A case report of spastic diplegic cerebral palsy in a late preterm child with hypoplastic left heart syndrome
Highlight box
Key findings
• This is an interesting case report of spastic diplegic cerebral palsy in a late preterm infant with critical congenital heart disease (CHD). Spastic diplegic cerebral palsy is uncommon in late preterm children; however, developmental surveillance enabled the identification of this condition.
• Motor delays can occur in children with conditions including CHD and cerebral palsy. Because this child had CHD, his motor delay in infancy was not unexpected; it was through developmental surveillance that ongoing motor deficits led to further evaluation, and his cerebral palsy was identified and treated.
What is known and what is new?
• Children with CHD are at high risk for neurodevelopmental disabilities and have an evolving neurodevelopment trajectory. Their maximal motor impairment occurs in infancy, their behavioral problems are first noted in toddlerhood, and they have learning disabilities during school age years.
• This case report also illustrates the importance of considering brain dysmaturity in infants with CHD, how brain dysmaturity contributes to neurodevelopment, and what diagnostic dilemmas may arise when evaluating and treating these formerly critically ill children.
What is the implication, and what should change now?
• With the evolution of neurodevelopmental trajectories in children with CHD, continued developmental surveillance is imperative for identification of persistent motor delays and risk for cerebral palsy.
• Research on brain dysmaturity and selective vulnerability in children with CHD should continue, with a focus on etiology and effects of cerebral palsy and other neurodevelopmental disabilities in this population.
Introduction
Congenital heart disease (CHD) is the most common birth defect, affecting 8 per 1,000 live births annually (1). One example of CHD is hypoplastic left heart syndrome, which accounts for 2–3% of all CHD (2). Children with hypoplastic left heart syndrome are at high risk for neurodevelopmental disabilities (NDD), a group of conditions that can affect cognition, language, behavior, and mobility (3).
In infancy, individuals with CHD often have abnormal neurologic findings, including hypotonia (low tone) and motor delay. As they mature, they commonly exhibit additional delays in language skills, cognition, and social-emotional development that persist into young adulthood (3,4). Some children have minimal neurodevelopmental impairment, and others have severe impairment (4).
In children with CHD, the prevalence of motor impairments ranges from 20–50%. In toddlers with critical CHD, the prevalence of severe motor impairments reaches 60% (5,6). Overall, the prevalence of severe motor impairments is highest in younger children and does not result in cerebral palsy, which suggests that their motor skills may improve over time (6).
Like children with CHD, children with cerebral palsy have motor impairments in infancy. Cerebral palsy is the leading cause of childhood disabilities in the United States, and it refers to a group of neurological disorders that permanently affect body movement and muscle coordination (7). Unlike children with CHD, children with cerebral palsy have motor impairments that continue, rather than dissipate, over time. Children with cerebral palsy have persistent primitive reflexes, asymmetric posture, and tone differences in their neck or trunk (8). One of the leading causes of cerebral palsy in very preterm (28–32 weeks’ gestation) infants is periventricular leukomalacia (9,10).
We present a case of a former late preterm (36 weeks’ gestation) male with hypoplastic left heart syndrome and a history of hypoxic ischemic encephalopathy and periventricular leukomalacia, first noted on head imaging in infancy. He was subsequently diagnosed with diplegic cerebral palsy during a neurodevelopmental evaluation in a Cardiac Neurodevelopmental Program specialty clinic. We present this case in accordance with the CARE reporting checklist (available at https://tp.amegroups.com/article/view/10.21037/tp-24-57/rc).
Case presentation
We evaluated the chart of a four-year-old boy who presented to a Cardiac Neurodevelopmental Program specialty clinic for neurological concerns in the setting of critical CHD.
Regarding his birth history, a prenatal echocardiogram showed an hypoplastic left heart syndrome variant. There were otherwise no pregnancy complications. The delivery was initially planned as an elective Cesarean section to a 25-year G2 P1002 mother. Prior to the scheduled delivery, the mother was admitted for premature rupture of membranes and preterm labor at 36 3/7 weeks’ gestation.
