Neuromuscular contributions to disability in children with cerebral palsy and the impact of dynamic stretching orthoses and therapeutic exercise interventions: a narrative review
Introduction
Cerebral palsy (CP) is the most common motor disability among children, occurring in two to three out of every 1,000 live births (1). CP results from an injury to the developing brain in utero, during delivery, or in early childhood. Though the cause of CP is often multifactorial, hypoxia, stroke, hypotension, and/or a cytokine cascade following an insult to the brain may contribute to CP (2). Depending on the location (pyramidal or extrapyramidal) and extent of cerebral dysfunction, a child with CP may experience mobility limitations.
Mobility is often impacted in children with CP. A child with CP’s mobility can vary greatly, spanning from independent ambulation without assistive devices to full reliance on caregiver assistance (3). Mobility impairments associated with CP encompass a broad spectrum of challenges affecting movement, balance, and coordination.
Ataxia associated with CP can result in unsteady movements, particularly during activities requiring precise control such as walking or standing (4). Additionally, muscle weakness and/or contractures may hinder weight-bearing and strength-dependent actions like climbing stairs or walking on uneven terrain.
Spasticity is a hallmark of CP and may also impact mobility in this population. Spasticity is defined as a velocity dependent increased resistance to passive muscle stretch and is a primary comorbidity in up to 88% of children with CP (5,6). Spasticity may induce stiffness and awkward movements, which may negatively impact mobility (7).
Despite the mobility-related challenges experienced by children with CP, orthotic intervention and therapeutic exercise techniques can be prescribed to improve function. Both static and dynamic orthoses are often incorporated into rehabilitation paradigms to ameliorate the neuromuscular manifestations of CP that contribute to disability. Orthoses can be tailored to the unique needs of each individual, offering a customized approach to addressing the specific challenges that may result in diminished mobility. By providing structural support and facilitating proper anatomical alignment, orthotic intervention is often recommended as part of a comprehensive treatment plan.
Therapeutic exercises compliment orthotic intervention and may also be prescribed to manage symptoms associated with CP (8). Aerobic exercise plays a critical role in improving cardiovascular health, especially for children with CP (8,9). This population often experiences challenges related to decreased mobility and muscle tone, factors that can adversely affect the cardiovascular system. Engaging in regular aerobic activities can help mitigate these risks and enhance overall well-being (10). Incorporating progressive resistive training into a therapeutic exercise program may also be beneficial, as increased muscular strength can improve a child with CP’s ability to perform daily activities and tasks, as well as enhancing overall physical function (11).
The synergy between orthotic intervention and therapeutic exercise is particularly pronounced in their collective impact on functional outcomes. By strategically incorporating these modalities into a multidisciplinary rehabilitation framework, healthcare professionals can address the diverse and dynamic nature of CP and optimize treatment outcomes (12). The tailored integration of an orthosis encourages ongoing structural support, while targeted exercises serve to reinforce neuromuscular pathways, fostering a more adaptive and functional movement repertoire (12-15).
Numerous studies have individually examined the neuromuscular dysfunction linked with CP, exploring its physiological underpinnings and its implications for disability. However, few reviews have examined the relationship between neuromuscular dysfunction, mobility, and rehabilitative frameworks.
Therefore, the objective of this review was to describe the impact of dynamic stretching orthoses and therapeutic exercise on range of motion (ROM), aerobic capacity, and mobility in the context of the neuromuscular contributions to activity limitation among children with CP. By synthesizing literature on these topics, healthcare professionals may apply a more comprehensive approach to patient care. We present this article in accordance with the Narrative Review reporting checklist (available at https://tp.amegroups.com/article/view/10.21037/tp-24-73/rc).
Methods
To address the specific aims, PubMed, Google Scholar, and Web of Science were searched using the terms ‘cerebral palsy’, ‘musculoskeletal’, ‘neuromuscular’, ‘spasticity’, ‘rehabilitation’, ‘exercise’, ‘aerobic’, and ‘orthosis’. A complete description of the search strategy is outlined in Table 1.
