Brain-gut-brain axis, nutrition, and autism spectrum disorders: a review
Introduction
A neurologically based developmental illness that first appears in childhood is known as autism spectrum disorder (ASD). It involves restricted or repetitive patterns of behavior, interests, and activities, as well as challenges in developing social relationships and interactions (1,2).
Over the past 20 years, there has been an enormous rise in both the occurrence and the prevalence of autism. With a male-to-female sex proportion between 2:1 and 3:1, it is more common in males. The cause of autism is mainly determined by genetics along with early environmental factors. According to epidemiologic research, only a small percentage of cases are attributed to external variables like teratogens, toxic environments, prenatal infections like cytomegalovirus (CMV) and rubella, and perinatal traumas (3,4). People with ASD can also have gut syndrome, a condition characterized by abnormal colonies of bacteria and gastrointestinal (GI) irritation that impairs bowel motility. There may be a basic connection between nutrition, ASD, and the gut microbiome. The wide range of GI symptoms present in ASD patients indicates the need to incorporate nutritional support into standard treatment. A healthy diet can help alleviate digestive, metabolic, and mental health symptoms. Unfortunately, very few medical professionals and dietitians monitor the dietary habits and food intake of patients with ASD (5). Approximately 25% of children diagnosed with ASD have at least one persistent digestive tract syndrome.
Moreover, GI problems may interfere with the absorption of vitamins and minerals. GI problems like diarrhea, constipation, discomfort in the abdomen, and gastric acid can be relieved by following a healthy diet. Scientific studies suggested that the pathophysiology of autism may start early in fetal development (2,4). One of the responsibilities of a dietitian is to assess a mother’s dietary status accurately before and during pregnancy. This assessment allows for dietary adjustments to be made as necessary to improve metabolic indicators (6). Eating habits may be impacted by some ASD traits, such as behavioral rigidity, a demand for uniformity, schedules, and routines (7). According to Nadon et al. (8), children with ASD likewise have a distinct perceptual profile that is susceptible to stringent food selection based on form, appearance, flavor, or warmth (9). These children may also exhibit low blood sugar or hyperactivity in response to tactile, smell, and visual cues. As a result, kids begin avoiding food and consume only a small amount of the items they eat regularly. In certain instances, kids may also exhibit digestive problems, physiological reactions to food, or difficulties with movement related to eating and chewing food (10-12). Specific diets are restricted due to these related disorders; for instance, acidic foods are frequently avoided, while sweet foods with high energy levels are encouraged (9,12). Diet and nutrition have crucial roles in maintaining a healthy body and mind. Consuming nutrient-rich foods can help prevent obesity (13).
Early-onset obesity can impact individuals across all age groups, including adults, adolescents, and children, as well as those with ASD and related illnesses. Eating disorders can lead to the accumulation of oxidative radicals, leading to a decline in both mental and physical health. Despite awareness of these issues, parents often find it challenging to regulate their children’s eating habits. Collaboration among doctors, parents, nutritionists, and dieticians is essential to enhance their quality of life.
According to the study of Esparham et al. (14), individuals with ASD suggest that there may be biological, metabolic, and dietary connections to the disorder in affected youngsters. Additionally, Rodop et al. (15) stated that everyone is required to eat well because it is one of our fundamental biological necessities. It is important for individuals with systemic symptoms like ASD to pay attention to their diet to enhance their quality of life and prevent the worsening of their condition. Different dietary strategies have been explored to alleviate ASD symptoms, especially in addressing digestive problems that individuals with ASD common experience. The relationship between microbiota, gut health, and brain functions has been taken into consideration in the development of various dietary interventions.
Abdelhaliem et al. (16) indicated that children with ASD often exhibit irregular feeding patterns and are at a higher risk of malnutrition compared to children without the condition. Consequently, it is essential to assess the dietary needs of kids with ASD to prevent nutrient deficiencies through dietary modifications or supplementation with the appropriate minerals and vitamins. Additionally, Caetano and Gurgel (17) found that children with ASD have higher rates of obesity and overweight, along with inadequate intake of essential vitamins and minerals. Also, Moiniafshari et al. (18) concluded that combining nutrition and exercise programs can have a more significant positive impact on individuals with ASD when implemented together.
Individuals with ASD commonly experience behavioral issues and GI problems due to disruptions in the gut-brain-microbiota axis. Research has explored the relationship between diet and gut-related issues, with this review aiming to investigate diet therapies for ASD patients and explore novel dietary interventions considering the microbiota-intestinal-brain axis.
Nutrition problems
According to Yang (19), feeding issues are conditions that have detrimental effects on dietary habits, growth, socialization, and psychology because of an individual’s incapacity or unwillingness to orally consume the necessary amounts of nutrients, fluids, or calories to thrive. Several feeding problems were listed by Rastam (20) in the DSM-5. The GI disorders pica/rumination disease (20) and avoidant/restrictive food consumption disorders are the most frequently observed in individuals with ASD. These feeding disorders include food rejection, a limited variety of foods, disrupted meal behaviors, food selectivity, and restricted intake (21).
Co-occurring conditions that often accompany ASD include symptoms related to the digestive tract, sleep problems, seizures, behavioral issues, attention-deficit/hyperactivity disorder, anxiety, and toileting difficulties (22-25). Another common issue associated with ASD is feeding problems (26). The incidence of feeding issues in children and adolescents with ASD varies greatly among studies. Previously, a study by Schreck et al. (27) found that 13–80% of individuals experience feeding difficulties. Nadon et al. (8) also supported this finding, reporting that 80% of children with ASD struggle with eating. Children with ASD tend to have more digestive issues and a narrower range of foods in their diet compared to children without ASD (28). Given the increasing recognition of feeding issues in recent years, it is likely that a significant proportion of individuals with ASD experience challenges related to food. Research indicates that concurrent eating issues could put a person with ASD at risk for health issues. Chronic alimentary problems can have a negative effect on health and development, leading to stunting, malnourishment, social impairments, and subpar academic performance (21). Major difficulties with food preparation are also related to deficiencies in skills, including oral-motor impairments that impact the child’s ability to chew, swallow, and suck (29). According to Schreck et al. (27), children with both ASD and feeding issues are more experienced with medical problems related to nutrition, such as osteoporosis or poor bone development (3). Children with ASD who struggle with eating can become malnourished and require invasive medical procedures like the insertion of tubes for nutrition, as noted in (30). Deficiencies in vitamins and minerals, such as reduced calcium intake (21), lowered levels of iron (31), and reduced vitamin C intake (32) are commonly observed in children with ASD who have eating difficulties (1). Research by Egan et al. (33) suggests that children with ASD tend to prefer foods high in fats or sweets, which aligns with the growing body of evidence indicating a higher risk of obesity in this population.
Nutrition disorder and symptoms of the GI
There are limited studies on the connection between digestive tract symptoms and feeding issues (26,34). According to Mannion et al. (35), GI symptoms had occurred in 79.3% of individuals with ASD. As pointed out by the last authors (35), 51.7% and 49.4% of those surveyed with ASD experienced abdominal discomfort and diarrhea as the most frequent GI symptoms. Additionally, research by Wang et al. (36) found that GI problems were significantly higher in children with ASD compared to typically developing children. Field et al. (37) noted that most participants had underlying health issues that contributed to their feeding difficulties. In research by Özen et al. (38) on GI symptoms and dietary habits in children with ASD, it was found that over 60% of the children had significant dietary preferences. The research indicated that some children experienced stomach symptoms on a weekly basis, and feeding issues were associated with bowel problems (38). To explore potential factors contributing to GI problems in children with development delays, Field et al. (37) examined nutritional issues in children.
They identified gastroesophageal reflux disease (GERD) as the most common condition in the group, closely linked to food aversion. Food aversion is a complex eating problem often observed in individuals with ASD. A study by Field et al. (37) reported that 14 out of 20 ASD children with selective eating also experienced constipation or diarrhea. Chronic digestive problems affect vitamin and mineral intake in 25% of children diagnosed with ASD. A healthy diet can help alleviate these symptoms. Dietitians evaluate mothers’ diets before and throughout pregnancy and make necessary adjustments to improve metabolic markers (6). Leaky gut syndrome is a potential side effect of individuals with ASD because of bacterial proliferation and inflammation. There is a proposed connection between food, gut microbiota, and ASD. Nutritional therapy is essential for relieving GI, metabolic, and psychological symptoms, but few doctors and nutritionists can provide close monitoring for patients with ASD (5). Additionally, eating disorders have been associated with an accumulation of reactive radicals, which can negatively impact both physical and mental well-being (13).
Effective therapies to enhance metabolic conditions and overall health in individuals with ASD have been the subject of current studies. Low levels of exercise, poor eating habits and quality, problems with behavior, and poor social relationships can all contribute to poor health. Dietary and exercise treatments have garnered considerable interest among the dozens of medical techniques. Owing to elevated inflammation levels, elevated interleukin-6 (IL-6), interleukin-8 (IL-8), and tumor necrosis factor-alpha (TNF-α), and decreased cell adhesion molecules (CAMs), nutrition and exercise will become increasingly crucial for individuals with ASD (39). It has recently been suggested that individuals with ASD may have a high frequency of nutritional deficits, potentially due to their food choices, eating habits, and restricted diets. By analyzing food logs and using the Healthy Eating Index (HEI) in comparison to the Dietary Reference Intake (DRI), nutritional assessment can help identify dietary patterns and nutritional status (40). Furthermore, nutritional quality and intake, as well as their impact on physiological markers such as IL-6, homocysteine, digestion, and other physiological markers, can be assessed using tools like dietary records and the Food Frequency Questionnaire (FFQ) (41).
Nutrition issues and challenging behavior
ASD is known for its repetitive and restricted habits, including regulated meals and rigid feeding schedules (34).