The infant was in the transverse lie position at the time of delivery. Initial Apgars were 8 at 1 minute and 9 at 5 minutes. He was cyanotic at delivery. He required supplemental oxygen with 25% FiO2 at 2 liters per minute after delivery for mild grunting and transient tachypnea of the newborn. He did not require any other resuscitation.
His birth weight was 2,560 g, and his head circumference was 32 cm. His initial blood gas was a pH of 7.18, CO2 of 49, and pO2 of 23 at the birth hospital. Prostaglandin infusion was initiated 0.05 mcg/kg/min at 45 min of life.
During transport to the cardiovascular intensive care unit, he had a transient episode of apnea that resolved when he was placed on nasal cannula and his prostaglandin infusion was decreased to 0.02 mcg/kg/min. The prostaglandin E (PGE) was further decreased to 0.01 mcg/kg/min. He remained in normal sinus rhythm throughout his course.
He had a postnatal echocardiogram that showed hypoplastic left heart syndrome with mitral and aortic atresia. He was then transported to another hospital for his Norwood palliation.
His birth was complicated by hypoxic ischemic encephalopathy. His brain magnetic resonance imaging (MRI) at 2 days chronologic age was consistent with hypoxic ischemic encephalopathy and periventricular leukomalacia with pre-Wallerian degeneration. Because he had not yet had any surgical intervention at that time, we believe that this hypoxic insult occurred prenatally. Genetic testing revealed unrelated findings. He was discharged from the hospital at three months of age, secondary to surgical palliation of his CHD, specifically the Norwood procedure.
Regarding his surgical history, he was initially palliated with a Norwood Blalock-Taussig-Thomas shunt and tricuspid valvuloplasty when he was 5 days old. During his surgery, he was on cardiopulmonary bypass for 165 minutes and had a cross-clamp time of 106 minutes. He had antegrade cerebral perfusion of 76 min and circulatory arrest of 30 minutes. His length of stay after the Norwood procedure was 97 days.
Prior to his Glenn surgery, he had a rotavirus infection, and he was readmitted when he was 4 months old. He underwent fluid resuscitation and remained in the hospital for his Bi-directional Glenn, which he had 6 days after readmission. During his surgery, he was on cardiopulmonary bypass for 76 minutes. He remained in the hospital for 26 days after his Glenn surgery. He was 5 months old when he was discharged home.
This child was noted to have a right-hand preference at five months of age. Otherwise, his development in infancy was age-appropriate in other domains. By fourteen months, he was receiving occupational, speech, and developmental therapies. He had increased muscle tone in his extremities, and he continued to have a right-sided preference. His brain MRI at sixteen months of age showed bilateral periventricular leukomalacia (Figure 1). By 20 months, he had both axial hypotonia and appendicular hypertonia. At four years of age, he continued to have axial hypotonia and left arm hypertonicity. He also had mild limb-length discrepancies, with his right extremities longer than left. He had gait asymmetry, mild Achilles tendon tightness, and ankle spasticity. At four years of age, another brain MRI showed periventricular leukomalacia with Wallerian degeneration, now more prominent on the right (Figure 2). Imaging findings were consistent with his developmental history and physical examination findings. He was diagnosed with asymmetric spastic diplegia and was referred to physical therapy for appropriate services.
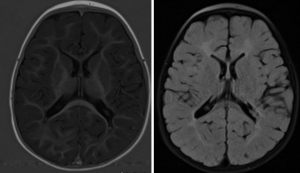
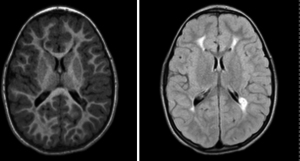
All procedures performed in this study were in accordance with the ethical standards of the institutional and/or national research committee(s) and with the Helsinki Declaration (as revised in 2013). Written informed consent was obtained from the patient’s legal guardian for the publication of this case report and accompanying images. A copy of the written consent is available for review by the editorial office of this journal.