Table 1
Items | Specification |
---|---|
Date of search | August 15, 2021 |
Databases and other sources searched | PubMed, Web of Science, Google Scholar |
Search terms used | The keywords and search terms utilized included: ‘cerebral palsy’, ‘musculoskeletal’, ‘neuromuscular’, ‘spasticity’, ‘rehabilitation’, ‘exercise’, ‘aerobic’, and ‘orthosis’ |
Timeframe | Inception to August 15, 2021 |
Inclusion and exclusion criteria | The inclusion criteria comprised original cross-sectional and longitudinal research studies published in English within the past 20 years, concentrating on children and adolescents aged 2 to 18 years with a diagnosis of spastic CP. Included studies featured experimental interventions involving orthotic intervention and/or therapeutic exercise, particularly focusing on aerobic and progressive resistive training, and required appropriate comparison groups assessing the effects of dynamic stretching orthoses or therapeutic exercise. Outcomes of interest encompassed measures related to lower extremity ROM and assessments of aerobic capacity, muscle strength, and mobility |
Exclusion criteria encompassed studies failing to meet the inclusion criteria based on title, abstract, and publication date, as well as those involving adults or individuals without a CP diagnosis. Studies focusing solely on interventions other than dynamic stretching orthoses or therapeutic exercise, case reports, editorials, non-peer-reviewed publications, conference abstracts, letters, studies lacking methodological rigor, and animal studies were also excluded, along with publications in languages other than English | |
Selection process | K.R.L. and J.R. evaluated the search results and conducted a comprehensive manual review of the full texts. Each study was independently assessed for inclusion or exclusion, with reasons for exclusion documented based on predefined criteria. In cases of disagreement, a third reviewer, A.S., was engaged to independently resolve any disputes |
CP, cerebral palsy; ROM, range of motion.
Inclusion criteria
The inclusion criteria encompassed original cross-sectional and longitudinal research studies published in the English language. All studies were published within the last 20 years and focused on children and adolescents aged between 2 and 18 years with a diagnosis of spastic CP. The decision to focus solely on spastic CP was driven by its high prevalence, aiming to enhance the review’s clinical applicability and streamline the identification of relevant articles describing the interventions of interest. Articles were considered if the experimental intervention involved orthotic intervention and/or therapeutic exercise, with an emphasis on aerobic and progressive resistive training. The decision to prioritize aerobic and progressive resistive training stemmed from their widespread clinical use for addressing key challenges associated with the target population. Studies with appropriate comparison groups, assessing the impact of dynamic stretching orthoses or therapeutic exercise, were included. The outcomes of interest included measures related to lower extremity ROM and assessments of aerobic capacity, muscle strength, and mobility.
Exclusion criteria
Articles were excluded if it was determined from the title, abstract, and publication date that the study failed to meet the inclusion criteria. Studies involving adults or individuals without a diagnosis of CP were excluded from the review. Additionally, studies focusing solely on interventions other than dynamic stretching orthoses or therapeutic exercise were not included. Case reports, editorials, non-peer-reviewed publications, conference abstracts, letters, studies with insufficient methodological rigor, and animal studies were also excluded. Studies published in languages other than English were excluded from the review. These exclusion criteria were designed to ensure a focused and comprehensive synthesis of literature related to the narrative review’s specific aims.
Results
The initial search yielded 303 manuscripts containing the search terms. The title and abstract of each manuscript were evaluated, resulting in the exclusion of 182 articles not meeting the inclusion criteria. The full text of the remaining 121 manuscripts were analyzed, and nine studies meeting the inclusion criteria were included the narrative review (Figure 1). Three longitudinal, and/or randomized controlled trials investigating the efficacy of dynamic stretching orthoses for ROM techniques were included. A total of six longitudinal and one cross-sectional studies were reviewed that investigated the impact of aerobic and strength training on various biomarkers and gait parameters among children with CP.
CP neuromuscular pathophysiology
The neuromuscular intricacies inherent with CP significantly shape the disability landscape experienced by children with CP. Among the myriad of challenges, muscle fatigue and weakness stand out as prominent factors influencing functional limitations and mobility impairments in this population. As a result, rehabilitation efforts often focus on maintaining and/or increasing strength and joint ROM (Figure 2).
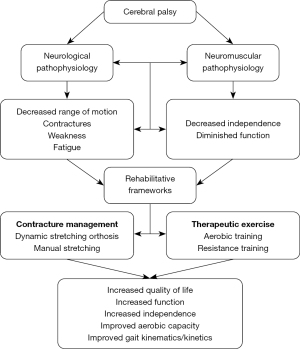
Muscle fatigue and weakness are common among individuals with CP. Children with spastic CP fatigue more rapidly during walking at a self-selected speed compared to their typically developing peers (16). This may be linked to greater lower extremity muscle fatigability in children with CP, as evident by larger increases in gastrocnemius and soleus muscle electromyographic (EMG) signals (17,18). Furthermore, isokinetic quadricep EMG data collected by Leunkeu et al. also supports that children with CP experience muscle fatigue at elevated rates compared to age-matched controls (19).
Differences in muscle fiber type have been observed in children with CP (20). Skeletal muscles typically contain a combination of type I (slow) and type II (fast) muscle fibers, with proportions varying according to the muscle’s function. Predominance of a specific muscle fiber type may influence muscle fatigue because type I muscle fibers are more fatigue resistant whereas type II muscle fibers are more fatigable. In children with CP, altered neural input secondary to cerebral damage may impact the differentiation of type I and type II muscle fibers (21). While no consensus exists regarding the predominate muscle fiber type in children with CP, it has been suggested that individuals with spasticity present with increased type I muscle fibers and/or atrophy of type II muscle fibers which may contribute to decreased strength (22,23).