It has been claimed that problematic eating habits and eating issues may be linked to behavioral routines and mental obsessions associated with ASD. Social expectations of feeding circumstances can impact interpersonal abilities and interactions when mealtime behavior is problematic (21). Socialization challenges may affect the enjoyment of eating with others (8), potentially leading to problematic habits. Despite the recognition of the commonality of food issues and challenging behavior, there is a lack of understanding in research addressing the reasons underlying these actions (42).
In their research, Curtin et al. (43) explained the importance of children eating healthily and embracing family rituals such as dining at the table without electronics or television, engaging in family conversations, and learning to eat independently. Adjusting to new foods and eating routines can be particularly challenging for children with autism and ASD (44). Additionally, children with ASD may exhibit a specific eating pattern, given the connections between food issues and other concurrent disorders. Some children with ASD and eating difficulties may have a higher likelihood of demanding behavior, GI symptoms, co-occurring psychopathology, and sensory abnormalities. The condition known as ASD is diverse (45). Research by Hill et al. (46) suggests that children with ASD tend to avoid “fruits, vegetables, and whole grains” in favor of high-energy, low-nutrient diets. Children with autism and ASD, often labeled as “picky eaters”, may have preferences for specific food types, flavors, and textures, posing a significant challenge to their parents and caregivers (44). To address these issues, parents should introduce nutritious diets to their children during the weaning process.
In addition, specific diets, limited food preferences, the introduction of new meals, or physical activity can often sometimes trigger aggressive behavior in children with ASD, leading parents to accommodate their requests (44). Therefore, it is advisable to gradually provide new foods to these children to help them become accustomed to different flavors and textures (8).
Nutrition problems and sensory disorder
Successful behavioral methods for children with ASD must address sensory sensitivity, such as color, taste, and texture. As noted by Twachtman-Reilly et al. (47), sensory regulation is the process that enables a person to misprocess the vast amount of sensory data that continuously nourishes the neural network properly. Leekam et al. (48) found that sensory processing problems affect 78–90% of children with ASD. A study by Laud et al. (49) revealed that there is a common observation in research that sensory impairment or defensive behavior is the cause of the elevated incidences of feeding difficulties in ASD.
Cermak et al. (50) found that the sense of smell can play a significant role in clarifying food choice and that there is a connection between feeding problems and sensory defensive behavior. In their study, Nadon et al. (10) investigated the connection between the frequency of eating issues in kids with ASD and their sensory processing issues. They found a substantial correlation between the number of dietary disorders in kids diagnosed with ASD and their visual and aural sensitivity. The same authors identified phenotypes for additional co-occurring disorders, like sleep issues. Goldman et al. (51) supported a case study of the sleep phenotype associated with ASD. Żarnowska et al. (52) performed an investigation on the therapeutic use of a ketogenic diet (KD) in children with autism. The research showed notable clinical benefits, including improved attention span, enhanced interpersonal abilities, and decreased fear, anxiety, and mood disorders. They also linked the behavioral and psychological changes with decreased electron chain activity and decreased gene expression in the mitochondria. Several researchers have demonstrated that individuals on a carbohydrate-free diet develop a dependence on ketones, leading to decreased mitochondrial activity and reduced energy levels, which may contribute to observable changes in behavior (53,54).
Additionally, Zeybek and Yurttagul (40) have shown that these screening methods could be useful for tracking growth patterns and body types, particularly among teenagers and young adults with ASD. Numerous nutritional therapies are designed to address ASD by improving physical and physiological health by reducing food allergies and intolerances and improving dietary patterns (55). These treatments focus on promoting a moderately nutritious and healthy diet to improve lipid profiles, body mass index (BMI), and metabolic status in individuals with ASD (56). Improving health is a critical aspect when addressing ASD, as studies have demonstrated the links between eating behaviors and elevated insulin levels, obesity, chronic inflammation, and metabolic syndrome in individuals with ASD. These conditions can be positively impacted by implementing effective nutritional therapies (57).
There is a suggestion that a greater frequency of systemic inflammation in ASD may lead to various metabolic abnormalities, such as insulin resistance and dyslipidemia, that can be treated with dietary therapies. The approach could be beneficial as individuals with ASD often have limited dietary intake, with refined foods making up a significant portion of their diet, potentially exacerbating the inflammatory response (58). Vitamin D3 and omega-3 fatty acids, when consumed as nutrients, have been shown to reduce inflammatory reactions in individuals with ASD, potentially improving metabolism (58).
Table 1 summarizes a few of the research topics we covered. Figure 1 illustrates how controlling metabolism through nutrition might improve the lives of kids with autism and ASD.
Table 1
Summary | Sample size (N) | References |
---|---|---|
Discovered that the ketone diet significantly reduces eating, tantrums, and behavior | 35 in addition to one case study | (52) |
Discovered sensory problems with food and dietary habits. The sensory patterns of these children can be completely uncontrollable and dissimilar | 95 instances compared siblings within the same family who were not autistic to autistic children | (8) |
To alleviate symptoms, a ketogenic diet lowers the expression of the mitochondrial gene | – | (53) |
Due to the decrease in mitochondrial gene expression brought about by a ketogenic diet, behavior, hunger, and other major symptoms of autism improved dramatically | – | (54) |
Tantrums, expressive language, autism and ASD, and improved PGI-R subscales are all areas where minerals and vitamins can be helpful | 141 | (59) |
Diets that are vegetarian and gluten-free improve behavioral outcomes | – | (60) |
Alternative and complementary therapies worked better for most children. The greatest outcomes and fewest adverse reactions came from cutting back on medication and raising melatonin | 376 | (61) |
A rise in sociability, motor abilities, and physical exercise controls appetite and alters psychological, emotional, and interpersonal growth | 35 | (62) |
ASD, autism spectrum disorder; PGI-R, patient global impression-revised.
The significance of microbes in nutrient metabolism
A population of microbes consisting of bacteria, fungi, viruses, archaea, and parasites is referred to as a microbiome (63). All multicellular species that have been studied, including plants and animals, have microbiota. After birth, bacteria begin to colonize the body, and by the ages of three to five, they resemble the mature microbiome, which then stabilizes largely (64). The absorption of drugs and defense against pathogens is influenced by microbiota (65). The microbial flora in the stomach affects the regulation of hunger, energy utilization, stomach processes, and nutrient uptake (66). Many human diseases, such as cancer, metabolic syndrome, bowel inflammation, obesity, and neurological disorders, have been linked to the disruption or imbalance of the microbiota (67,68).
The brain and the GI tract interact in several significant ways. One method is through the parasympathetic nervous system’s Vagus nerve. The Vagus nerve serves as an interface between the brain and the intestinal tract, distinguishing between harmful and beneficial bacteria and transmitting messages with anxiogenic and calming properties (67). Bacterial species also play a role in generating immune-regulating metabolites, such as short-chain fatty acids produced during the decomposition of carbohydrates. According to Louis et al., short-chain fatty acids like propionic acid (PPA), butyric acid (BA), and acetic acid (AA) offer various benefits to the host (68), including improved lipid profile, colon health, and weight control. However, the accumulation of these acids, particularly PPA, can also impact the neurological system and has been linked to ASD (69-72).
Propionate reduces serotonin (5-hydroxytryptamine, or 5-HT) release in the gut. It affects the functioning of the central nervous system and acts as a neurochemical. Low levels of dopamine and 5-HT in the brain are believed to be responsible for these effects (73). Additionally, they may have a role in hyperserotonemia (excessive serotonin production) that is seen in autistic children (74). Certain symptoms of ASD may arise due to the displacement of the brain by transporters or passive diffusion through the blood-brain barrier of higher levels of microorganisms that produce short-chain fatty acids (such as Clostridia, Desulfovibrio, and Bacteroides) found in autistic individuals (75). While the exact mechanism of how short-chain fatty acids affect behavior is still unclear, both epigenetic modifications and mitochondrial dysfunction (Krebs cycle) may be involved (Figure 2) (73). Another important factor for social interaction and repetitive behaviors is 5-HT. Due to its high production in the gut and metabolism by intestinal microbiota, 5-HT is implicated in regulating neurodevelopment (76).
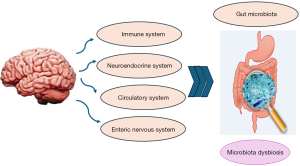
According to reports, certain bacterial species (Lactobacillus and Clostridium spp.) found in the feces specimens of children with autism can alter the metabolism and functionality of neurons such as 5-HT and catecholamines, exacerbating symptoms associated with serotonergic structure malfunction (76,77). Approximately 30% of children with autism have high levels of 5-HT in their red blood cells and blood cells, making 5-HT a potential indicator for the presence of ASD (78). Intestinal permeability may allow 5-HT to enter the bloodstream and elevate blood levels of the hormone (78,79). Furthermore, reduced 5-HT inactivation can lead to increased 5-HT levels, which are associated with intestinal inflammation and may contribute to the inflammatory response (80). Contrary to popular belief, the availability of tryptophan and serotonin synthesis can be affected by an imbalance (disturbance of the microbiome) that reduces the absorption of amino acids from food. A decrease in tryptophan levels is linked to the exacerbation of repetitive behaviors observed in individuals with ASD (81). As Rodop et al. (15) emphasized, dietary habits are crucial for individuals with ASD or other systemic symptoms to maintain a better quality of life and slow the progression of their condition. Various diets have been explored to alleviate ASD symptoms, with a focus on addressing digestive issues in individuals with ASD (82). Many dietary regimens have been designed with the gut-brain-microbiota axis in mind.
