Soluble transferrin receptor is associated with red blood cell transfusion in infant cardiac surgery
Highlight box
Key findings
• Preoperative soluble transferrin receptor (sTfR) concentrations were significantly associated with high-volume red blood cell (RBC) transfusions.
What is known and what is new?
• The sTfR, an inflammation-independent marker of iron deficiency, has emerged as a potential marker for evaluating iron status.
• The relationship between sTfR level and the need for a high-volume of RBC transfusion in pediatric cardiac surgery patients has not been explored, our study may provide new evidence on this issue.
What is the implication, and what should change now?
• These findings suggest that the sTfR may be a useful biomarker for predicting the need for higher-volume RBC transfusions in this population.
Introduction
Perioperative red blood cell (RBC) transfusion is commonly needed in pediatric cardiac surgery. It is associated with an increased risk of postoperative complications, including longer pediatric intensive care unit (PICU) length of stay, infection, and mortality (1-4). Infant cardiac surgery often requires RBC transfusion due to iron deficiency (ID), hemodilution, and coagulation-related challenges (5-7). Despite the implementation of numerous blood conservation strategies in recent years, the risk of perioperative blood transfusion remains high for pediatric cardiac surgery patients.
Low-weight infants are at high risk of ID due to rapid growth and development (6). Previous studies have shown an association between ID and an increased risk of perioperative RBC transfusion, and iron-related indicators are independent predictors of perioperative RBC transfusion (8-10). Additionally, due to the disease itself, preoperative medical interventions, and exposure to a new environment, infants with congenital heart disease (CHD) are more prone to being in an inflammatory state before surgery. However, malnutrition and inflammatory conditions affect the serum concentrations of traditional iron biomarkers, such as ferritin (11-13). Hence, the accuracy of these traditional iron-related indicators for infants might be questionable. The soluble transferrin receptor (sTfR) level is a useful indicator of iron requirements for tissue and erythropoiesis and is not affected by inflammation (14,15). It is considered a stronger predictor of ID than other biomarkers (16).
The association between sTfR level and the need for perioperative RBC transfusion has not been fully elucidated in cardiac surgery, especially in low-weight infants (≤10 kg), who are more likely to suffer from malnutrition and ID (17). Therefore, the purpose of this study was to explore the distribution of iron-related indicators in low-weight infants undergoing cardiac surgery and the relationship between sTfR level and the need for higher-volume perioperative RBC transfusions. We present this article in accordance with the STROBE reporting checklist (available at https://tp.amegroups.com/article/view/10.21037/tp-24-132/rc).
Methods
Study design
This was a secondary analysis of a database used for a prospective observational study of infants who underwent elective cardiac surgery with cardiopulmonary bypass (CPB) (18). The study was conducted in accordance with the Declaration of Helsinki (as revised in 2013) and was approved by the Ethics Committee of the Chinese Academy of Medical Sciences Fuwai Hospital (No. 2021-1483). Informed consent was obtained from all the patients’ parents or legal guardians. The aim of the initial study was to investigate the relationship between oxygen delivery during CPB and the incidence of postoperative acute kidney injury (AKI). Low-weight infants (≤10 kg) who underwent cardiac surgery with CPB for 1–3 hours were selected for the study. A total of 413 infants were included in the original study from August 2021 to July 2022, and 177 patients were excluded from this analysis. Patients (I) with missing preoperative sTfR data, (II) with extreme sTfR values (sTfR >100 nmol/L), and (III) receiving RBC transfusion 1 week before the operation were excluded.
Data sources and definitions
Patient data were manually extracted from the electronic health records. Cardiac surgical procedures were categorized according to the Society of Thoracic Surgeons-European Association for Cardiothoracic Surgery (STS-EACTS) categories (19). The baseline laboratory indices were collected within 1 week before the operation. Patient demographic characteristics, laboratory biomarkers, intraoperative information, postoperative data, and clinical characteristics were collected for analysis. The baseline laboratory biomarkers included routine blood, biochemical, and iron-related marker data. Intraoperative information included CPB duration, aortic cross-clamping (ACC) duration, vasoactive-inotropic score (VIS), and plasma free hemoglobin (pFHb) level. Postoperative data included duration of mechanical ventilation (MV), reoperation, PICU length of stay (days), length of hospital stay, AKI, and in-hospital mortality. The clinical characteristics included STS-EACTS categories, cyanotic heart disease, and perioperative RBC transfusion.
Perioperative RBC transfusion was defined as the receipt of both intraoperative and postoperative RBC transfusion. Patients who underwent RBC transfusion were further categorized into two groups: those receiving ≤2 units (lower volume) and those receiving >2 units (higher volume) during the perioperative period. We determined postoperative AKI based on the Kidney Disease Improving Global Outcomes (KDIGO) criteria (20). Anemia is defined as a hemoglobin (Hb) concentration less than 110 g/L (12). VIS was calculated as previously described (21). A pFHb >500 mg/L was considered severe hemolysis after CPB (22). In our study, a length of stay >30 days was defined as prolonged hospitalization, and a duration of MV >100 hours was defined as extended MV. Postoperative bleeding was determined if there was ≥16 mL/kg of blood in chest tube drainage fluid per day (23).