Differences in a muscle’s extracellular matrix have also been connected to muscle weakness and fatiguability among children with CP. Muscle extracellular matrix plays an essential role in transmitting the forces produced by muscle fibers and setting the passive stiffness of muscle tissue. Evidence suggests that the muscle extracellular matrix may be fibrotic and exhibit considerable collagen accumulation in children with CP, which can negatively impact ROM (24).
Because CP has few disease-modifying interventions, management of neuromuscular comorbidities comprises the majority of treatment options. The optimal treatment of symptoms is highly dependent on clinical presentation. Neural lesions contributing to muscle spasticity, weakness, and contractures can lead to a myriad of musculoskeletal abnormalities and reduced motor function. Overstretched sarcomeres combined with shortened muscle tissue commonly result in longer, anti-phasic sarcomere orientation (25). As a result, the optimal sarcomere length-tension ratio may be distorted, leading to decreased muscle force production (25). Additional muscular adaptations common among children with CP include atrophy, contractures, and fibrosis (26). Moreover, children with CP have been shown to co-activate antagonist muscles during upper and lower extremity movements, resulting in increased energy expenditure and fatigue (27). Based on these factors, a primary objective of rehabilitation typically focuses on maintaining and/or improving mobility through muscle ROM and therapeutic exercise programs.
ROM and contracture management
Stretching programs are commonly implemented to treat lower extremity contractures. The primary goal of many stretching programs is to lengthen the shortened muscle, improving joint ROM for functional movement (27). By restoring muscle length, independence may be improved, and surgical intervention may be delayed or avoided.
Prolonged dynamic stretching, where an orthosis maintains a low load stretch for an extended period, is commonly prescribed independently or in conjunction with physical therapy, anti-spasticity medications, or active stretching (28,29). The therapeutic benefit ascribed to dynamic stretching orthoses may result in improved ROM and overall quality of life in patients with contractures due to causes other than CP (i.e., prolonged immobilization, excessive arthrofibrosis, etc.) (29). While these devices are also commonly implemented among children with CP, evidence supporting the efficacy of dynamic stretching orthotic intervention for enhancing lower extremity ROM is limited and nuanced.
Dynamic stretching orthoses
Three original experimental research articles published within the last 7 years have explored the effects of dynamic stretching orthoses in children with CP (Table 2) (30-32). Of these studies, one randomized controlled trial investigated ankle dorsiflexion ROM improvement, compliance, and functional outcomes while using a knee ankle foot orthosis (KAFO) with dynamic Ultraflex® ankle joints. In the study, participants were required to wear the orthosis for at least 6 hours, every other night, for 1 year (30). Ankle dorsiflexion ROM, ankle-foot and knee angle during gait, gross motor function, and wearing time measurements were obtained at 3-month intervals. No significant differences in ankle-foot dorsiflexion ROM werew reported between the experimental and control groups. Furthermore, participants wore the device an average of 3.2 out of 6 hours prescribed, suggesting low compliance.
Table 2
Author, year | Therapeutic modality | Study type | Participant cohort | Intervention | Conclusions |
---|---|---|---|---|---|
Samsir et al., 2020 (15) | Therapist-guided progressive strength training | Single arm pre- and post-test design | Ten independently ambulatory children with spastic CP | Participants completed 6 months of exercise therapy, twice a week for 1 hour per session. Resistance was increased as functional strength increased | Guided progressive resistance exercise therapy improved motor abilities and white matter connectivity in children with CP |
Maas et al., 2014 (30) | KAFO | Randomized controlled trial | Twenty-eight ambulatory children with spastic CP (experimental group, n=15; control group, n=13) | Utilization of a KAFO equipped with Ultraflex ankle joints for ≥6 hours every other night for 1 year | KAFOs are poorly tolerated and not beneficial in preventing reduced ankle foot dorsiflexion ROM in children with CP |
Chen et al., 2017 (31) | Ankle foot orthosis | Clinical trial/experimental study | Fifty-eight children with CP and 30 typically developing children | Twenty-eight children with CP treated with splint assisted AFO correction, 30 children with CP treated with static AFO correction | Adjustable splint assisted AFOs are an effective treatment for plantar flexion contractures in children with CP |
Hösl et al., 2015 (32) | Ankle foot orthosis | Clinical trial/experimental study | Seventeen independently ambulant children with CP with a GMFCS I or II classification and non-rigid equinus and 17 typically developing children | Utilization of an articulated AFO aligned in maximum passive dorsiflexion. Nine of 17 children with CP wore the brace day and night. Eight of 17 wore the brace only at night | No significant gains of muscle belly or tendon length between groups. Fascicle length shortened, and muscle bulk decreased in the AFO group. Gait improvements were noted with brace wear |
Hanssen et al., 2022 (33) |