Different types of treatment for metabolic status in ASD
Increasing the levels of physical activity seems to be an effective additional treatment to improve the metabolic state in individuals with ASD and enhance dietary conditions. Since ASD is more prevalent in individuals who are overweight and have lower levels of the hormone’s adiponectin, ghrelin, and leptin, this health concern is becoming more significant (83). Various physical exercise strategies are recommended as beneficial activities for individuals with ASD; however, it is crucial to consider that ASD has specific needs. Therefore, some activities that are recommended or utilized may not be suitable for individuals with ASD.
Moderate-intensity physical activity has been demonstrated to enhance metabolic reactions and inflammatory responses, even without changes in adipose tissue. Regular participation in higher-intensity physical activity has been linked to multiple reported benefits (84,85). The optimal amount of physical activity is still debated in several studies. Some studies suggest that higher-intensity physical activities may increase inflammation, and longer rest intervals may have the opposite effect (86). This finding seems to improve the metabolic condition of individuals with ASD. There is growing interest in the impact of different types of sports on the health of individuals with ASD.
Aerobic exercises, which can be performed both indoors and outdoors, are among the physical activities most frequently prescribed for individuals with ASD. In the study of Shahrasfenghar et al. (87), these exercises can have greater positive effects, particularly when recommended for longer periods. They may improve physical fitness and metabolic conditions in people with ASD. When combined with dietary therapies such as vitamin D3 supplements, aquatic sports can be an effective way to enhance the metabolic state of individuals with ASD (58).
Recently, there has been a notable increase in the popularity of aquatic physical exercise, and several therapies, such as combining it with vitamin medication, are beneficial for reducing inflammation and enhancing metabolic conditions in people with ASD (55). An improved inflammatory reaction could result in an improved metabolic state. Following Mazahery et al. (58), there is evidence to suggest that incorporating water-based activities with the addition of vitamin D3 may enhance the BMI, waist-to-hip ratio (WHR), and other demographic metrics, including cholesterol levels, in individuals with ASD.
Other therapeutic techniques for enhancing cardiovascular alterations in cholesterol levels and metabolic states in ASD include coordinated physical activity (88). Additional physical activity interventions could involve indoor and home-based activities, which were particularly important throughout the coronavirus disease 2019 (COVID-19) pandemic. Individuals with ASD spent more time at home due to quarantines and lockdowns. Given the poor physical fitness of many individuals with ASD, this kind of physical activity has concentrated on improving strength and flexibility in a safe, comfortable setting under the guidance of a therapist. Typically, these exercises are recommended to enhance body composition, manage weight, and prevent metabolic illnesses (89,90).
Some diets that affect ASDs
KD
A KD consists of very low carbohydrates, adequate protein, and high fat. It has a proven track record of working for patients who are resistant to anticonvulsant medication therapy, particularly those with epilepsy (91). Studies have also shown that a KD can reduce the severity of behavioral issues and seizures in patients with mild to severe ASD (92,93). In one study, pregnant Swiss rats were injected with valproic acid (VPA) on the eleventh day of pregnancy at a dose of 600 mg/kg. The rats were divided into two groups: a control group that did not receive VPA and a treatment group that did. Half of each group was fed a KD, while the other half was fed a standard diet (SD). After 70 days, a significant difference in social behavior was observed between the VPA mice that received SD and those that were treated with KD (94).
In another study, male and female epileptic (EL) mice with ASD and epilepsy were evaluated for their behavior, as they are commonly used in models of autism and epilepsy. Three different diet regimens were tested for 3 to 4 weeks: one SD and two KD, with one KD having a higher fat ratio. In the social recognition test, male and female mice in the KD-fed groups spent more time interacting with a foreign mouse. It was recently discovered that preferences for novel mice increased, especially in females on a higher-fat KD. Meanwhile, self-grooming, a repetitive behavior test, significantly decreased in male mice. According to Ruskin et al. (95), the decrease in self-grooming activity among females was not statistically significant.
In a human pilot study, thirty children diagnosed with ASD were examined. They followed a diet consisting of 30% medium-chain triglycerides, 30% heavy cream, 11% saturated fat, 19% carbohydrates, and 10% protein for 6 months. Forty percent of the children either could not tolerate the diet or did not adhere to it. Only two children showed significant improvements on the Childhood Autism Rating Scale (CARS), while the rest demonstrated modest to moderate improvements (92).
In another study, a child who experienced both seizures and autism was placed on a KD with a fat ratio of 1.5 to protein and carbohydrates. Several benefits were observed following the diet, including improvements in behavioral features and reversal of morbid obesity and dementia. After adhering to a similar diet for a few years, the child’s IQ increased by 70 points, and his severe autism classification changed to a non-autistic condition as his CARS rating decreased from 49 to 17 (30). Seizures also ceased after following the diet for 14 months. Although the KD was originally developed for treating EL seizures, it requires a high-fat content, moderate carbohydrates, and adequate protein. Studies have shown that the KD reduces repetitive movements and promotes social interactions in mice and rats.
Furthermore, children on the KD exhibited higher IQ scores and lower CARS scores. Conducting further research on the efficacy of the KD for ASD will help establish the correlation between the two. Additionally, parents of the children noted reductions in common ASD symptoms such as inattention, speech difficulties, attention issues, and gaze problems (96).
In another trial, 15 children with ASD received extra medium-chain triglycerides oil and a modified ketogenic, gluten-free meal. This intervention was implemented due to the presence of both primary and secondary mitochondrial issues in individuals with ASD. After 3 months, the children showed significant improvements in their Impact on Society and Total Plan-2 scores for ASD monitoring. Furthermore, parents of these children observed reductions in common ASD symptoms, including inattention, speech difficulties, attention issues, and gaze problems (96).
Diet is crucial for treating GI issues that individuals with ASD experience (15). Several dietary regimes have been developed with the gut-brain-microbiota axis kept in mind. Some studies have examined how ASD and neurodevelopmental problems are affected by the Mediterranean diet, the low-oxalate diet, camel’s milk, the gluten-free/casein-free eating habits, and the KD. Nevertheless, the investigation provides scant evidence to support the efficacy of these diets. Table 2 provides an overview of the studies covering the impact of KD treatment on autism.
Table 2
Results and conclusions | Method | Sample | References |
---|---|---|---|
The KD and SD groups experienced a novel environment, which resulted in enhanced motor behavior and reduced anxiety. In KD, limiting calories is more crucial than food composition | KD “(7.2 kcal/g energy, 3.3% cho, 16.7% pro, 80% fat) and SD (4.1 kcal/g energy, 65.0% cho, 28.1% pro, 6.9% fat)” for 30 days | 19 Mecp2 mice, 12 controls, and an RTT model | (97) |
It is believed that KD considerably reduces seizures, which is greater than the GFCF diet | utilizing reports from parents to record seizures and the basic symptoms of ASD (attention, behavior, communication, etc.) while putting KD into practice | One hundred forty-six children with ASD who had seizures and a control group | (98) |
KD helps the mice exhibit fewer autistic behaviors. Mice administered KD have shown to be more gregarious | KD or a control diet | Mice model BTBR | (99) |
In terms of bioenergetic disorder, KD could get well. KD has changed complex behaviors and metabolism of mitochondria, and this change may be useful in the treatment of ASD | Control and KD | Mice model | (100) |
The KD-fed mice showed a rise in brain norepinephrine, but there was no alteration in peripheral monoamine levels | KD or a control diet | Adult engrailed two null mice, wild-type mice | (101) |
KD modulated motor input and corrected reduced motion thresholds | In this research, the BTBR mice’s baseline system excitability was examined | BTBR mice model | (102) |
VPA-KD enhanced several social behaviors. Compared to VPA-SD, VPA-KD scored higher in friendliness and social novelty ratings | Four groups were investigated in this investigation: (I) supplemented with SD, (II) VPA mice fed with SD (VPA-SD), (III) VPA mice fed with KD (VPA-KD), and (IV) fed with KD | Mice model | (94) |
In mice, KD has reduced several neurological signs linked to ASD | For 10–14 days, use either SD or KD (75% kcal fat) | BTBR and C57BL/6 (B6) mice | (103) |
There was reduced habitual conduct and increased social behavior and interaction in mice fed KD | KD and SD for 3–5 weeks. KD: 3.2% cho, 75.1% fat, 8.6% pro, 7.24 kcal/g energy. SD: 57.9% cho, 5% fat, 23.9% pro, 3.02 kcal/g energy |
28 control, 30 BTBR mice | (95) |
Following KD medication, ASD impairments related to myelin production and white matter growth improved in both brain areas | Investigated alterations in gene expression in BTBR mice after KD therapy | BTBR mice | (104) |
For men, KD eating completely or partially corrected all MIA-induced behavioral problems; female behavior was unaffected. KD decreased MIA-elevated self-directed repetitive behavior | KD or control diet for 3 weeks | Young adult C57Bl/6 mice | (105) |
In Glut3+/− males, but not in females, enhanced synaptic protein levels and spontaneous excitatory postsynaptic activity decrease hippocampal glucose concentration and brain amyloid β1-40 accumulation. KD somewhat restores social skills, although vocalization or cognitive deficiencies are not | Assessed sociability and electroencephalogram seizures in KD patients | Glut3+/− mice | (106) |
KD, ketogenic diet; SD, standard diet; cho, carbohydrates; pro, protein; RTT, Rett syndrome; GFCF, gluten-free casein-free; ASD, autism spectrum disorder; VPA, valproic acid; MIA, maternal immune activation.
Probiotics supplementation
Probiotic treatment for individuals with ASD is a new area of research and interest. Currently, there are very few investigations on the benefits of probiotics in people with ASD. This is compounded by the wide variety of probiotics available and the lack of knowledge regarding side effects, therapeutic benefits, proper dosage, and strains that are beneficial for individuals with ASD. Probiotics can influence the gut microbiota and potentially reduce gut permeability and GI inflammation (107). One study involved administering probiotics to children with ASD aged between two and nine. The results showed a decrease in intestinal inflammation and restoration of the abnormal GI microbiome (108). Several other studies have also demonstrated reductions in ASD symptoms and GI issues (109,110). Another study suggested that probiotic supplementation may reduce the risk of developing neuropsychiatric disorders later in childhood through mechanisms beyond gut microbiota composition (111).