Clinical practice
Surgical procedures, general anesthesia, and CPB were performed according to the standard protocol of Fuwai Hospital. The CPB circuit consists of a roller pump (Stockert S5, Sorin, Arvada, CO, USA), a hollow fiber membrane oxygenator (Fx05, Terumo, Tokyo, Japan), an infant blood tubing pack (Tianjin Plastic Research Institution, Tianjin, China) and a blood filter (MaquetBC20, Hirrlingen, Germany). Compounded electrolyte solutions, gelofusine, and human ALB were precharged in the CPB circuit. For patients with a body weight <8 kg and a preoperative Hb concentration <120 g/L, allogeneic RBCs were supplemented in the priming fluid. CPB was established after systemic heparinization. Modified St. Thomas solution or histidine-tryptophan-ketoglutarate solution was selected for myocardial protection according to the operation time and complexity of the procedure. During surgery, all infants underwent conventional ultrafiltration during CPB and modified ultrafiltration after CPB weaning, with a target Hb >100 g/L. During the operation, when the Hb was <70 g/L during CPB or the Hb was <90 g/L after CPB weaning, an RBC transfusion was needed. All patients were admitted to the PICU immediately after the operation. After infants were transferred to the PICU, the threshold for RBC transfusion was 80 g/L; for infants with end-organ ischemia or low cardiac output, the transfusion threshold was 90 g/L.
Statistical analysis
The Shapiro-Wilk test was used to assess the normality of the distribution of the variables. Continuous variables were presented as the mean ± interquartile range (IQR) and were analyzed using the Mann-Whitney U test or Student’s t-test. Categorical variables were expressed as numbers and percentages, and the chi-squared test or Fisher’s exact test was used for comparisons. Missing data were handled using multiple imputation techniques.
To assess the predictive performance of circulating iron biomarkers for perioperative RBC transfusion, receiver operating characteristic (ROC) curve analysis was performed, and the area under the curve (AUC) was estimated. Sensitivity and specificity were calculated to identify the most accurate cutoff values for each biomarker.
Multivariate logistic regression models were constructed to explore the association between sTfR level and the need for perioperative RBC transfusion. Model 1 was a crude model without adjustment for potential confounders. Model 2 included adjustments for age and sex as inherent demographic factors. Model 3 was further adjusted for weight, cyanotic heart disease, anemia, platelet, hypersensitive C-reactive protein (hs-CRP), direct bilirubin, Hb, VIS, STS-EACTS categories, CPB duration, ACC duration, and pFHb. We embedded categorical and continuous models into the multivariable logistic regression model. In the categorical analysis, sTfRs were dichotomized using a previously established cutoff value (≥17.53 nmol/L).
Sensitivity analyses were performed to check the robustness of our results. First, to minimize the potential confounding influence of excessive bleeding, we excluded infants with postoperative bleeding. Second, we excluded patients who had prolonged hospitalization, extended ventilation, or who required secondary intensive care unit (ICU) admission. Finally, we further excluded infants with severe hemolysis after CPB. Additionally, Spearman correlation analysis was used to explore the correlation between preoperative sTfR and baseline laboratory values, which was also used to explore the correlation between preoperative sTfR and clinical outcomes.
For both the continuous and categorical variable models, the estimated odds ratios (ORs) and 95% confidence intervals (CIs) were reported. A P value <0.05 was considered to indicate statistical significance. All the statistical analyses were performed in R version 4.3.1 (The R Foundation, Vienna, Austria) and IBM SPSS Statistics 26.
Results
A total of 413 infants were included in the original study between August 2021 and July 2022. One hundred and seventy-seven patients were excluded from this analysis: 169 patients with missing sTfR data, 5 patients with extreme sTfR values, and 3 patients who received preoperative RBC transfusions (Figure 1).
The baseline demographic characteristics, preoperative laboratory biomarkers, intraoperative information, postoperative data, and clinical characteristics are summarized in Table 1. The median age was 195.00 days (IQR, 130.75–293.75 days), and 48.7% of the patients were female. All patients underwent CPB for a duration of 85.00 minutes (IQR, 65.50–111.00 minutes). The baseline concentrations of sTfR, serum iron (SI), serum ferritin (SF), transferrin saturation (TAST), and total iron binding capacity (TIBC) were 14.59 nmol/L (IQR, 12.46–18.90 nmol/L), 10.62 µmol/L (IQR, 7.62–13.25 µmol/L), 38.08 µg/L (IQR, 19.60–84.32 µg/L), 18.05% (IQR, 13.18–24.92%), and 56.42 µmol/L (IQR, 49.42–64.29 µmol/L), respectively. In the total cohort, 226 (95.8%) infants were transfused with at least half a unit of RBCs, and 29 (12.3%) infants required more than 2 units of RBCs during the perioperative period. Figure 2 shows the iron biomarker distribution according to sex, and there was no difference in the distribution of iron markers between sexes (all P values >0.05).