Progressive resistance training exercise program | Randomized controlled trial | Forty-nine children with spastic CP | The intervention group underwent a PRT program. comprised 3–4 sessions per week, with exercises performed in three sets of 10 repetitions, targeting 60–80% of the participants’ one-repetition maximum. The control group received standard care, involving regular physiotherapy sessions |
Significant improvements in knee flexion strength, unilateral heel raises, knee extension, and plantar flexion isometric strength, as well as muscle volume and functional strength measures were noted. Muscle morphology showed minimal changes. Supervised home-based progressive resistance training programs can effectively enhance muscle strength and function in children CP |
Scholtes et al., 2010 (34) | Therapist-guided progressive circuit strength training | Randomized controlled trial | Fifty-one children with spastic unilateral and bilateral CP with GMFCS levels I–III | The intervention group participants completed a five-exercise circuit comprised of leg press and functional exercises, three times per week, for 12 weeks | A 12-week progressive exercise program resulted in increased muscle strength, but did not translate to improved mobility in children with CP |
Eek et al., 2008 (35) |
Home- and group-based lower extremity strength training exercises | Clinical trial/experimental study | Sixteen children with spastic diplegic CP with GMFCS levels I–II | Participants completed eight weeks of strength training, three times per week. Exercises were completed with body wight, weight cuffs, and exercises bands | Muscle strength and GMFCS scores increased, muscle velocity was unchanged, stride length increased, cadence was reduced. Eight weeks of strength training improved muscle strength and gait function |
Unnithan et al., 2007 (36) | Aerobic interval and strength training program | Longitudinal pre-posttest experimental study | Seven adolescents with spastic diplegic CP. Each participant was able to walk, with or without walking aids | Participants completed 12-week aerobic interval and strength training program, three times per week, for 70 minutes per session. The aerobic training required the subjects to perform 60-m uphill walking repetitions | Application of a 12-week strength and aerobic interval training program can reduce oxygen cost of arm cranking, improve aerobic capacity, and motor function in children with CP |
Abo-zaid et al., 2024 (37) | Aerobic exercise training | Randomized controlled trial | Sixty children between the ages of 7 and 11 years with left-sided hemiplegic CP | Participants were randomly assigned to two groups: control and aerobic exercise. The control group received standard physical therapy for 1 hour, while the aerobic exercise group combined 30 minutes of standard therapy with 30 minutes of moderate-intensity cycling on a bicycle ergometer |
The exercise group demonstrated significant enhancements in Flanker and Stroop response accuracy (P=0.001) and notable reductions in Flanker and Stroop congruent reaction times (P<0.05). There were no significant disparities between the two groups regarding Flanker and Stroop incongruent reaction times (P>0.05) |
CP, cerebral palsy; KAFO, knee ankle foot orthosis; ROM, range of motion; AFO, ankle foot orthosis; GMFCS, Gross Motor Function Classification System; PRT, Passive Resistive Training.
Similar conclusions were drawn in a review by Wiart et al. (27). Based on the seven articles reviewed, the authors concluded that mechanisms behind muscle contractures in children with CP were unclear, and additional research was needed to determine the efficacy of stretching practices in this group. The importance of fitness and activity participation was stressed to promote optimal mobility outcomes in this population.
Contrary to these findings, Chen et al. suggested that utilization of an adjustable splint-assisted ankle-foot orthosis (AFO) may effectively reduce plantarflexion contractures in children with CP (31). After a 1-year treatment program featuring either a dynamic stretching AFO or manual stretching, improvements in heel/forefoot ratios among the cohort receiving the splint-assisted AFO were reported at 6- and 12-month intervals. These findings suggest that dynamic stretching AFOs may be a viable option for increasing dorsiflexion ROM.
Hösl et al. also examined the effects of positional AFOs on gastrocnemius contractures in children with CP (32). Seventeen ambulant children with CP presenting with calf muscle contractures and 17 untreated, typically developing children participated in the protocol which involved utilizing an articulated AFO. Children with CP were analyzed before and after 16±4 weeks of AFO usage. Prior to introducing the AFO, children with CP exhibited less extensibility in the medial gastrocnemius muscle-tendon unit compared to the typically developing children. When subjected to the same amount of stretch, children with CP presented with shorter muscle belly and fascicle length. Further, spastic fascicles among the children with CP demonstrated significantly less extensibility than those in the control group. The study reported that AFO utilization resulted in increased passive flexed-knee dorsiflexion, improved walking speed, and increased foot lift during the swing phase of gait. After AFO utilization, the authors noted that the gastrocnemius muscle belly and tendon length largely remained unchanged, but the muscle fascicles shortened further, and overall muscle thickness decreased.