Multiple ideas support the possible processes underlying probiotics and their relationship with ASD. Firstly, the opioid-excess explanation explains why the gluten-free casein-free (GFCF) dietary is effective. It proposes that probiotics can aid in digesting gluten products without significantly altering intestinal permeability, preventing peptide leakage, and fortifying the blood-brain barrier (112,113). This finding is also supported by the second theory, which deals with dietary protein allergies. According to this notion, probiotics’ anti-inflammatory properties may be able to mitigate the inflammatory and immunological responses that people with ASD experience, possibly even helping with behavioral issues related to the disorder (114). The last theory is based on using probiotics to treat gut dysbiosis and decrease endotoxin generation. This would lessen gut permeability and inflammation and stop endotoxins from damaging the central nervous system (107). This notion has been validated by multiple research projects, which have also shown a linked finding of enhanced behavioral results (115).
Additionally, Iovene et al. (116) discovered that gut dysbiosis in ASD patients is indicated by a drop in Lactobacillus and Clostridium spp. and a rise in invasive Candida spp. This dysbiosis raises intestinal permeability and has a significant relationship with the intensity of ASD symptoms. Questions have been raised about supplementing mothers with probiotics to prevent ASD and other disorders based on the benefits of probiotics. It is reasonable to hypothesize that prescribing probiotics to mothers could be a significant area of research for preventing disorders in individuals, especially ASD, as there is evidence linking gut health to human illnesses (117). To determine if adding probiotics can be beneficial for pregnant mothers as a preventive measure for ASD, more research is needed. Despite varying opinions and conclusions, the response has been cautiously optimistic. One group has stated that there is currently no conclusive evidence of the beneficial impact of oral probiotics on ASD. However, many studies on similar topics have indicated the need for further research (118). On the other hand, some argue that probiotic supplements could be an adjunct treatment (110). More research is needed to fully understand the role of probiotics as a therapeutic option for ASD.
Specific carbohydrate diet (SCD)
Sidney Haas developed the strict SCD in the 1920s to treat celiac disease. Later, the mother of an individual cured by Haas of irritable bowel syndrome promoted it (119). The goal of SCD is to restrict the intake of complex carbohydrates, starches, and disaccharides such as lactose, maltose, and sucrose to reduce the symptoms of gut microbial overgrowth and carbohydrate digestion. Instead, the diet recommends foods high in monosaccharides such as glucose, fructose, and galactose, including fruits, vegetables, honey, meats, eggs, nuts, and certain legumes. Foods like grains and potatoes, sugar, dairy products, and refined foods are examples of restricted foods.
Studies showing dysbiosis and reduced absorption and digesting of carbohydrates in individuals with ASD provide support for the use of SCD for autism (120,121). It is thought that these disruptions in the microbiota may contribute to the coexisting GI symptoms and possibly even the behavioral symptoms. However, there is a lack of published data supporting the safety or effectiveness of SCD in individuals with ASD, as it is a commonly used dietary therapy with and without professional guidance. The authors investigated the use of SCD for treating GI issues in a person with fragile X syndrome and ASD. They observed improvements in behavioral, nutritional, and GI aspects, and the nutritional intervention was well-received (122). Despite these positive results, there are concerns about potential nutritional deficiencies that may arise from restrictive dietary therapies, especially in cases where professional supervision is lacking or in individuals with limited dietary variety and selective eating habits. These findings highlight the need for further research before implementing the SCD regimen in individuals with ASD.
Dietary supplements vitamins
Vitamin A
The cause of ASD is also linked to several other nutritional deficits, including hemoglobin and the vitamins A, C, B6, B12, folate, and D. These potential deficiencies have prompted several supplement studies aimed at addressing ASD symptoms. Like polyunsaturated fatty acids (PUFAs), several research studies have shown that vitamin A insufficiency is frequently observed in children with ASD diagnoses (123). In China, 154 children participated in a nationwide survey, which revealed that the incidence of blood vitamin A deficiency was low among children with ASD. Still, it was adversely connected to CARS ratings (P=0.021) (124). Regarding potential pathologies linked to a lack of vitamin A for ASD, especially those connected to the pregnant woman’s oxytocin-CD38-vitamin A pathway, authors of additional research have called for the establishment of standards for vitamin A in infants and expectant mothers (125).
Additionally, studies (126,127) found that a vitamin A deficiency could cause ASD in rats. When puppies with gestational vitamin A insufficiency were given vitamin A supplements, their autistic-like behaviors improved. This suggests that the deficiency inhibits the retinoic acid receptors b signal transmission.
Research on vitamin A supplementation in children with ASD has been limited or accompanied by caution. Guo et al. (123) found that supplementing with vitamin A significantly reduced autism symptoms. Still, they also cautioned that some children with ASD may benefit more from daily vitamin doses than others. Similarly, researchers stressed the need for more research to determine the process supporting the link between ASD and vitamin A before drawing firm conclusions from another study. It is important to note that vitamin A supplementation increased vitamin A intake, which may not be desirable, according to a continuous investigation into nutritional supplements in children with ASD. These findings were supported by another study, which found that certain age groups of children with ASD consumed excessive amounts of vitamin A (128).
Additionally, based on the findings of a study examining the effects of vitamin A supplementation on gut microbes, researchers hypothesized that while vitamin A may influence autistic symptoms (129), there is no clear relationship between vitamin A levels, supplementation, and ASD symptoms (130). Overall, these results suggest that there may be a mechanism between vitamin A deficiency and ASD, with the b-CD38-oxytocin retinal transporter axis, also known as the oxytocin-CD38-vitamin A axis, being a promising potential mechanism. However, supplementing to address a vitamin A deficiency has not been shown to be an effective therapy for ASD symptoms in recent research.
Vitamin C
Many studies on vitamin C and ASD approach the subject from the perspective of nutritional deficiency, as individuals with ASD are particularly prone to eating difficulties and inadequate nourishment. A case report implemented by Rafee et al. (131) highlighted a patient with significant dietary selectivity leading to severe vitamin C deficiency; another case involved a 10-year-old boy with scurvy and anemia (132).
Remarkably, scurvy has been frequently shown in children with ASD, with seven cases reported at Boston Children’s Hospital among patients with developmental disorders (133). Similar findings have been reported in other studies (134,135). Likewise, a comparison of nutritional intake between 50 typically developing children and 63 children with ASD revealed that a higher proportion of children with ASD had lower vitamin C intake (136). A controlled trial involving male children and teenagers diagnosed with ASD, along with findings from comparison research conducted in Valencia, Spain (137), further supported these findings.
Additionally, data showed that supplementation led to excessive vitamin C in 2- to 3-year-old children with ASD (128). The long-term investigation on dietary supplements in children with ASD (n=288) highlighted the issue of overconsumption of micronutrient supplements among children diagnosed with ASD, particularly in the case of vitamin C (128). However, the Chinese study on the relationship between symptoms and nutrition in ASD did not specifically examine vitamins (124). Scientifically, it has been hypothesized that these increased vitamin C levels might be advantageous in addressing oxidative stress. In a randomly assigned, blinded, placebo-controlled mineral and vitamin treatment research, children with ASD who received vitamin C showed a significant reduction in oxidative damage [measured by the glutathione disulfide (GSSG), ratio to decreased glutathione (GSH)]. It was proposed that these elevated vitamin C levels helped these individuals experience a considerable decrease in oxidative stress (59).
Additionally, micronutrients such as folate, vitamins B6, and B12 are involved in methylation and transculturation processes. Methylation-dependent epigenetic control, involving the methylation of DNA and histone proteins, is a key component of the programmed changes in gene expression that characterize healthy neurodevelopment (138). Low GSH and oxidative stress levels are linked to deficiencies in methylation status in ASD, supporting the idea of dietary supplements containing micronutrients that promote methylation (139).
Vitamin B
The transsulfuration pathway connects the methylation process from MET to GSH production by converting homocysteine (HCY) to CYS. Folate, also known as 5-methyltetrahydrofolate (5-MTHF), and vitamin B12, or methylcobalamin (methyl-B12), are necessary cofactors for MET synthase (MTR) to convert HCY to MET. Vitamin B12 is particularly vulnerable to oxidation as it awaits the replacement of the methyl group by 5-MTHF during the catalytic cycle of MTR (140).
Oxidative stress can exacerbate the inactivation of MTR, which leads to an overproduction of HCY. This excess HCY can be redirected to the transsulfuration route, which boosts the production of GSH, a type of antioxidant. The interplay between oxidative stress and methylation processes can impact regulatory functions, as vitamin B12 serves as a sensor of cellular redox status. Therefore, the presence of oxidative stress in ASD is likely to disrupt neurodevelopment regulated by epigenetic mechanisms. Vitamin B6 is an important cofactor for the transsulfuration pathway proteins cystathionine-β-synthase (CBS) and cystathionine-γ-lyase, also known as cystathionase (CSE). Vitamin D stimulates the development of both CBS and CSE (141). Evidence suggests that brain cells could lack a transsulfuration mechanism, as evidenced by the significant finding that CBS expression is limited to astrocyte cells (142). Reduced cystathionine concentrations suggest that human brain CSE action is low, particularly in younger age groups, but increases beginning in the fifth decade of life (143), TNF-α, or TNF, a cytokine whose concentrations rise with aging (144), induces CSE expression. Cysteine concentrations in the spinal canal are higher compared to those in the cerebral cortex of young children with ASD, which is compatible with premature CSE production induced by TNF-α (145).