Table 1
Characteristics | Overall (n=236) |
---|---|
Age (days) | 195.00 (130.75, 293.75) |
Sex (female) | 115 (48.7) |
Weight (kg) | 7.00 (5.90, 8.50) |
BSA (m2) | 0.34 (0.31, 0.40) |
Anemia | 82 (34.7) |
Cyanotic heart disease | 57 (24.2) |
STS-EACTS categories | |
1 | 139 (58.9) |
2 | 82 (34.7) |
3 | 8 (3.4) |
4 | 7 (3.0) |
Preoperative information | |
sTfR (nmol/L) | 14.59 (12.46, 18.90) |
SI (µmol/L) | 10.62 (7.62, 13.25) |
SF (µg/L) | 38.08 (19.60, 84.32) |
TAST (g/L) | 18.05 (13.18, 24.92) |
TIBC (µmol/L) | 56.42 (49.42, 64.29) |
Hb (g/L) | 113.00 (106.00,123.00) |
HCT (L/L) | 34.45 (32.00, 37.10) |
hs-CRP (mg/L) | 0.20 (0.11, 0.45) |
ALT (IU/L) | 24.00 (18.00, 35.00) |
AST (IU/L) | 52.00 (44.00, 62.00) |
Albumin (g/L) | 41.80 (40.10, 43.40) |
Creatinine (µmol/L) | 29.68 (25.95, 33.62) |
Blood urea nitrogen (mmol/L) | 3.18 (2.27, 4.61) |
Total bilirubin (µmol/L) | 5.87 (3.91, 8.48) |
Direct bilirubin (µmol/L) | 1.33 (0.92, 2.01) |
Platelet count (×109/L) | 325.50 (279.75,381.25) |
Intraoperative information | |
CPB time (min) | 85.00 (65.50, 111.00) |
ACC duration (min) | 55.00 (44.00,80.00) |
Intraoperative VIS | 9.00 (9.00, 11.00) |
pFHb after CPB (mg/L) | 4.00 (2.00, 6.00) |
Postoperative information | |
ICU time (days) | 3.00 (2.00, 5.00) |
Ventilation time (hours) | 15.00 (6.00, 25.00) |
Length of hospital stay (days) | 13.00 (10.00, 17.00) |
Re-operation | 4 (1.7) |
AKI | 54 (22.9) |
RBC transfusion | 226 (95.8) |
RBC transfusion more than 2 units | 29 (12.3) |
ECMO | 3 (1.3) |
In-hospital mortality | 1 (0.4) |
Continuous data are presented as median (IQR) and categorical data as n (%). BSA, body surface area; STS-EACTS, Society of Thoracic Surgeons-European Association for Cardiothoracic Surgery; sTfR, soluble transferrin receptor; SI, serum iron; SF, serum ferritin; TAST, transferrin saturation; TIBC, total iron binding capacity; Hb, hemoglobin; HCT, hematocrit; hs-CRP, hypersensitive C-reactive protein; ALT, alanine aminotransferase; AST, aspartate aminotransferase; CPB, cardiopulmonary bypass; ACC, aortic cross-clamping; VIS, vasoactive-inotropic score; pFHb, plasma free hemoglobin; ICU, intensive care unit; AKI, acute kidney injury; RBC, red blood cell; ECMO, extracorporeal membrane oxygenation; IQR, interquartile range.
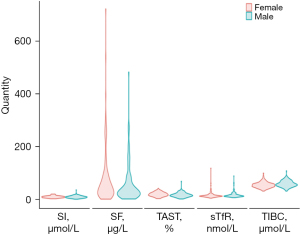
As shown in Table 2, patients requiring higher volume transfusions were younger, had a higher incidence of cyanotic heart disease, and had a greater VIS than patients receiving ≤2 units of RBCs. Additionally, significant differences were observed in weight, body surface area, STS-EACTS categories, preoperative iron biomarkers (including sTfR, SI, and TAST), hs-CRP, serum creatinine, direct bilirubin, CPB time, ACC duration, VIS, pFHb after CPB, PICU duration, MV duration, and length of hospital stay (all P values <0.05).