Hösl et al.’s conclusions suggest that AFO utilization may improve function but may also lead to a loss of sarcomeres in series, potentially explaining the shortened fascicles. To promote gastrocnemius muscle growth, the authors speculated that AFOs might need to extend the knee, or that complementary training may be warranted to counteract the device’s immobilizing effects. Overall, the study highlighted both the benefits and potential drawbacks of AFOs in managing muscle contracture and improving function in children with CP.
Based on these findings, the efficacy of dynamic stretching AFOs for improving function and mobility among children with CP is largely inconclusive. While these devices may provide therapeutic benefit when utilized in conjunction with manual stretching and prolonged therapy, extended brace wear prescription and low compliance may limit value, especially when considering nighttime devices. Further longitudinal, large cohort studies are necessary to determine the utility of dynamic stretching orthoses for improving ROM in children with CP.
Exercise recommendations for individuals with CP
In addition to reduced joint ROM, individuals with CP may also exhibit reduced cardiorespiratory endurance, muscle strength and endurance, and overall physical activity. Reduced physical activity may result in increased risk for metabolic disorders and all-cause mortality (38). As discussed previously, children with CP tend to present with decreased muscular strength secondary to neuromuscular and musculoskeletal deficiencies (39). Depending on the child’s clinical presentation, comparing exercise types among children with CP can be difficult. In addition, the origin of inherent weakness and influence of altered muscle structures in children with CP remains ambiguous. However, therapeutic exercises may provide neurologic and musculoskeletal benefits in this population (Table 2).
A recent study by Hanssen et al. investigated the effects of a progressive resistance training program on lower limb muscle strength, morphology, and gross motor function in children with spastic CP (33). Forty-nine participants were randomized into intervention and control groups. The intervention group underwent a 12-week progressive resistive training program, while the control group received usual care. Results indicated significant improvements in knee flexion strength and unilateral heel raises in the intervention group. Additionally, improvements were observed in knee extension and plantar flexion isometric strength, muscle volume, and functional strength measures. Muscle morphology remained largely unchanged. The study concluded that the supervised home-based progressive resistive training programs may effectively improve muscle strength and function in children with spastic CP without adverse effects on muscle properties (33).
There is evidence that therapist-guided progressive strength training may improve motor abilities and strengthen motor pathways in children with CP (15). In a study by Samsir et al., children with CP participated in 1 hour of guided strength training, twice per week. At the completion of the 6-month protocol, motor abilities improved, and neural white matter connections were strengthened. These findings provide insight into potential neuromuscular benefits of strength training among children with CP.
The benefits of functional progressive resistance exercises for children with spastic unilateral and bilateral CP have also been investigated (34). After a 12-week guided training program consisting of functional upper and lower extremity exercises, children with CP demonstrated a ≥14% increase in muscle strength compared to a control group receiving standard care. While these results are encouraging, muscle strength improvements did not translate to improved mobility among the cohort, limiting clinical applicability.
Contrary to these findings Eek et al. noted improvements in gait function in children with CP after an 8-week free weight, exercise band, and body weight strength training regimen (35). At the program’s conclusion, participants demonstrated increased muscle strength, stride length, and functional level. These findings suggest that the 8-week strengthening program was effective for increasing muscle strength and gait parameters in children with CP, which may translate into increased independence.
Core stability influences balance and coordination, which may be comprosed in children with CP. Elshafey et al. assessed the impact of a core stability exercise program on balance, coordination, and ataxia severity in children with cerebellar ataxic CP (40). Forty children with CP were randomly assigned to a control or intervention group. The control group received standard physical therapy, while the intervention group received an additional 30-minute core stability program in conjunction with standard therapy. Results indicated significant improvements in ataxia severity, balance, and coordination in both groups, with greater enhancements observed in the intervention group. This suggests that incorporating a core stability program into standard physical therapy can enhance balance and coordination in children with cerebellar ataxic CP, further supporting the efficacy of therapeutic exercises to improve function in children with CP.
Incongruities between aerobic fitness have been noted when comparing children with CP to typically developing children (41,42). Peak oxygen uptake (VO2peak) is considered the best indicator of aerobic fitness. The three studies in this review each compared VO2peak between children with CP and healthy controls. Based on the studies, it is apparent that children with CP present with decreased aerobic capacity compared to children without CP. Furthermore, children with CP also present with lower maximal oxygen uptake (VO2max) and higher ventilatory equivalent for oxygen compared to their healthy counterparts. Implications of these findings may be attributed to inefficient ventilation, compromised circulation, or local fatigue in spastic limb muscles (42).