From a molecular standpoint, it has been suggested that certain indications of ASD might be due to inadequacies in the neurotransmitter system and that vitamin B6 could improve these mechanisms due to its involvement in the production of neurotransmitters (59). However, researchers found that supplementing with B6 and magnesium did not significantly improve symptoms of ASD in a 2017 evaluation of seven randomized controlled trials (RCTs) (146). The limited number of available high-quality trials led to a similar conclusion: there was insufficient data to support the effectiveness of the therapy (147). It’s crucial to remember that the American Psychiatric Association and the American Academy of Pediatrics have stated that megavitamin treatment for learning disabilities and autism is not justified when discussing the use of vitamin B6 and magnesium supplementation (148). This may explain the lack of current studies on their effectiveness (148). However, a study suggests that plasma glutamine concentrations could predict the response to vitamin B6 in individuals with ASD. However, the study had limitations, such as a small sample size and a low percentage of B6 sensitivity in ASD (149). Despite this, research has shown that children with ASD often have low B6 intake (129).
Camel milk
With only 2 percent fat, camel milk is primarily made up of polyunsaturated fats with long chains, which are essential for brain growth. Camel milk tastes watery because of its low-fat content (150). Camel milk has a similar protein level (3.2%) to cow’s milk. However, camel milk does not include the allergens beta-lactoglobulin and beta-casein found in cow’s milk. Incorrect separation of these caseins can lead to the synthesis of opioids (casomorphin) in children with autism (151). According to the research of Shabo et al. (152), the absence of these caseins in camel milk can inhibit the onset of symptoms associated with autism. Camel milk contains bacterial immune system enzymes and defense proteins that have beneficial and antibacterial properties (153). Additionally, camel milk includes insulin. Despite camel milk and cow’s milk having similar amounts of lactose, camel milk is suitable for consumption by individuals who are lactose intolerant.
Additionally, it is an excellent supply of vitamin C, calcium, and iron. Its high vitamin C content ensures a low pH, which helps in the more effective absorption of calcium and iron (150). Camels have a unique heavy-chain antibody construction, which lacks a light-chain antibody organization, making their antibodies distinct from other types. These antibodies are active against numerous viral illnesses and can effectively neutralize enzymes, giving them an advantage over human antibodies. Camel serum contains antibodies against ‘IgM, IgG, IgA, and IgD’ (154). Individuals with weakened immune systems may benefit from the smaller size of camel antibodies (152). The immunoglobulins in camel milk are absorbed by the recipient, impacting tissues and potentially affecting autoimmune disorders like ASD. Improper breakdown of casein in camel milk can lead to the production of opioid casomorphin, which can compromise the body’s defenses and result in brain injury, leading to behavioral and cognitive abnormalities (150).
There is a theory that autoimmune diseases and autism may be related. Intravenous immunoglobulin therapy (IVIGT) is linked to a decrease in the intensity of mental and emotional signs. Children under ten have shown full recovery after receiving camel milk or IVIGT therapy. When IVIGT or camel milk therapies were stopped in children above the age of 15 years, it was seen that the same symptoms reappeared. Eliminating cow’s milk from the diet suppresses symptoms but does not cause the condition to reverse. Parents have noticed that younger children respond more positively to camel milk than older children, suggesting that camel milk may help prevent brain damage from developing at a young age (150). In animals used for research, the idea that morphine-like effects result from milk casein breaking down abnormally in autistic children was accepted. Beta-casomorphin-7 was administered to rats (155). The booming injection-related bell did not pique the curiosity of the rats who had watched it ring. The analysis of the brain tissues from laboratory animals, 32 different brain areas contained beta-casomorphine-7, which is involved in vision, hearing, and communication (150).
Randomized trials were done to explore how camel milk intake affected the indicators of oxidative stress in kids with autism. Sixty autistic children participated in this study. These children were divided into three groups at random: those who drank raw camel milk, those who drank cooked camel milk, and those who drank cow’s milk, which was used as a placebo. Each child drank 500 cc of milk regularly for 2 weeks. The Childhood Autism Assessment Scale (CAAS) improved, and ‘plasma glutathione, superoxide dismutase, and myeloperoxidase levels’ rose in groups that drank camel milk. According to Al-Ayadh and Elamin (156), these results suggest that camel milk can help improve autistic behaviors by reducing oxidative stress associated with autism (157).
Conclusions
Behavioral and speech interventions for ASD aim to improve social interaction, while dietary therapies for GI disorders have garnered significant interest. However, there is a lack of scientific research on the effects of therapeutic diets on autism, leading to no definitive dietary recommendations. Dietary supplements have shown promising results in addressing GI issues and behavior in individuals with ASD. Nevertheless, the efficacy of these therapies is often inconsistent due to the lack of blind studies, control groups, and high expectations. Additionally, the use of various outcome measures, such as questionnaires and diagnostic tools, can skew results, and small sample sizes limit the generalizability of findings. It is essential to standardize the assessment of ASD symptoms and evaluation methods. Nutritional interventions can complement integrated psychological treatment, occupational therapy, speech and language therapy, and educational programs. Further research is needed to identify the factors contributing to positive and negative outcomes and develop effective and straightforward therapies to improve symptoms.
Acknowledgments
The author thanks Umm Al-Qura University for their support.
Funding: None.
Footnote
Peer Review File: Available at https://tp.amegroups.com/article/view/10.21037/tp-24-182/prf
Conflicts of Interest: The author has completed the ICMJE uniform disclosure form (available at https://tp.amegroups.com/article/view/10.21037/tp-24-182/coif). The author has no conflicts of interest to declare.
Ethical Statement: The author is accountable for all aspects of the work in ensuring that questions related to the accuracy or integrity of any part of the work are appropriately investigated and resolved.
Open Access Statement: This is an Open Access article distributed in accordance with the Creative Commons Attribution-NonCommercial-NoDerivs 4.0 International License (CC BY-NC-ND 4.0), which permits the non-commercial replication and distribution of the article with the strict proviso that no changes or edits are made and the original work is properly cited (including links to both the formal publication through the relevant DOI and the license). See: https://creativecommons.org/licenses/by-nc-nd/4.0/.
References
- Bandini LG, Anderson SE, Curtin C, et al. Food selectivity in children with autism spectrum disorders and typically developing children. J Pediatr 2010;157:259-64. [Crossref] [PubMed]
- American Psychiatric Association. Diagnostic and statistical manual of mental disorders. 2013, (5th ed.). Available online: https://doi.org/
10.1176/appi.books.9780890425596 - Hediger ML, England LJ, Molloy CA, et al. Reduced bone cortical thickness in boys with autism or autism spectrum disorder. J Autism Dev Disord 2008;38:848-56. [Crossref] [PubMed]
- Herndon AC, DiGuiseppi C, Johnson SL, et al. Does nutritional intake differ between children with autism spectrum disorders and children with typical development? J Autism Dev Disord 2009;39:212-22. [Crossref] [PubMed]
- Institute of Medicine (US) Subcommittee on Interpretation and Uses of Dietary Reference Intakes; Institute of Medicine (US) Standing Committee on the Scientific Evaluation of Dietary Reference Intakes. DRI Dietary Reference Intakes: Applications in Dietary Assessment. Washington (DC): National Academies Press (US); 2000.
- Ledford JR, Gast DL. Feeding problems in children with autism spectrum disorders: A review. Focus Autism Other Dev Disabil 2006;21:153-66.
- Matheson BE, Douglas JM. Overweight and obesity in children with autism spectrum disorder (ASD): A critical review investigating the etiology, development, and maintenance of this relationship. Review. J Autism Dev Disord 2017;4:142-56.
- Nadon G, Feldman DE, Dunn W, et al. Association of sensory processing and eating problems in children with autism spectrum disorders. Autism Res Treat 2011;2011:541926. [Crossref] [PubMed]
- Hubbard KL, Anderson SE, Curtin C, et al. A comparison of food refusal related to characteristics of food in children with autism spectrum disorder and typically developing children. J Acad Nutr Diet 2014;114:1981-7. [Crossref] [PubMed]
- Nadon G, Feldman DE, Dunn W, et al. Mealtime problems in children with autism spectrum disorder and their typically developing siblings: a comparison study. Autism 2011;15:98-113. [Crossref] [PubMed]
- Barnhill K, Gutierrez A, Ghossainy M, et al. Dietary status and nutrient intake of children with autism spectrum disorder: A case-control study. Res Autism Spectr Disord 2018;50:51-9.
- Leader G, Tuohy E, Chen JL, et al. Feeding Problems, Gastrointestinal Symptoms, Challenging Behavior and Sensory Issues in Children and Adolescents with Autism Spectrum Disorder. J Autism Dev Disord 2020;50:1401-10. [Crossref] [PubMed]
- Doreswamy S, Bashir A, Guarecuco JE, et al. Effects of Diet, Nutrition, and Exercise in Children With Autism and Autism Spectrum Disorder: A Literature Review. Cureus 2020;12:e12222. [Crossref] [PubMed]
- Esparham AE, Smith T, Belmont JM, et al. Nutritional and Metabolic Biomarkers in Autism Spectrum Disorders: An Exploratory Study. Integr Med (Encinitas) 2015;14:40-53.
- Rodop BB, Başkaya E, Altuntaş İ, et al. Nutrition Effect on Autism Spectrum Disorders. J Exp Med Sci 2021:2:007-017.
- Abdelhaliem HS, Sheha HG, Habib EE. The nutritional and some metabolic markers of autistic children. Egypt J Nutr Health 2021;16:21-34.
- Caetano MV, Gurgel DC. Nutritional profile of children bearing autism spectrum disorder. Rev Bras Promoç Saúde 2018;31:1-9.
- Moiniafshari K, Kalantari F, Nezhad HB. How Different Health-Related Interventions Improve Metabolic Impairments in Individuals with Autism Spectrum Disorder. Int J Diabetes Metab Disord 2022;7:176-8.