Table 2
Characteristics | Lower volume RBC transfusion (n=207) | Higher volume RBC transfusion (n=29) | P value |
---|---|---|---|
Age (days) | 197.00 (131.00, 314.00) | 162.00 (130.00, 208.00) | 0.043 |
Sex (female) | 104 (50.2) | 11 (37.9) | 0.30 |
Weight (kg) | 7.10 (6.00, 8.75) | 6.60 (5.10, 7.10) | 0.005 |
BSA (m2) | 0.35 (0.31, 0.40) | 0.33 (0.30, 0.33) | 0.003 |
Anemia | 73 (35.3) | 9 (31.0) | 0.81 |
Cyanotic heart disease | 43 (20.8) | 14 (48.3) | 0.003 |
STS-EACTS categories | 0.03 | ||
1 | 129 (62.3) | 10 (34.5) | |
2 | 67 (32.4) | 15 (51.7) | |
3 | 6 (2.9) | 2 (6.9) | |
4 | 5 (2.4) | 2 (6.9) | |
sTfR (nmol/L) | 14.34 (12.34, 18.08) | 18.15 (13.09, 25.96) | 0.01 |
SI (µmol/L) | 10.76 (7.80, 13.88) | 9.14 (6.16, 10.95) | 0.02 |
SF (µg/L) | 37.53 (20.13, 70.23) | 48.84 (18.97, 116.92) | 0.52 |
TAST (g/L) | 18.55 (13.67, 25.83) | 16.44 (12.00, 19.74) | 0.02 |
TIBC (µmol/L) | 56.30 (49.40, 63.51) | 59.58 (53.29, 70.21) | 0.27 |
Hb (g/L) | 113.00 (105.50,123.00) | 114.00 (107.00, 129.00) | 0.32 |
HCT (L/L) | 34.20 (32.00, 36.90) | 35.10 (33.50, 40.10) | 0.08 |
hs-CRP (mg/L) | 0.20 (0.11, 0.36) | 0.22 (0.11, 0.53) | 0.35 |
ALT (IU/L) | 24.00 (18.00, 35.00) | 26.00 (16.00, 39.00) | 0.72 |
AST (IU/L) | 52.00 (44.00, 61.00) | 52.00 (46.00, 73.00) | 0.057 |
Albumin (g/L) | 41.80 (40.15, 43.50) | 41.40 (39.30, 42.60) | 0.31 |
Creatinine (µmol/L) | 29.57 (25.70, 32.78) | 31.91 (27.72, 36.40) | 0.06 |
Blood urea nitrogen (mmol/L) | 3.27 (2.28, 4.64) | 2.93 (2.15, 3.73) | 0.48 |
Total bilirubin (µmol/L) | 5.69 (3.80, 8.25) | 7.49 (4.76, 12.26) | 0.049 |
Direct bilirubin (µmol/L) | 1.29 (0.88, 1.97) | 1.80 (1.33, 3.14) | 0.004 |
Platelet count (×109/L) | 332.00 (281.00, 381.50) | 309.00 (280.00, 380.00) | 0.33 |
CPB time (min) | 82.00 (64.00, 103.50) | 118.00 (96.00, 135.00) | <0.001 |
ACC duration (min) | 54.00 (43.00, 76.00) | 80.00 (64.00, 95.00) | <0.001 |
Intraoperative VIS | 9.00 (9.00, 11.00) | 11.00 (9.00, 14.00) | 0.001 |
pFHb after CPB (mg/L) | 4.00 (2.00, 6.00) | 6.00 (5.00, 11.00) | <0.001 |
ICU time (days) | 3.00 (2.00, 5.00) | 7.00 (4.00, 14.00) | <0.001 |
Ventilation time (hours) | 10.00 (6.00, 24.00) | 26.00 (22.00, 50.00) | <0.00 |
Length of hospital stay (days) | 13.00 (10.00, 16.00) | 20.00 (16.00, 30.00) | <0.001 |
Re-operation | 3 (1.4) | 1 (3.4) | 0.99 |
AKI | 46 (22.2) | 8 (27.6) | 0.68 |
ECMO | 2 (1.0) | 1 (3.4) | 0.82 |
In-hospital mortality | 0 (0.0) | 1 (3.4) | 0.25 |
Continuous data are presented as median (IQR) and categorical data as n (%). RBC, red blood cell; BSA, body surface area; STS-EACTS, Society of Thoracic Surgeons-European Association for Cardiothoracic Surgery; sTfR, soluble transferrin receptor; SI, serum iron; SF, serum ferritin; TAST, transferrin saturation; TIBC, total iron binding capacity; Hb, hemoglobin; HCT, hematocrit; hs-CRP, hypersensitive C-reactive protein; ALT, alanine aminotransferase; AST, aspartate aminotransferase; CPB, cardiopulmonary bypass; ACC, aortic cross-clamping; VIS, vasoactive-inotropic score; pFHb, plasma free hemoglobin; ICU, intensive care unit; AKI, acute kidney injury; ECMO, extracorporeal membrane oxygenation; IQR, interquartile range.
Correlation of sTfR levels with continuous covariates
The correlations between sTfR and preoperative continuous covariates are shown in Table S1. The sTfR was negatively correlated with the SI, SF, and TSAT (Spearman’s rank correlation coefficient =−0.66, −0.42, and −0.75, respectively; all P values <0.001). The sTfR was positively correlated with the TIBC (Spearman’s rank correlation coefficient =0.410; P<0.001). Moreover, there was no significant difference in the correlation between the inflammatory marker hs-CRP and sTfR (P=0.11).