While evidence supports that aerobic capacity may be diminished in children with CP, Unnithan et al. found that a 12-week aerobic interval arm-crank ergometer program combined with strength training can reduce oxygen cost, improve aerobic capacity, and enhance gross motor function in children with CP (36). Consequently, the application of an aerobic training routine may have a positive impact on aerobic fitness in children with CP. Although the author’s findings support this notion, further research is warranted to determine optimal aerobic and strength training exercise prescription in individuals with CP.
Research also suggests that aerobic exercise may enhance inhibitory control and executive function in children with CP (37). A study by Abo-zaid et al. investigated the impact of aerobic exercise training on inhibitory control in children with hemiplegic CP through a single-blind randomized controlled trial involving 60 children with CP, aged 7–11 years. One group underwent standard physical therapy, while the other combined it with moderate-intensity continuous exercise on a bike ergometer. After 12 weeks, the exercise group demonstrated significant improvements in response accuracy and reaction times compared to the control group. However, no significant differences were observed in certain reaction times between the two groups. These findings suggest that aerobic exercise holds potential for enhancing inhibitory control in children with hemiplegic CP (37).
These findings emphasize the importance of integrating aerobic exercise into rehabilitation programs for children with CP to optimize their physical and cognitive development. However, further research is necessary to refine exercise protocols and determine the most effective approaches for maximizing outcomes in this population. Overall, the evidence underscores the potential of aerobic exercise as a valuable component of comprehensive care for children with CP, offering hope for improved functional outcomes and quality of life.
Limitations
While careful consideration was given during the composition of this review, there are several limitations that are noteworthy. First, we chose to limit our description of the pathophysiology behind CP strictly to the neuromuscular contributions to disability. While it is recognized that many additional systems are impacted by CP, the decision to base this review on the neurological contributions to disability was made because deficiencies inherent with this system is one of the primary contributors to decreased independence and mobility in this group. Furthermore, additional stretching techniques including manual stretching are commonly implemented in clinical practice but were omitted in this review.
Conducting longitudinal research featuring children with CP is challenging due to experimental mortality, compliance, and the unique clinical presentation of each child. However, methods to ameliorate these threats to validity may include providing custom orthoses or personal exercise programs for free for study participants.
Conclusions
An appreciation of the neurological and musculoskeletal mechanisms behind CP is warranted when considering acute and long-term treatment strategies for children with CP. Children with CP often require a multidisciplinary approach to care to ensure optimal treatment outcomes. While the underlying cause of CP is non-progressive, without proper management of neurological and musculoskeletal comorbidities, independence and quality of life may be diminished.
Regardless of clinical presentation, developing optimal rehabilitation frameworks is essential for maximizing quality of life and independence. Based on current evidence, the effectiveness of dynamic stretching orthoses for contracture management among children with CP is inconclusive. Due to the long wear times and cumbersome nature associated with dynamic stretching compliance is typically low. As such, additional high-quality, longitudinal research is necessary to further validate the effectiveness of these devices.
Though evidence on the efficacy of dynamic stretching orthoses is largely inconclusive, it is clear that aerobic and progressive resistance training is effective for maintaining functional status. By incorporating progressive resistive and aerobic exercises into a rehabilitation plan, muscle fatigue, mobility, and aerobic capacity can be improved. As such, clinicians should consider resistance and aerobic exercise prescription as part of a long-term treatment plan for children with CP.
Acknowledgments
Funding: None.
Footnote
Reporting Checklist: The authors have completed the Narrative Review reporting checklist. Available at https://tp.amegroups.com/article/view/10.21037/tp-24-73/rc
Peer Review File: Available at https://tp.amegroups.com/article/view/10.21037/tp-24-73/prf
Conflicts of Interest: All authors have completed the ICMJE uniform disclosure form (available at https://tp.amegroups.com/article/view/10.21037/tp-24-73/coif). The authors have no conflicts of interest to declare.
Ethical Statement: The authors are accountable for all aspects of the work in ensuring that questions related to the accuracy or integrity of any part of the work are appropriately investigated and resolved.
Open Access Statement: This is an Open Access article distributed in accordance with the Creative Commons Attribution-NonCommercial-NoDerivs 4.0 International License (CC BY-NC-ND 4.0), which permits the non-commercial replication and distribution of the article with the strict proviso that no changes or edits are made and the original work is properly cited (including links to both the formal publication through the relevant DOI and the license). See: https://creativecommons.org/licenses/by-nc-nd/4.0/.