- Yang HR. How to approach feeding difficulties in young children. Korean J Pediatr 2017;60:379-84. [Crossref] [PubMed]
- Rastam M. Eating disturbances in autism spectrum disorders with focus on adolescent and adult years. Clin Neuropsych 2008;5:31-42.
- Sharp WG, Berry RC, McCracken C, et al. Feeding problems and nutrient intake in children with autism spectrum disorders: a meta-analysis and comprehensive review of the literature. J Autism Dev Disord 2013;43:2159-73. [Crossref] [PubMed]
- Devlin S, Healy O, Leader G, et al. The analysis and treatment of problem behavior evoked by auditory stimulation. Res Autism Spectr Disord 2008;2:671-80.
- Williams S, Leader G, Mannion A, et al. An investigation of anxiety in children and adolescents with autism spectrum disorder. Res Autism Spectr Disord 2015;10:30-40.
- Leader G, Mannion A. Challenging behaviors. Handbook of assessment and diagnosis of autism spectrum disorder; 2016:209-32.
- Francis K, Mannion A, Leader G. The assessment and treatment of toileting difficulties in individuals with autism spectrum disorder and other developmental disabilities. Rev J Autism Dev Disord 2017;4:190-204.
- Mannion A, Leader G. Comorbidity in autism spectrum disorder: A literature review. Res Autism Spectr Disord 2013;7:1595-616.
- Schreck KA, Williams K, Smith AF. A comparison of eating behaviors between children with and without autism. J Autism Dev Disord 2004;34:433-8. [Crossref] [PubMed]
- Seiverling L, Towle P, Hendy HM, et al. Prevalence of feeding problems in young children with and without autism spectrum disorder: A chart review study. J Early Interv 2018;40:335-46.
- Ahearn WH. Why does my son only eat macaroni and cheese? Dealing with feeding problems in children with autism. In: Maurice C, Foxx R, Green G, editors. Making a difference: Behavioral intervention for autism. Austin: Pro-ed; 2001.
- Herbert MR, Buckley JA. Autism and dietary therapy: case report and review of the literature. J Child Neurol 2013;28:975-82. [Crossref] [PubMed]
- Latif A, Heinz P, Cook R. Iron deficiency in autism and Asperger syndrome. Autism 2002;6:103-14. [Crossref] [PubMed]
- Emond A, Emmett P, Steer C, et al. Feeding symptoms, dietary patterns, and growth in young children with autism spectrum disorders. Pediatrics 2010;126:e337-42. [Crossref] [PubMed]
- Egan AM, Dreyer ML, Odar CC, et al. Obesity in young children with autism spectrum disorders: prevalence and associated factors. Child Obes 2013;9:125-31. [Crossref] [PubMed]
- Matson JL, Fodstad JC. The treatment of food selectivity and other feeding problems in children with autism spectrum disorders. Res Autism Spectr Disord 2009;3:455-61.
- Mannion A, Leader G, Healy O. An investigation of comorbid psychological disorders, sleep problems, gastrointestinal symptoms and epilepsy in children and adolescents with autism spectrum disorder. Res Autism Spectr Disord 2013;7:35-42.
- Wang LW, Tancredi DJ, Thomas DW. The prevalence of gastrointestinal problems in children across the United States with autism spectrum disorders from families with multiple affected members. J Dev Behav Pediatr 2011;32:351-60. [Crossref] [PubMed]
- Field D, Garland M, Williams K. Correlates of specific childhood feeding problems. J Paediatr Child Health 2003;39:299-304. [Crossref] [PubMed]
- Özen G, Güneş B, Yalçın S, et al. Mother-child pairs' eating and feeding behaviours in two different nutritional status from two distinct provinces. BMC Pediatr 2024;24:25. [Crossref] [PubMed]
- Rivell A, Mattson MP. Intergenerational Metabolic Syndrome and Neuronal Network Hyperexcitability in Autism. Trends Neurosci 2019;42:709-26. [Crossref] [PubMed]
- Zeybek SG, Yurttagul M. Nutrient status, diet quality and growth parameters of children with autism spectrum disorder in Northern Cyprus. Progress in Nutrition 2020;
- Namjoo I, Alavi Naeini A, Najafi M, et al. The Relationship Between Antioxidants and Inflammation in Children With Attention Deficit Hyperactivity Disorder. Basic Clin Neurosci 2020;11:313-21. [Crossref] [PubMed]
- Nadon G, Feldman D, Gisel E. Feeding issues associated with autism spectrum disorders. In: Recent Advances in Autism Spectrum Disorders-Volume I. IntechOpen; 2013.
- Curtin C, Must A, Phillips S, et al. The healthy weight research network: a research agenda to promote healthy weight among youth with autism spectrum disorder and other developmental disabilities. Pediatr Obes 2017;12:e6-9. [Crossref] [PubMed]
- Dawson G, Rogers S, Munson J, et al. Randomized, controlled trial of an intervention for toddlers with autism: the Early Start Denver Model. Pediatrics 2010;125:e17-23. [Crossref] [PubMed]
- Masi A, DeMayo MM, Glozier N, et al. An Overview of Autism Spectrum Disorder, Heterogeneity and Treatment Options. Neurosci Bull 2017;33:183-93. [Crossref] [PubMed]
- Hill AP, Zuckerman KE, Fombonne E. Obesity and Autism. Pediatrics 2015;136:1051-61. [Crossref] [PubMed]
- Twachtman-Reilly J, Amaral SC, Zebrowski PP. Addressing feeding disorders in children on the autism spectrum in school-based settings: Physiological and behavioral issues. Language, Speech, and Hearing Services in Schools 2008; [Crossref]
- Leekam SR, Nieto C, Libby SJ, et al. Describing the sensory abnormalities of children and adults with autism. J Autism Dev Disord 2007;37:894-910. [Crossref] [PubMed]
- Laud RB, Girolami PA, Boscoe JH, et al. Treatment outcomes for severe feeding problems in children with autism spectrum disorder. Behav Modif 2009;33:520-36. [Crossref] [PubMed]
- Cermak SA, Curtin C, Bandini LG. Food selectivity and sensory sensitivity in children with autism spectrum disorders. J Am Diet Assoc 2010;110:238-46. [Crossref] [PubMed]
- Goldman SE, Surdyka K, Cuevas R, et al. Defining the sleep phenotype in children with autism. Dev Neuropsychol 2009;34:560-73. [Crossref] [PubMed]
- Żarnowska I, Chrapko B, Gwizda G, et al. Therapeutic use of carbohydrate-restricted diets in an autistic child; a case report of clinical and 18FDG PET findings. Metab Brain Dis 2018;33:1187-92. [Crossref] [PubMed]
- Napoli E, Dueñas N, Giulivi C. Potential therapeutic use of the ketogenic diet in autism spectrum disorders. Front Pediatr 2014;2:69. [Crossref] [PubMed]
- Cheng N, Rho JM, Masino SA. Metabolic Dysfunction Underlying Autism Spectrum Disorder and Potential Treatment Approaches. Front Mol Neurosci 2017;10:34. [Crossref] [PubMed]
- Kolachahi SA. Water-based training in combined with vitamin D supplementation improves lipid profile in children with ASD. Res Autism Spectr Disord 2020;76:101603.