Iron biomarkers for predicting perioperative high-volume RBC transfusion
Table S2 presents the accuracy of various iron biomarkers for predicting perioperative RBC transfusion. Among these biomarkers, the SF demonstrated the lowest predictive accuracy (AUC =0.537; optimal cutoff value ≤81.50 µg/L; sensitivity =44.8%; specificity =76.8%). Conversely, the sTfR exhibited the highest accuracy in predicting the need for high-volume RBC transfusion (AUC =0.643; optimal cutoff value ≥17.53 nmol/L; sensitivity =58.6%; specificity =73.9%).
Association of serum sTfR levels with perioperative increased-volume RBC transfusion
Table 3 shows the association between the serum sTfR level and the RBC transfusion rate in low-weight pediatric cardiac surgery patients. A high sTfR, both when dichotomized at ≥17.53 vs. <17.53 nmol/L and when analyzed as a continuous variable, was associated with a higher volume RBC transfusion. According to the categorical model of model 3, compared with patients with lower serum sTfR levels (<17.53 nmol/L), patients with higher sTfR levels (≥17.53 nmol/L) had a 4.014-fold higher risk (adjusted OR, 4.013; 95% CI: 1.815–9.140; P<0.001) of receiving high-volume RBC transfusions in the total population. Similarly, in the continuous model, higher serum sTfR levels were significantly associated with an increased risk of needing more RBC transfusions [adjusted OR per 1 nmol/L increase of 1.07 (1.027–1.126); P=0.005].
Table 3
Outcomes | Categorical models | Continuous models | |||
---|---|---|---|---|---|
OR (95% CI) | P value | OR (95% CI) | P value | ||
Model 1 | 4.013 (1.815–9.140) | <0.001 | 1.034 (1.008–1.063) | 0.009 | |
Model 2 | 4.388 (1.922–10.389) | <0.001 | 1.043 (1.015–1.076) | 0.005 | |
Model 3 | 10.730 (3.442–42.061) | <0.001 | 1.070 (1.027–1.126) | 0.006 |
Model 1 did not adjust any potential confounders; model 2 adjusted for age, sex; model 3 further adjusted for weight, cyanosis, anemia, platelet, hs-CRP, direct bilirubin, Hb, STS-EACTS categories, CPB time, ACC duration, intraoperative VIS, pFHb. sTfR, soluble transferrin receptor; RBC, red blood cell; OR, odds ratio; CI, confidence interval; hs-CRP, hypersensitive C-reactive protein; Hb, hemoglobin; STS-EACTS, Society of Thoracic Surgeons-European Association for Cardiothoracic Surgery; CPB, cardiopulmonary bypass; ACC, aortic cross-clamping; VIS, vasoactive-inotropic score; pFHb, plasma free hemoglobin.
Serum sTfR levels and adverse outcomes
Table S3 presents the relationships between serum sTfR level and various adverse outcomes, including duration of MV, length of stay in the PICU, length of hospital stay, AKI incidence, and in-hospital mortality, in low-weight patients who underwent pediatric cardiac surgery. Notably, no significant associations were observed between sTfR levels and adverse outcomes (all P values >0.05).
Sensitivity analyses
To assess the robustness of our findings, we conducted sensitivity analyses by excluding patients with potential confounding factors. As shown in Table S4, we excluded patients with postoperative bleeding. The relationship between sTfR level and high-volume RBC transfusion remained statistically significant across all three models (continuous models and categorical models; P<0.05). Similarly, we excluded patients who had prolonged hospital stays, who had extended MV, or who had secondary ICU admissions. Finally, we excluded infants with severe hemolysis after CPB. These results were consistent with those of the main analysis (P<0.05).
Discussion
In our study of low-weight infants who underwent cardiac surgery, 12.3% required more than 2 units of RBCs for perioperative transfusions. We investigated the distribution of iron-related markers in infants with CHD according to sex and investigated the relationship between sTfR level and high-volume RBC transfusions. Analysis of the entire cohort indicated a significant association between preoperatively elevated sTfR level and the need for a high-volume RBC transfusion. The results of all sensitivity analyses were consistent with the main results. Furthermore, the predictive ability of sTfR level for the need for perioperative transfusions was stronger than other iron indicators.