Discussion
This is an interesting case report of a late preterm infant with critical CHD and spastic diplegic cerebral palsy.
A strength of this case is that this child had multidisciplinary evaluations on a regular basis. After he was discharged from the cardiovascular intensive care unit, he was scheduled to have outpatient care through Pediatric Cardiology. He also received outpatient care from several specialty services, which included Pediatric Neurology, Neurodevelopment Disabilities, and Developmental Therapies. Using the 2012 American Heart Association (AHA) Scientific Statement’s risk categories, our center identifies high-risk individuals who meet the criteria for risk category 1; these are individuals who were cyanotic or acyanotic and had open-heart surgery in infancy (11). Infants and toddlers with single ventricle physiology fit this category. We evaluate all children with single ventricle physiology through periodic developmental and medical evaluations every 6 months in the outpatient setting.
A limitation of this study is that knowledge about neurologic and neurodevelopmental differences in children with CHD is still emerging, and not all etiologies have been identified. The etiologies are not well understood and are multifactorial (12). Early research has focused on the intraoperative and perioperative factors and the risk they pose on early neurodevelopment. Research has shown that these factors can affect motor, language, and cognitive outcomes in children with CHD (13-15). Prolonged cardiopulmonary bypass time and length of stay also pose a risk to brain growth (16).
Recently, genetic research has found some evidence that alterations in certain genes may contribute to NDD in children with CHD. One study found 12 NDD genes with damaging de novo variants that alter both cardiac patterning and the connectome (17). Many genetic syndromes such as Trisomy 21, 22q11 deletions, and specific genes such as CHD7 have been commonly associated with both neurodevelopmental and cardiac features (18).
Cardiac pathophysiology can also lead to risk of NDD. Heart defects such as single ventricle physiology decrease the oxygen delivery to the brain, which may contribute to neurodevelopmental impairment (12,19-22).
Prematurity is another factor that can affect neurodevelopment. Children with CHD who are born prematurely are at an increased risk for neurodevelopmental impairments and mortality (23,24). A recent study found that early term birth was associated with greater prevalence of executive dysfunction (25).
There are also socioeconomic factors that can affect neurodevelopment. These include maternal education, health insurance, transportation to the hospital, and living environment. Previous research has shown that children with poor socioeconomic status have poor neurodevelopmental outcomes (26-29). Because it is difficult to identify one primary cause of NDD, we have presented information about several risk factors that may have contributed to this patient’s neurodevelopmental outcomes.
While we hypothesize that brain dysmaturity is a potential cause for this child’s increased susceptibility to injury, we cannot be certain that this is a definitive contributing factor. We have assumed that he had brain insults before or during birth that led to periventricular leukomalacia, but we cannot validate this assertion retrospectively, as our earliest information about brain imaging findings is when he was 2 days old. This was prior to his surgical palliation procedures. He did not have seizure activity in the post-operative period. Despite the remaining questions about what made this child susceptible to periventricular leukomalacia, our findings help to stress the importance of continued developmental monitoring in children with critical CHD.
From 24–40 weeks of gestation, pre oligodendrocytes are developing and are susceptible to hypoxia (9). Hypoxia can result in the pathogenesis of periventricular leukomalacia, which is associated with neurodevelopmental disorders that include cerebral palsy (9). In a study of children with severe periventricular leukomalacia, 63% were born between 24–28 weeks gestation, 22% were born between 28–30 weeks gestation, and 2% were born >37 weeks gestation (9).
Although it is rare for late preterm or full-term infants to have diplegic cerebral palsy in the setting of periventricular leukomalacia, this white matter injury should still be considered. This child may have been more susceptible to this condition, because children with CHD have dysmature brain development, with brain volumes about 4–6 weeks behind their healthy same-age peers (30,31). Thus, his brain dysmaturity may have increased his risk for cerebral palsy in the setting of periventricular leukomalacia (32).