- Błażewicz A, Szymańska I, Astel A, et al. Assessment of Changes over Time of Lipid Profile, C-Reactive Protein Level and Body Mass Index in Teenagers and Young Adults on Different Diets Belonging to Autism Spectrum Disorder. Nutrients 2020;12:2594. [Crossref] [PubMed]
- Toscano CVA, Carvalho HM, Ferreira JP. Exercise Effects for Children With Autism Spectrum Disorder: Metabolic Health, Autistic Traits, and Quality of Life. Percept Mot Skills 2018;125:126-46. [Crossref] [PubMed]
- Mazahery H, Conlon CA, Beck KL, et al. Inflammation (IL-1β) Modifies the Effect of Vitamin D and Omega-3 Long Chain Polyunsaturated Fatty Acids on Core Symptoms of Autism Spectrum Disorder-An Exploratory Pilot Study. Nutrients 2020;12:661. [Crossref] [PubMed]
- Adams JB, Audhya T, McDonough-Means S, et al. Effect of a vitamin/mineral supplement on children and adults with autism. BMC Pediatr 2011;11:111. [Crossref] [PubMed]
- Cruchet S, Lucero Y, Cornejo V. Truths, Myths and Needs of Special Diets: Attention-Deficit/Hyperactivity Disorder, Autism, Non-Celiac Gluten Sensitivity, and Vegetarianism. Ann Nutr Metab 2016;68:43-50. [Crossref] [PubMed]
- Zuckerman KE, Hill AP, Guion K, et al. Overweight and obesity: prevalence and correlates in a large clinical sample of children with autism spectrum disorder. J Autism Dev Disord 2014;44:1708-19. [Crossref] [PubMed]
- Jones RA, Downing K, Rinehart NJ, et al. Physical activity, sedentary behavior and their correlates in children with Autism Spectrum Disorder: A systematic review. PLoS One 2017;12:e0172482. [Crossref] [PubMed]
- Sekirov I, Russell SL, Antunes LC, et al. Gut microbiota in health and disease. Physiol Rev 2010;90:859-904. [Crossref] [PubMed]
- Grenham S, Clarke G, Cryan JF, et al. Brain-gut-microbe communication in health and disease. Front Physiol 2011;2:94. [Crossref] [PubMed]
- Olszak T, An D, Zeissig S, et al. Microbial exposure during early life has persistent effects on natural killer T cell function. Science 2012;336:489-93. [Crossref] [PubMed]
- Yatsunenko T, Rey FE, Manary MJ, et al. Human gut microbiome viewed across age and geography. Nature 2012;486:222-7. [Crossref] [PubMed]
- White MT, Sears CL. The microbial landscape of colorectal cancer. Nat Rev Microbiol 2024;22:240-54. [Crossref] [PubMed]
- Louis P, Hold GL, Flint HJ. The gut microbiota, bacterial metabolites and colorectal cancer. Nat Rev Microbiol 2014;12:661-72. [Crossref] [PubMed]
- Forsythe P, Bienenstock J, Kunze WA. Vagal pathways for microbiome-brain-gut axis communication. Adv Exp Med Biol 2014;817:115-33. [Crossref] [PubMed]
- Smith PM, Howitt MR, Panikov N, et al. The microbial metabolites, short-chain fatty acids, regulate colonic Treg cell homeostasis. Science 2013;341:569-73. [Crossref] [PubMed]
- Byrne CS, Chambers ES, Morrison DJ, et al. The role of short chain fatty acids in appetite regulation and energy homeostasis. Int J Obes (Lond) 2015;39:1331-8. [Crossref] [PubMed]
- MacFabe DF. Enteric short-chain fatty acids: microbial messengers of metabolism, mitochondria, and mind: implications in autism spectrum disorders. Microb Ecol Health Dis 2015;26:28177. [Crossref] [PubMed]
- Reigstad CS, Salmonson CE, Rainey JF 3rd, et al. Gut microbes promote colonic serotonin production through an effect of short-chain fatty acids on enterochromaffin cells. FASEB J 2015;29:1395-403. [Crossref] [PubMed]
- El-Ansary A, Al-Ayadhi L. Relative abundance of short chain and polyunsaturated fatty acids in propionic acid-induced autistic features in rat pups as potential markers in autism. Lipids Health Dis 2014;13:140. [Crossref] [PubMed]
- De Angelis M, Piccolo M, Vannini L, et al. Fecal microbiota and metabolome of children with autism and pervasive developmental disorder not otherwise specified. PLoS One 2013;8:e76993. [Crossref] [PubMed]
- Muller CL, Anacker AMJ, Veenstra-VanderWeele J. The serotonin system in autism spectrum disorder: From biomarker to animal models. Neuroscience 2016;321:24-41. [Crossref] [PubMed]
- Melke J, Goubran Botros H, Chaste P, et al. Abnormal melatonin synthesis in autism spectrum disorders. Mol Psychiatry 2008;13:90-8. [Crossref] [PubMed]
- Gabriele S, Sacco R, Persico AM. Blood serotonin levels in autism spectrum disorder: a systematic review and meta-analysis. Eur Neuropsychopharmacol 2014;24:919-29. [Crossref] [PubMed]
- Ibrahim SH, Voigt RG, Katusic SK, et al. Incidence of gastrointestinal symptoms in children with autism: a population-based study. Pediatrics 2009;124:680-6. [Crossref] [PubMed]
- Bischoff SC, Mailer R, Pabst O, et al. Role of serotonin in intestinal inflammation: knockout of serotonin reuptake transporter exacerbates 2,4,6-trinitrobenzene sulfonic acid colitis in mice. Am J Physiol Gastrointest Liver Physiol 2009;296:G685-95. [Crossref] [PubMed]
- Kraneveld AD, Szklany K, de Theije CG, et al. Gut-to-Brain Axis in Autism Spectrum Disorders: Central Role for the Microbiome. Int Rev Neurobiol 2016;131:263-87. [Crossref] [PubMed]
- Karhu E, Zukerman R, Eshraghi RS, et al. Nutritional interventions for autism spectrum disorder. Nutr Rev 2020;78:515-31. [Crossref] [PubMed]
- Cekici H, Sanlier N. Current nutritional approaches in managing autism spectrum disorder: A review. Nutr Neurosci 2019;22:145-55. [Crossref] [PubMed]
- Alzghoul L, Abdelhamid SS, Yanis AH, et al. The association between levels of inflammatory markers in autistic children compared to their unaffected siblings and unrelated healthy controls. Turk J Med Sci 2019;49:1047-53. [Crossref] [PubMed]
- Posnakidis G, Aphamis G, Giannaki CD, et al. High-Intensity Functional Training Improves Cardiorespiratory Fitness and Neuromuscular Performance Without Inflammation or Muscle Damage. J Strength Cond Res 2022;36:615-23. [Crossref] [PubMed]
- Ansari Kolachahi S, Hojjati Zidashti Z, Elmieh A, et al. Comparison of aquatic exercise program and vitamin D supplementation on vitamin D and hs-CRP serum levels in children with autism spectrum disorders. J Jahrom Univ Med Sci 2018;16:48-56.
- Shahrasfenghar A, Arabameri E, Daneshfar A, et al. The effect of aerobic exercise on motor skills and body composition of children with autism. J Health Care 2019;20:332-41.
- Dhaliwal KK, Orsso CE, Richard C, et al. Risk Factors for Unhealthy Weight Gain and Obesity among Children with Autism Spectrum Disorder. Int J Mol Sci 2019;20:3285. [Crossref] [PubMed]
- Cerqueira É, Marinho DA, Neiva HP, et al. Inflammatory effects of high and moderate intensity exercise—A systematic review. Front Physiol 2020;10:1550. [Crossref] [PubMed]
- Yarımkaya E, Esentürk OK. Promoting physical activity for children with autism spectrum disorders during Coronavirus outbreak: benefits, strategies, and examples. Int J Dev Disabil 2022;68:430-5. [Crossref] [PubMed]
- Ros E, Martínez-González MA, Estruch R, et al. Mediterranean diet and cardiovascular health: Teachings of the PREDIMED study. Adv Nutr 2014;5:330S-6S. [Crossref] [PubMed]
- Evangeliou A, Vlachonikolis I, Mihailidou H, et al. Application of a ketogenic diet in children with autistic behavior: pilot study. J Child Neurol 2003;18:113-8. [Crossref] [PubMed]
- Castro K, Faccioli LS, Baronio D, et al. Effect of a ketogenic diet on autism spectrum disorder: A systematic review. Res Autism Spectr Disord 2015;20:31-8.
- Castro K, Baronio D, Perry IS, et al. The effect of ketogenic diet in an animal model of autism induced by prenatal exposure to valproic acid. Nutr Neurosci 2017;20:343-50. [Crossref] [PubMed]
- Ruskin DN, Fortin JA, Bisnauth SN, et al. Ketogenic diets improve behaviors associated with autism spectrum disorder in a sex-specific manner in the EL mouse. Physiol Behav 2017;168:138-45. [Crossref] [PubMed]
- Lee RWY, Corley MJ, Pang A, et al. A modified ketogenic gluten-free diet with MCT improves behavior in children with autism spectrum disorder. Physiol Behav 2018;188:205-11. [Crossref] [PubMed]
- Mantis JG, Fritz CL, Marsh J, et al. Improvement in motor and exploratory behavior in Rett syndrome mice with restricted ketogenic and standard diets. Epilepsy Behav 2009;15:133-41. [Crossref] [PubMed]
- Frye RE, Sreenivasula S, Adams JB. Traditional and non-traditional treatments for autism spectrum disorder with seizures: an on-line survey. BMC Pediatr 2011;11:37. [Crossref] [PubMed]
- Ruskin DN, Svedova J, Cote JL, et al. Ketogenic diet improves core symptoms of autism in BTBR mice. PLoS One 2013;8:e65021. [Crossref] [PubMed]
- Ahn Y, Narous M, Tobias R, et al. The ketogenic diet modifies social and metabolic alterations identified in the prenatal valproic acid model of autism spectrum disorder. Dev Neurosci 2014;36:371-80. [Crossref] [PubMed]
- Verpeut JL, DiCicco-Bloom E, Bello NT. Ketogenic diet exposure during the juvenile period increases social behaviors and forebrain neural activation in adult Engrailed 2 null mice. Physiol Behav 2016;161:90-8. [Crossref] [PubMed]
- Smith J, Rho JM, Teskey GC. Ketogenic diet restores aberrant cortical motor maps and excitation-to-inhibition imbalance in the BTBR mouse model of autism spectrum disorder. Behav Brain Res 2016;304:67-70. [Crossref] [PubMed]
- Newell C, Bomhof MR, Reimer RA, et al. Ketogenic diet modifies the gut microbiota in a murine model of autism spectrum disorder. Mol Autism 2016;7:37. [Crossref] [PubMed]
- Mychasiuk R, Rho JM. Genetic modifications associated with ketogenic diet treatment in the BTBR(T+Tf/J) mouse model of autism spectrum disorder. Autism Res 2017;10:456-71. [Crossref] [PubMed]
- Ruskin DN, Murphy MI, Slade SL, et al. Ketogenic diet improves behaviors in a maternal immune activation model of autism spectrum disorder. PLoS One 2017;12:e0171643. [Crossref] [PubMed]
- Dai Y, Zhao Y, Tomi M, et al. Sex-Specific Life Course Changes in the Neuro-Metabolic Phenotype of Glut3 Null Heterozygous Mice: Ketogenic Diet Ameliorates Electroencephalographic Seizures and Improves Sociability. Endocrinology 2017;158:936-49. [Crossref] [PubMed]
- Doenyas C. Dietary interventions for autism spectrum disorder: New perspectives from the gut-brain axis. Physiol Behav 2018;194:577-82. [Crossref] [PubMed]
- Tomova A, Husarova V, Lakatosova S, et al. Gastrointestinal microbiota in children with autism in Slovakia. Physiol Behav 2015;138:179-87. [Crossref] [PubMed]
- Grossi E, Melli S, Dunca D, et al. Unexpected improvement in core autism spectrum disorder symptoms after long-term treatment with probiotics. SAGE Open Med Case Rep 2016;4:2050313X16666231.