References
- Vitrikas K, Dalton H, Breish D. Cerebral Palsy: An Overview. Am Fam Physician 2020;101:213-20. [PubMed]
- Dammann O, Leviton A. Role of the fetus in perinatal infection and neonatal brain damage. Curr Opin Pediatr 2000;12:99-104. [Crossref] [PubMed]
- Palisano RJ, Tieman BL, Walter SD, et al. Effect of environmental setting on mobility methods of children with cerebral palsy. Dev Med Child Neurol 2003;45:113-20. [Crossref] [PubMed]
- Monbaliu E, De Cock P, Mailleux L, et al. The relationship of dystonia and choreoathetosis with activity, participation and quality of life in children and youth with dyskinetic cerebral palsy. Eur J Paediatr Neurol 2017;21:327-35. [Crossref] [PubMed]
- Hollung SJ, Bakken IJ, Vik T, et al. Comorbidities in cerebral palsy: a patient registry study. Dev Med Child Neurol 2020;62:97-103. [Crossref] [PubMed]
- Sanger TD, Delgado MR, Gaebler-Spira D, et al. Classification and definition of disorders causing hypertonia in childhood. Pediatrics 2003;111:e89-97. [Crossref] [PubMed]
- Shamsoddini A, Amirsalari S, Hollisaz MT, et al. Management of spasticity in children with cerebral palsy. Iran J Pediatr 2014;24:345-51. [PubMed]
- Rogers A, Furler BL, Brinks S, et al. A systematic review of the effectiveness of aerobic exercise interventions for children with cerebral palsy: an AACPDM evidence report. Dev Med Child Neurol 2008;50:808-14. [Crossref] [PubMed]
- Nsenga AL, Shephard RJ, Ahmaidi S. Aerobic training in children with cerebral palsy. Int J Sports Med 2013;34:533-7. [Crossref] [PubMed]
- Teixeira JS, Santos LMLJ, Santos NL, et al. A case study of the effect of cardiovascular training on cerebral palsy. Fisioterapia em Movimento 2016;29:713-21. [Crossref]
- Taylor NF, Dodd KJ, Baker RJ, et al. Progressive resistance training and mobility-related function in young people with cerebral palsy: a randomized controlled trial. Dev Med Child Neurol 2013;55:806-12. [Crossref] [PubMed]
- Damiano DL. Rehabilitative therapies in cerebral palsy: the good, the not as good, and the possible. J Child Neurol 2009;24:1200-4. [Crossref] [PubMed]
- Aboutorabi A, Arazpour M, Ahmadi Bani M, et al. Efficacy of ankle foot orthoses types on walking in children with cerebral palsy: A systematic review. Ann Phys Rehabil Med 2017;60:393-402. [Crossref] [PubMed]
- Wright E, DiBello SA. Principles of Ankle-Foot Orthosis Prescription in Ambulatory Bilateral Cerebral Palsy. Phys Med Rehabil Clin N Am 2020;31:69-89. [Crossref] [PubMed]
- Samsir MS, Zakaria R, Razak SA, et al. Six Months Guided Exercise Therapy Improves Motor Abilities and White Matter Connectivity in Children with Cerebral Palsy. Malays J Med Sci 2020;27:90-100. [Crossref] [PubMed]
- Eken MM, Lamberts RP, Koschnick S, et al. Lower Extremity Strength Profile in Ambulatory Adults with Cerebral Palsy and Spastic Diplegia: Norm Values and Reliability for Hand-Held Dynamometry. PM R 2020;12:573-80. [Crossref] [PubMed]
- Eken MM, Dallmeijer AJ, Houdijk H, et al. Muscle fatigue during repetitive voluntary contractions: a comparison between children with cerebral palsy, typically developing children and young healthy adults. Gait Posture 2013;38:962-7. [Crossref] [PubMed]
- Eken MM, Braendvik SM, Bardal EM, et al. Lower limb muscle fatigue during walking in children with cerebral palsy. Dev Med Child Neurol 2019;61:212-8. [Crossref] [PubMed]
- Leunkeu AN, Keefer DJ, Imed M, et al. Electromyographic (EMG) analysis of quadriceps muscle fatigue in children with cerebral palsy during a sustained isometric contraction. J Child Neurol 2010;25:287-93. [Crossref] [PubMed]
- Verschuren O, Smorenburg ARP, Luiking Y, et al. Determinants of muscle preservation in individuals with cerebral palsy across the lifespan: a narrative review of the literature. J Cachexia Sarcopenia Muscle 2018;9:453-64. [Crossref] [PubMed]
- Rose J, Haskell WL, Gamble JG, et al. Muscle pathology and clinical measures of disability in children with cerebral palsy. J Orthop Res 1994;12:758-68. [Crossref] [PubMed]
- Marbini A, Ferrari A, Cioni G, et al. Immunohistochemical study of muscle biopsy in children with cerebral palsy. Brain Dev 2002;24:63-6. [Crossref] [PubMed]