Circulating sTfRs have emerged as a relatively novel biomarker for assessing iron status, reflecting the cellular iron uptake pathway (11). sTfR levels primarily depend on erythropoietic activity and iron stores and are less susceptible to the influence of inflammation than other iron markers (15,24). However, the distribution of iron biomarkers in infants undergoing cardiac surgery is not well characterized, particularly in low-weight infants. Therefore, we present the distribution of iron biomarkers in this specific population. Although some studies suggest potential sex differences in iron biomarkers during infancy (25,26), we did not observe any significant sex differences in our cohort. Additionally, except for iron-related indicators, no correlation was found between other variables and sTfR, among which the correlation between TAST and sTfR was the strongest. In institutions that cannot monitor sTfR, the TAST may play a role in predicting the need for perioperative RBC transfusion. Consistent with the findings of previous studies, there was no correlation between inflammatory markers and sTfR in our study, which further proves that sTfR is not affected by inflammatory markers.
While bone marrow iron estimation remains the gold standard for assessing iron status, its invasiveness limits its routine clinical use (27). Therefore, SI markers, such as SF, are commonly employed in clinical settings to diagnose ID (12). However, the use of these indicators in the inflammatory state is still limited, especially in pediatric patients with CHD. As mentioned earlier, sTfR is less susceptible to inflammation than are other iron markers (24), which led to investigations of its efficacy in identifying ID in patients with inflammatory conditions such as inflammatory bowel disease and heart failure (16,28-30). Compared with other iron indicators, the sTfR has been shown to be the most reliable indicator for identifying ID (16). While previous research has linked high sTfR levels to adverse outcomes, primarily in adults (16,28,31,32), few studies have explored the relationship between sTfR levels and the need for RBC transfusion in low-weight infants undergoing cardiac surgery. In our study, we compared the accuracy of various iron markers in predicting the need for a high-volume RBC transfusion, although the AUC value was relatively low, the predictive power of sTfR levels was higher than other indicators.
We adjusted for relevant covariates in both the continuous and categorical variable models. Notably, a consistent association between sTfR level and the need for a high-volume transfusion was observed across all three models. To mitigate the influence of confounders, infants with excessive postoperative bleeding or severe hemolysis after CPB were excluded. Additionally, patients who had prolonged hospital stays, prolonged MV, or who required secondary ICU admission were excluded. The sensitivity analysis yielded conclusions consistent with those of the initial analysis. However, the sensitivity analysis of categorical variable models revealed a wider CI, potentially due to the limited sample size. Previous studies have shown that a high sTfR level is associated with adverse outcomes (16,28,31,33). However, in our study, no correlation was found between high sTfR levels and adverse outcomes, including ICU stay, MV time, length of hospital stay, in-hospital mortality, or AKI incidence.
Iron supplementation is an effective means to reduce the need for perioperative RBC transfusion (9,34,35), sTfR can be more accurately identified in patients with heart failure and inflammatory patients who need iron supplementation. In addition, sTfR levels can reflect erythropoiesis activity, so some scholars believe that it can also be used as a marker for monitoring the response to iron supplementation (15). Although previous studies have reported a normal reference range (36), standardization remains challenging due to differences in units, reference ranges, age, and sex (26,36,37). In the future, standardization of sTfR assays is crucial for establishing a uniform intervention threshold.
There are some limitations in this study. First, the small sample size led to wider CIs for ORs in the categorical variable model. Nevertheless, given the relative rarity of perioperative iron testing and pediatric cardiac surgery, our study included as many patients as possible from a large pediatric heart center. Second, considering that the coagulation status during cardiac surgery is dynamic, we did not evaluate the coagulation status of the infants intraoperatively. The coagulation status of infants has an impact on the need for perioperative RBC transfusion. Therefore, coagulation disorders might be a potential confounder even if infants with more postoperative bleeding were excluded from the sensitivity analysis. Third, from 2021 to 2022, sTfR monitoring was not fully implemented in our center. Consequently, many low-weight infants in our original study were not screened for sTfR, which may have introduced bias into the results. Over the past 2 years, in view of the important role of this marker in the diagnosis of ID anemia, our center now routinely monitors sTfRs. Lastly, the role of sTfR in monitoring iron supplementation and evaluating its effectiveness has been underexplored in infant cardiac surgery. Future prospective studies with larger sample sizes are needed to further investigate the potential of sTfR in this context.
Conclusions
In conclusion, we investigated the distribution of iron-related indicators in infants with CHD. More importantly, our results showed that preoperative sTfR concentrations were significantly associated with high-volume RBC transfusions. These findings suggest that the sTfR may be a useful biomarker for predicting the need for higher-volume RBC transfusions in this population. However, further prospective studies and randomized controlled trials are warranted to confirm this association and to establish its clinical utility.
Acknowledgments
We thank all participants for their contribution to this study.
Funding: This study was supported by
Footnote
Reporting Checklist: The authors have completed the STROBE reporting checklist. Available at https://tp.amegroups.com/article/view/10.21037/tp-24-132/rc
Data Sharing Statement: Available at https://tp.amegroups.com/article/view/10.21037/tp-24-132/dss
Peer Review File: Available at https://tp.amegroups.com/article/view/10.21037/tp-24-132/prf
Conflicts of Interest: All authors have completed the ICMJE uniform disclosure form (available at https://tp.amegroups.com/article/view/10.21037/tp-24-132/coif). All authors report that this work was supported by the National High-Level Hospital Clinical Research Funding (No. 2022-GSP-GG-22). The authors have no other conflicts of interest to declare.
