK, Sandhu HS, et al. Correlation between vertebral body rotation and two-dimensional vertebral bone density measurement. Osteoporos Int 2001;12:738-40. [Crossref] [PubMed]
- Hahn MH, Won YY. Bone Mineral Density and Fatty Degeneration of Thigh Muscles Measured by Computed Tomography in Hip Fracture Patients. J Bone Metab 2016;23:215-21. [Crossref] [PubMed]
- Shi W, Zhang Q. Association between Metabolic Syndrome and the Musculoskeletal System. Nutrients 2023;15:4475. [Crossref] [PubMed]
- Moon RJ, Citeroni NL, Aihie RR, et al. Early Life Programming of Skeletal Health. Curr Osteoporos Rep 2023;21:433-46. [Crossref] [PubMed]
- Cheung JPY, Luk KD. Managing the Pediatric Spine: Growth Assessment. Asian Spine J 2017;11:804-16. [Crossref] [PubMed]
- Chen ZQ, Zhao YF, He SS, et al. Factors as predictors for thoracic and thoracolumbar/lumbar structural curves in adolescent idiopathic scoliosis. Chin Med J (Engl) 2012;125:1439-42.
- Schell TL, Krueger D, Binkley N, et al. Opportunistic use of dual-energy X-ray absorptiometry to evaluate lumbar scoliosis. Arch Osteoporos 2021;16:38. [Crossref] [PubMed]
- Brzęczek M, Hyla-Klekot L, Kokot F, et al. Evaluation of Selected Parameters of Calcium and Phosphate Metabolism in Children with Adolescent Idiopathic Scoliosis. Ortop Traumatol Rehabil 2019;21:271-8. [Crossref] [PubMed]
- Bala MM, Bala KA. Association Among Complete Blood Count Parameters, Bone Mineral Density, and Cobb Angle in Cases of Adolescent Idiopathic Scoliosis. Med Sci Monit 2023;29:e940355. [Crossref] [PubMed]