- Ito J, Araki A, Tanaka H, et al. Muscle histopathology in spastic cerebral palsy. Brain Dev 1996;18:299-303. [Crossref] [PubMed]
- Smith LR, Pichika R, Meza RC, et al. Contribution of extracellular matrix components to the stiffness of skeletal muscle contractures in patients with cerebral palsy. Connect Tissue Res 2021;62:287-98. [Crossref] [PubMed]
- Mathewson MA, Ward SR, Chambers HG, et al. High resolution muscle measurements provide insights into equinus contractures in patients with cerebral palsy. J Orthop Res 2015;33:33-9. [Crossref] [PubMed]
- Kalkman BM, Bar-On L, O'Brien TD, et al. Stretching Interventions in Children With Cerebral Palsy: Why Are They Ineffective in Improving Muscle Function and How Can We Better Their Outcome? Front Physiol 2020;11:131. [Crossref] [PubMed]
- Wiart L, Darrah J, Kembhavi G. Stretching with children with cerebral palsy: what do we know and where are we going? Pediatr Phys Ther 2008;20:173-8. [Crossref] [PubMed]
- Pin T, Dyke P, Chan M. The effectiveness of passive stretching in children with cerebral palsy. Dev Med Child Neurol 2006;48:855-62. [Crossref] [PubMed]
- Furia JP, Willis FB, Shanmugam R, et al. Systematic review of contracture reduction in the lower extremity with dynamic splinting. Adv Ther 2013;30:763-70. [Crossref] [PubMed]
- Maas J, Dallmeijer A, Huijing P, et al. A randomized controlled trial studying efficacy and tolerance of a knee-ankle-foot orthosis used to prevent equinus in children with spastic cerebral palsy. Clin Rehabil 2014;28:1025-38. [Crossref] [PubMed]
- Chen W, Liu X, Pu F, et al. Conservative treatment for equinus deformity in children with cerebral palsy using an adjustable splint-assisted ankle-foot orthosis. Medicine (Baltimore) 2017;96:e8186. [Crossref] [PubMed]
- Hösl M, Böhm H, Arampatzis A, et al. Effects of ankle-foot braces on medial gastrocnemius morphometrics and gait in children with cerebral palsy. J Child Orthop 2015;9:209-19. [Crossref] [PubMed]
- Hanssen B, Peeters N, De Beukelaer N, et al. Progressive resistance training for children with cerebral palsy: A randomized controlled trial evaluating the effects on muscle strength and morphology. Front Physiol 2022;13:911162. [Crossref] [PubMed]
- Scholtes VA, Becher JG, Comuth A, et al. Effectiveness of functional progressive resistance exercise strength training on muscle strength and mobility in children with cerebral palsy: a randomized controlled trial. Dev Med Child Neurol 2010;52:e107-13. [Crossref] [PubMed]
- Eek MN, Tranberg R, Zügner R, et al. Muscle strength training to improve gait function in children with cerebral palsy. Dev Med Child Neurol 2008;50:759-64. [Crossref] [PubMed]
- Unnithan VB, Katsimanis G, Evangelinou C, et al. Effect of strength and aerobic training in children with cerebral palsy. Med Sci Sports Exerc 2007;39:1902-9. [Crossref] [PubMed]
- Abo-Zaid NA, El-Gendy AM, Hewidy I, et al. Influence of aerobic exercise on inhibitory control of executive functions in children with hemiplegic cerebral palsy: A randomized controlled trial. Clin Rehabil 2024;38:337-46. [Crossref] [PubMed]
- Lear SA, Hu W, Rangarajan S, et al. The effect of physical activity on mortality and cardiovascular disease in 130 000 people from 17 high-income, middle-income, and low-income countries: the PURE study. Lancet 2017;390:2643-54. [Crossref] [PubMed]
- Malaiya R, McNee AE, Fry NR, et al. The morphology of the medial gastrocnemius in typically developing children and children with spastic hemiplegic cerebral palsy. J Electromyogr Kinesiol 2007;17:657-63. [Crossref] [PubMed]
- Elshafey MA, Abdrabo MS, Elnaggar RK. Effects of a core stability exercise program on balance and coordination in children with cerebellar ataxic cerebral palsy. J Musculoskelet Neuronal Interact 2022;22:172-8. [PubMed]
- Verschuren O, Takken T. Aerobic capacity in children and adolescents with cerebral palsy. Res Dev Disabil 2010;31:1352-7. [Crossref] [PubMed]
- Hoofwijk M, Unnithan V, Bar-Or O. Maximal treadmill performance of children with cerebral palsy. Pediatr Exerc Sci 1995;7:305-13. [Crossref]