Ethical Statement: The authors are accountable for all aspects of the work in ensuring that questions related to the accuracy or integrity of any part of the work are appropriately investigated and resolved. The study was conducted in accordance with the Declaration of Helsinki (as revised in 2013). The study was approved by the Ethics Committee of the Chinese Academy of Medical Sciences Fuwai Hospital (No. 2021-1483) and informed consent was obtained from all the patients’ parents or legal guardians.
Open Access Statement: This is an Open Access article distributed in accordance with the Creative Commons Attribution-NonCommercial-NoDerivs 4.0 International License (CC BY-NC-ND 4.0), which permits the non-commercial replication and distribution of the article with the strict proviso that no changes or edits are made and the original work is properly cited (including links to both the formal publication through the relevant DOI and the license). See: https://creativecommons.org/licenses/by-nc-nd/4.0/.
References
- Kneyber MC, Grotenhuis F, Berger RF, et al. Transfusion of leukocyte-depleted RBCs is independently associated with increased morbidity after pediatric cardiac surgery. Pediatr Crit Care Med 2013;14:298-305. [Crossref] [PubMed]
- Mazine A, Rached-D'Astous S, Ducruet T, et al. Blood Transfusions After Pediatric Cardiac Operations: A North American Multicenter Prospective Study. Ann Thorac Surg 2015;100:671-7. [Crossref] [PubMed]
- Busack C, Rana MS, Beidas Y, et al. Intraoperative blood product transfusion in pediatric cardiac surgery patients: A retrospective review of adverse outcomes. Paediatr Anaesth 2023;33:387-97. [Crossref] [PubMed]
- DiNardo JA. Blood transfusions might be bad for you; that is unless you are bleeding. Anesth Analg 2013;116:1201-3. [Crossref] [PubMed]
- Faraoni D, DiNardo JA. Red Blood Cell Transfusion and Adverse Outcomes in Pediatric Cardiac Surgery Patients: Where Does the Blame Lie? Anesth Analg 2021;133:1074-6. [Crossref] [PubMed]
- Berglund SK, Domellöf M. Iron deficiency in infancy: current insights. Curr Opin Clin Nutr Metab Care 2021;24:240-5. [Crossref] [PubMed]
- Cholette JM, Faraoni D, Goobie SM, et al. Patient Blood Management in Pediatric Cardiac Surgery: A Review. Anesth Analg 2018;127:1002-16. [Crossref] [PubMed]
- Gao P, Wang X, Zhang P, et al. Preoperative Iron Deficiency Is Associated With Increased Blood Transfusion in Infants Undergoing Cardiac Surgery. Front Cardiovasc Med 2022;9:887535. [Crossref] [PubMed]
- Peri V, Devlin P, Perry L, et al. Associations Between Nonanemic Iron Deficiency and Postoperative Outcomes in Cardiac Surgery: A Systematic Review and Meta-Analysis. Anesth Analg 2024;139:47-57. [Crossref] [PubMed]
- Tonino RPB, Wilson M, Zwaginga JJ, et al. Prevalence of iron deficiency and red blood cell transfusions in surgical patients. Vox Sang 2022;117:379-85. [Crossref] [PubMed]
- Skikne BS. Serum transferrin receptor. Am J Hematol 2008;83:872-5. [Crossref] [PubMed]
- Lopez A, Cacoub P, Macdougall IC, et al. Iron deficiency anaemia. Lancet 2016;387:907-16. [Crossref] [PubMed]
- Wish JB. Assessing iron status: beyond serum ferritin and transferrin saturation. Clin J Am Soc Nephrol 2006;1:S4-8. [Crossref] [PubMed]
- Pasricha SR, Tye-Din J, Muckenthaler MU, et al. Iron deficiency. Lancet 2021;397:233-48. [Crossref] [PubMed]
- Harms K, Kaiser T. Beyond soluble transferrin receptor: old challenges and new horizons. Best Pract Res Clin Endocrinol Metab 2015;29:799-810. [Crossref] [PubMed]
- Sierpinski R, Josiak K, Suchocki T, et al. High soluble transferrin receptor in patients with heart failure: a measure of iron deficiency and a strong predictor of mortality. Eur J Heart Fail 2021;23:919-32. [Crossref] [PubMed]
- Cerami C. Iron Nutriture of the Fetus, Neonate, Infant, and Child. Ann Nutr Metab 2017;71:8-14. [Crossref] [PubMed]
- Gao P, Jin Y, Zhang P, et al. Nadir oxygen delivery is associated with postoperative acute kidney injury in low-weight infants undergoing cardiopulmonary bypass. Front Cardiovasc Med 2022;9:1020846. [Crossref] [PubMed]