There were several clinical challenges in this case. Like many children with critical CHD, he had motor delays in infancy. Therefore, it was challenging to determine at an early age if his motor delays were a sign of cerebral palsy, or if they were secondary to the neurological sequalae associated with his existing CHD. His neurologic examination continued to evolve through infancy and early childhood. For instance, his combination of both axial hypotonia and appendicular hypertonia made his cerebral palsy diagnosis challenging.
In children with CHD, early motor delays and an evolving neurological examination make early diagnosis of cerebral palsy and other motor disorders difficult. Therefore, it is important to adhere to the 2012 AHA’s recommendation for ongoing neurodevelopmental surveillance for all children with CHD (11). This statement recommended that high-risk children with CHD undergo periodic developmental evaluations for developmental delay from 12 to 24 months, 3 to 5 years, and 11 to 12 years of age. Concerning our single ventricle population, our cardiac neurodevelopmental program evaluates infants and toddlers (from birth to 3 years of age) every 6 months. We also evaluate children for school readiness and provide school related services as they mature. Because of adherence to these recommendations, our program identified the persistence of motor delays in this child, diagnosed him with spastic diplegic cerebral palsy, and enrolled him in additional appropriate therapies to optimize his neurodevelopmental outcomes.
In 2024, a new AHA statement was released, and it recommended that children with CHD be evaluated for developmental delay at key time points: 6 months, 18 months, 3 years, and 5 years (21). These granular milestones may allow us to identify delays at a much earlier age. This systematic approach may allow us to better identify and refer individuals to the appropriate therapies. Going forward, we hope to incorporate this systematic approach within our cardiac neurodevelopmental program.
Conclusions
With this case report, we hope to increase awareness of diagnostic dilemmas related to motor impairments in children with CHD. Through continued developmental surveillance, clinicians can identify NDD and recommend proven therapies that will ultimately improve quality of life and neurodevelopmental outcomes in these formerly critically ill children.
Acknowledgments
The authors would like to acknowledge the guidance provided during preparation of this manuscript made possible by the Center for Childhood Obesity Prevention funded by the National Institute of General Medical Sciences of the National Institutes of Health under Award Number P20GM109096 (Arkansas Children’s Research Institute, PI: Weber). We would also like to acknowledge REDCap resources provided by the National Center for Advancing Translational Sciences of the National Institutes of Health under Award Number ULI RR029884 (University of Arkansas for Medical, PI: James). We would also like to thank the patient and his family for their participation in our case report.
Funding: This work was supported by
Footnote
Reporting Checklist: The authors have completed the CARE reporting checklist. Available at https://tp.amegroups.com/article/view/10.21037/tp-24-57/rc
Peer Review File: Available at https://tp.amegroups.com/article/view/10.21037/tp-24-57/prf
Conflicts of Interest: All authors have completed the ICMJE uniform disclosure form (available at https://tp.amegroups.com/article/view/10.21037/tp-24-57/coif). T.L.J. received salary support from University of Arkansas for Medical Sciences Translational Research Institute: UL1 TR003107, KL2 TR003108. The other authors have no conflicts of interest to declare.
Ethical Statement: The authors are accountable for all aspects of the work in ensuring that questions related to the accuracy or integrity of any part of the work are appropriately investigated and resolved. All procedures performed in this study were in accordance with the ethical standards of the institutional and/or national research committee(s) and with the Helsinki Declaration (as revised in 2013). Written informed consent was obtained from the patient’s legal guardian for the publication of this case report and accompanying images. A copy of the written consent is available for review by the editorial office of this journal.