- Jacobs ML, O'Brien SM, Jacobs JP, et al. An empirically based tool for analyzing morbidity associated with operations for congenital heart disease. J Thorac Cardiovasc Surg 2013;145:1046-1057.e1. [Crossref] [PubMed]
- Kellum JA, Lameire NKDIGO AKI Guideline Work Group. Diagnosis, evaluation, and management of acute kidney injury: a KDIGO summary (Part 1). Crit Care 2013;17:204. [Crossref] [PubMed]
- Gaies MG, Jeffries HE, Niebler RA, et al. Vasoactive-inotropic score is associated with outcome after infant cardiac surgery: an analysis from the Pediatric Cardiac Critical Care Consortium and Virtual PICU System Registries. Pediatr Crit Care Med 2014;15:529-37. [Crossref] [PubMed]
- Dufour N, Radjou A, Thuong M. Hemolysis and Plasma Free Hemoglobin During Extracorporeal Membrane Oxygenation Support: From Clinical Implications to Laboratory Details. ASAIO J 2020;66:239-46. [Crossref] [PubMed]
- Spiezia L, Di Gregorio G, Campello E, et al. Predictors of postoperative bleeding in children undergoing cardiopulmonary bypass: A preliminary Italian study. Thromb Res 2017;153:85-9. [Crossref] [PubMed]
- Skikne BS, Punnonen K, Caldron PH, et al. Improved differential diagnosis of anemia of chronic disease and iron deficiency anemia: a prospective multicenter evaluation of soluble transferrin receptor and the sTfR/log ferritin index. Am J Hematol 2011;86:923-7. [Crossref] [PubMed]
- Hay G, Refsum H, Whitelaw A, et al. Predictors of serum ferritin and serum soluble transferrin receptor in newborns and their associations with iron status during the first 2 y of life. Am J Clin Nutr 2007;86:64-73. [Crossref] [PubMed]
- Domellöf M, Lönnerdal B, Dewey KG, et al. Sex differences in iron status during infancy. Pediatrics 2002;110:545-52. [Crossref] [PubMed]
- Restrepo-Gallego M, Díaz LE, Rondó PHC. Classic and emergent indicators for the assessment of human iron status. Crit Rev Food Sci Nutr 2021;61:2827-40. [Crossref] [PubMed]
- Fitzsimons S, Poppe KK, Choi Y, et al. Relationship Between Soluble Transferrin Receptor and Clinical Outcomes in Patients With Heart Failure According to Ejection Fraction Phenotype: The New Zealand PEOPLE Study. J Card Fail 2022;28:1255-63. [Crossref] [PubMed]
- Krawiec P, Pac-Kożuchowska E. Soluble transferrin receptor and soluble transferrin receptor/log ferritin index in diagnosis of iron deficiency anemia in pediatric inflammatory bowel disease. Dig Liver Dis 2019;51:352-7. [Crossref] [PubMed]
- Daude S, Remen T, Chateau T, et al. Comparative accuracy of ferritin, transferrin saturation and soluble transferrin receptor for the diagnosis of iron deficiency in inflammatory bowel disease. Aliment Pharmacol Ther 2020;51:1087-95. [Crossref] [PubMed]
- Zhu S, Liu C, Zhao C, et al. Increased Serum Soluble Transferrin Receptor Levels Were Associated With High Prevalence of Cardiovascular Diseases: Insights From the National Health and Nutrition Examination Survey 2017-2018. Front Cell Dev Biol 2022;10:874846. [Crossref] [PubMed]
- Kang M, Kwon S, Lee W, et al. Soluble transferrin receptor can predict all-cause mortality regardless of anaemia and iron storage status. Sci Rep 2022;12:11911. [Crossref] [PubMed]
- Zhu Y, Liu X, Li N, et al. Association Between Iron Status and Risk of Chronic Kidney Disease in Chinese Adults. Front Med (Lausanne) 2019;6:303. [Crossref] [PubMed]
- Litton E, Xiao J, Ho KM. Safety and efficacy of intravenous iron therapy in reducing requirement for allogeneic blood transfusion: systematic review and meta-analysis of randomised clinical trials. BMJ 2013;347:f4822. [Crossref] [PubMed]
- Corsi F, Pasquini A, Guerrera M, et al. Single shot of intravenous iron in cardiac surgery: The ICARUS study. J Clin Anesth 2023;84:111009. [Crossref] [PubMed]
- Larsson SM, Hillarp A, Karlsland Åkeson P, et al. Soluble Transferrin Receptor during infancy and reference intervals for the Roche Cobas platform. Int J Lab Hematol 2021;43:378-86. [Crossref] [PubMed]
- Speeckaert MM, Speeckaert R, Delanghe JR. Biological and clinical aspects of soluble transferrin receptor. Crit Rev Clin Lab Sci 2010;47:213-28. [Crossref] [PubMed]