Open Access Statement: This is an Open Access article distributed in accordance with the Creative Commons Attribution-NonCommercial-NoDerivs 4.0 International License (CC BY-NC-ND 4.0), which permits the non-commercial replication and distribution of the article with the strict proviso that no changes or edits are made and the original work is properly cited (including links to both the formal publication through the relevant DOI and the license). See: https://creativecommons.org/licenses/by-nc-nd/4.0/.
References
- Hasan AA, Abu Lehyah NAA, Al Tarawneh MK, et al. Incidence and types of congenital heart disease at a referral hospital in Jordan: retrospective study from a tertiary center. Front Pediatr 2023;11:1261130. [Crossref] [PubMed]
- Datta S, Cao W, Skillman M, Wu M. Hypoplastic Left Heart Syndrome: Signaling & Molecular Perspectives, and the Road Ahead. Int J Mol Sci 2023;24:15249. [Crossref] [PubMed]
- Knirsch W, De Silvestro A, von Rhein M. Neurodevelopmental and functional outcome in hypoplastic left heart syndrome after Hybrid procedure as stage I. Front Pediatr 2022;10:1099283. [Crossref] [PubMed]
- Liu ZY, Wang QQ, Pang XY, et al. Association of congenital heart disease and neurodevelopmental disorders: an observational and Mendelian randomization study. Ital J Pediatr 2024;50:63. [Crossref] [PubMed]
- Bolduc ME, Dionne E, Gagnon I, et al. Motor Impairment in Children With Congenital Heart Defects: A Systematic Review. Pediatrics 2020;146:e20200083. [Crossref] [PubMed]
- Kaeslin R, Latal B, Mitteregger E. A systematic review of early motor interventions for infants with congenital heart disease and open-heart surgery. Syst Rev 2023;12:149. [Crossref] [PubMed]
- Patel DR, Neelakantan M, Pandher K, et al. Cerebral palsy in children: a clinical overview. Transl Pediatr 2020;9:S125-35. [Crossref] [PubMed]
- Khanna S, Arunmozhi R, Goyal C. Neurodevelopmental Treatment in Children With Cerebral Palsy: A Review of the Literature. Cureus 2023;15:e50389. [Crossref] [PubMed]
- Ahya KP, Suryawanshi P. Neonatal periventricular leukomalacia: current perspectives. Res Reports Neonatol 2017; [Crossref]
- Song J, Yue Y, Sun H, et al. Clinical characteristics and long-term neurodevelopmental outcomes of leukomalacia in preterm infants and term infants: a cohort study. J Neurodev Disord 2023;15:24. [Crossref] [PubMed]
- Marino BS, Lipkin PH, Newburger JW, et al. Neurodevelopmental outcomes in children with congenital heart disease: evaluation and management: a scientific statement from the American Heart Association. Circulation 2012;126:1143-72. [Crossref] [PubMed]
- Verrall CE, Blue GM, Loughran-Fowlds A, et al. 'Big issues' in neurodevelopment for children and adults with congenital heart disease. Open Heart 2019;6:e000998. [Crossref] [PubMed]
- Neukomm A, Claessens NHP, Bonthrone AF, et al. Perioperative Brain Injury in Relation to Early Neurodevelopment Among Children with Severe Congenital Heart Disease: Results from a European Collaboration. J Pediatr 2024;266:113838. [Crossref] [PubMed]
- Allen KY, Marino BS. Developmental Delay in Children with Congenital Heart Disease. In: Abdulla R, Berger S, Backer C, et al., editors. Pediatric Cardiology. Cham: Springer International Publishing; 2023. doi:
10.1007/978-3-030-42937-9_86-1 . - Cañizo-Vazquez D, Hadley S, Leonhardt M, et al. Early post-operative neurodevelopment and visual assessment in neonates with congenital heart disease undergoing cardiac surgery. J Perinatol 2023;43:856-63. [Crossref] [PubMed]
- Cromb D, Bonthrone AF, Maggioni A, et al. Individual Assessment of Perioperative Brain Growth Trajectories in Infants With Congenital Heart Disease: Correlation With Clinical and Surgical Risk Factors. J Am Heart Assoc 2023;12:e028565. [Crossref] [PubMed]
- Ji W, Ferdman D, Copel J, et al. De novo damaging variants associated with congenital heart diseases contribute to the connectome. Sci Rep 2020;10:7046. [Crossref] [PubMed]
- Patt E, Singhania A, Roberts AE, et al. The Genetics of Neurodevelopment in Congenital Heart Disease. Can J Cardiol 2023;39:97-114. [Crossref] [PubMed]
- Corno AF, Findley TO, Salazar JD. Narrative review of single ventricle: where are we after 40 years? Transl Pediatr 2023;12:221-44. [Crossref] [PubMed]
- Trivedi A, Browning Carmo K, Jatana V, et al. Growth and risk of adverse neuro-developmental outcome in infants with congenital heart disease: A systematic review. Acta Paediatr 2023;112:53-62. [Crossref] [PubMed]
- Sood E, Newburger JW, Anixt JS, et al. Neurodevelopmental Outcomes for Individuals With Congenital Heart Disease: Updates in Neuroprotection, Risk-Stratification, Evaluation, and Management: A Scientific Statement From the American Heart Association. Circulation 2024;149:e997-e1022. [Crossref] [PubMed]
- Kordopati-Zilou K, Sergentanis T, Pervanidou P, et al. Dextro-Transposition of Great Arteries and Neurodevelopmental Outcomes: A Review of the Literature. Children (Basel) 2022;9:502. [Crossref] [PubMed]
- Reddy RK, McVadon DH, Zyblewski SC, et al. Prematurity and Congenital Heart Disease: A Contemporary Review. Neoreviews 2022;23:e472-85. [Crossref] [PubMed]
- Katz JA, Levy PT, Butler SC, et al. Preterm congenital heart disease and neurodevelopment: the importance of looking beyond the initial hospitalization. J Perinatol 2023;43:958-62. [Crossref] [PubMed]
- Calderon J, Stopp C, Wypij D, et al. Early-Term Birth in Single-Ventricle Congenital Heart Disease After the Fontan Procedure: Neurodevelopmental and Psychiatric Outcomes. J Pediatr 2016;179:96-103. [Crossref] [PubMed]
- Robinson J, Sahai S, Pennacchio C, et al. Effects of Sociodemographic Factors on Access to and Outcomes in Congenital Heart Disease in the United States. J Cardiovasc Dev Dis 2024;11:67. [Crossref] [PubMed]
- Neukomm A, Ehrler M, Feldmann M, et al. Perioperative Course and Socioeconomic Status Predict Long-Term Neurodevelopment Better Than Perioperative Conventional Neuroimaging in Children with Congenital Heart Disease. J Pediatr 2022;251:140-148.e3. [Crossref] [PubMed]
- Mayourian J, Brown E, Javalkar K, et al. Insight into the Role of the Child Opportunity Index on Surgical Outcomes in Congenital Heart Disease. J Pediatr 2023;259:113464. [Crossref] [PubMed]
- Nashed LM, O'Neil J. The impact of socioeconomic status and race on the outcomes of congenital heart disease. Curr Opin Cardiol 2022;37:86-90. [Crossref] [PubMed]
- Leonetti C, Back SA, Gallo V, et al. Cortical Dysmaturation in Congenital Heart Disease. Trends Neurosci 2019;42:192-204. [Crossref] [PubMed]
- Volpe JJ. Fetal origin of brain dysmaturation in congenital heart disease - Challenges and opportunities for interventions. J Neonatal Perinatal Med 2022;15:489-94. [Crossref] [PubMed]
- Ryan MA, Murray DM, Dempsey EM, et al. Neurodevelopmental outcome of low-risk moderate to late preterm infants at 18 months. Front Pediatr 2023;11:1256872. [Crossref] [PubMed]