- Shaaban SY, El Gendy YG, Mehanna NS, et al. The role of probiotics in children with autism spectrum disorder: A prospective, open-label study. Nutr Neurosci 2018;21:676-81. [Crossref] [PubMed]
- Pärtty A, Kalliomäki M, Wacklin P, et al. A possible link between early probiotic intervention and the risk of neuropsychiatric disorders later in childhood: a randomized trial. Pediatr Res 2015;77:823-8. [Crossref] [PubMed]
- Anderson RC, Cookson AL, McNabb WC, et al. Lactobacillus plantarum DSM 2648 is a potential probiotic that enhances intestinal barrier function. FEMS Microbiol Lett 2010;309:184-92. [Crossref] [PubMed]
- Doenyas C. Gut Microbiota, Inflammation, and Probiotics on Neural Development in Autism Spectrum Disorder. Neuroscience 2018;374:271-86. [Crossref] [PubMed]
- West R, Roberts E, Sichel LS, et al. Improvements in gastrointestinal symptoms among children with autism spectrum disorder receiving the Delpro® probiotic and immunomodulator formulation. J Prob Health 2013;1:1-6.
- Kantarcioglu AS, Kiraz N, Aydin A. Microbiota-Gut-Brain Axis: Yeast Species Isolated from Stool Samples of Children with Suspected or Diagnosed Autism Spectrum Disorders and In Vitro Susceptibility Against Nystatin and Fluconazole. Mycopathologia 2016;181:1-7. [Crossref] [PubMed]
- Iovene MR, Bombace F, Maresca R, et al. Intestinal Dysbiosis and Yeast Isolation in Stool of Subjects with Autism Spectrum Disorders. Mycopathologia 2017;182:349-63. [Crossref] [PubMed]
- Baldassarre ME, Palladino V, Amoruso A, et al. Rationale of Probiotic Supplementation during Pregnancy and Neonatal Period. Nutrients 2018;10:1693. [Crossref] [PubMed]
- Salvatore S, Pensabene L, Borrelli O, et al. Mind the gut: probiotics in paediatric neurogastroenterology. Benef Microbes 2018;9:883-98. [Crossref] [PubMed]
- Gottschall E. Digestion-gut-autism connection: the specific carbohydrate diet. Medical Veritas The Journal of Medical Truth 2004;1:261-71.
- Kushak RI, Winter HS, Buie TM, et al. Analysis of the Duodenal Microbiome in Autistic Individuals: Association With Carbohydrate Digestion. J Pediatr Gastroenterol Nutr 2017;64:e110-6. [Crossref] [PubMed]
- Strati F, Cavalieri D, Albanese D, et al. New evidences on the altered gut microbiota in autism spectrum disorders. Microbiome 2017;5:24. [Crossref] [PubMed]
- Barnhill K, Devlin M, Moreno HT, et al. Brief Report: Implementation of a Specific Carbohydrate Diet for a Child with Autism Spectrum Disorder and Fragile X Syndrome. J Autism Dev Disord 2020;50:1800-8. [Crossref] [PubMed]
- Guo M, Zhu J, Yang T, et al. Vitamin A improves the symptoms of autism spectrum disorders and decreases 5-hydroxytryptamine (5-HT): A pilot study. Brain Res Bull 2018;137:35-40. [Crossref] [PubMed]
- Liu X, Liu J, Xiong X, et al. Correlation between Nutrition and Symptoms: Nutritional Survey of Children with Autism Spectrum Disorder in Chongqing, China. Nutrients 2016;8:294. [Crossref] [PubMed]
- Gamliel M, Anderson KL, Ebstein RP, et al. The oxytocin-CD38-vitamin A axis in pregnant women involves both hypothalamic and placental regulation. J Matern Fetal Neonatal Med 2016;29:2685-90. [Crossref] [PubMed]
- Cheng B, Zhu J, Yang T, et al. Vitamin A deficiency exacerbates autism-like behaviors and abnormalities of the enteric nervous system in a valproic acid-induced rat model of autism. Neurotoxicology 2020;79:184-90. [Crossref] [PubMed]
- Lai X, Wu X, Hou N, et al. Vitamin A Deficiency Induces Autistic-Like Behaviors in Rats by Regulating the RARβ-CD38-Oxytocin Axis in the Hypothalamus. Mol Nutr Food Res 2018; [Crossref]
- Stewart PA, Hyman SL, Schmidt BL, et al. Dietary Supplementation in Children with Autism Spectrum Disorders: Common, Insufficient, and Excessive. J Acad Nutr Diet 2015;115:1237-48. [Crossref] [PubMed]
- Hyman SL, Stewart PA, Schmidt B, et al. Nutrient intake from food in children with autism. Pediatrics 2012;130:S145-53. [Crossref] [PubMed]
- Liu J, Liu X, Xiong XQ, et al. Effect of vitamin A supplementation on gut microbiota in children with autism spectrum disorders - a pilot study. BMC Microbiol 2017;17:204. [Crossref] [PubMed]
- Rafee Y, Burrell K, Cederna-Meko C. Lessons in early identification and treatment from a case of disabling vitamin C deficiency in a child with autism spectrum disorder. Int J Psychiatry Med 2019;54:64-73. [Crossref] [PubMed]
- Kinlin LM, Blanchard AC, Silver S, et al. Scurvy as a mimicker of osteomyelitis in a child with autism spectrum disorder. Int J Infect Dis 2018;69:99-102. [Crossref] [PubMed]
- Ma NS, Thompson C, Weston S. Brief Report: Scurvy as a Manifestation of Food Selectivity in Children with Autism. J Autism Dev Disord 2016;46:1464-70. [Crossref] [PubMed]
- Niwa T, Aida N, Tanaka Y, et al. Scurvy in a child with autism: magnetic resonance imaging and pathological findings. J Pediatr Hematol Oncol 2012;34:484-7. [Crossref] [PubMed]
- Harknett KM, Hussain SK, Rogers MK, et al. Scurvy mimicking osteomyelitis: case report and review of the literature. Clin Pediatr (Phila) 2014;53:995-9. [Crossref] [PubMed]
- Malhi P, Venkatesh L, Bharti B, et al. Feeding Problems and Nutrient Intake in Children with and without Autism: A Comparative Study. Indian J Pediatr 2017;84:283-8. [Crossref] [PubMed]
- Marí-Bauset S, Llopis-González A, Zazpe I, et al. Comparison of nutritional status between children with autism spectrum disorder and typically developing children in the Mediterranean Region (Valencia, Spain). Autism 2017;21:310-22. [Crossref] [PubMed]
- Amberg N, Laukoter S, Hippenmeyer S. Epigenetic cues modulating the generation of cell-type diversity in the cerebral cortex. J Neurochem 2019;149:12-26. [Crossref] [PubMed]
- Moody L, Chen H, Pan YX. Early-Life Nutritional Programming of Cognition-The Fundamental Role of Epigenetic Mechanisms in Mediating the Relation between Early-Life Environment and Learning and Memory Process. Adv Nutr 2017;8:337-50. [Crossref] [PubMed]
- Gherasim C, Lofgren M, Banerjee R. Navigating the B(12) road: assimilation, delivery, and disorders of cobalamin. J Biol Chem 2013;288:13186-93. [Crossref] [PubMed]
- Zhang H, Zhuang XD, Meng FH, et al. Calcitriol prevents peripheral RSC96 Schwann neural cells from high glucose & methylglyoxal-induced injury through restoration of CBS/H2S expression. Neurochem Int 2016;92:49-57. [Crossref] [PubMed]
- Lee M, Schwab C, Yu S, et al. Astrocytes produce the antiinflammatory and neuroprotective agent hydrogen sulfide. Neurobiol Aging 2009;30:1523-34. [Crossref] [PubMed]
- Zhang Y, Hodgson NW, Trivedi MS, et al. Decreased Brain Levels of Vitamin B12 in Aging, Autism and Schizophrenia. PLoS One 2016;11:e0146797. [Crossref] [PubMed]
- Huang CY, Yao WF, Wu WG, et al. Endogenous CSE/H2S system mediates TNF-α-induced insulin resistance in 3T3-L1 adipocytes. Cell Biochem Funct 2013;31:468-75. [Crossref] [PubMed]
- Chez MG, Dowling T, Patel PB, et al. Elevation of tumor necrosis factor-alpha in cerebrospinal fluid of autistic children. Pediatr Neurol 2007;36:361-5. [Crossref] [PubMed]
- Li YJ, Li YM, Xiang DX. Supplement intervention associated with nutritional deficiencies in autism spectrum disorders: a systematic review. Eur J Nutr 2018;57:2571-82. [Crossref] [PubMed]
- Murza KA, Pavelko SL, Malani MD, et al. Vitamin B6-magnesium treatment for autism: the current status of the research. Magnes Res 2010;23:115-7. [Crossref] [PubMed]
- Mousain-Bosc M, Siatka C, Bali JP. Magnesium, hyperactivity and autism in children. In: Vink R, Nechifor M, eds. Magnesium in the Central Nervous System. Adelaide (AU): University of Adelaide Press; 2011.
- Obara T, Ishikuro M, Tamiya G, et al. Potential identification of vitamin B6 responsiveness in autism spectrum disorder utilizing phenotype variables and machine learning methods. Sci Rep 2018;8:14840. [Crossref] [PubMed]
- Shabo Y, Yagil R. Etiology of autism and camel milk as therapy. Int J Disabil Hum Dev 2005;4:67-70.
- Abu-Lehia IH. Composition of camel milk. Milchwissenschaft 1987;42:368-71.
- Shabo Y, Barzel R, Margoulis M, et al. Camel milk for food allergies in children. Isr Med Assoc J 2005;7:796-8.
- Beg OU, von Bahr-Lindström H, Zaidi ZH, et al. Characterization of a camel milk protein rich in proline identifies a new beta-casein fragment. Regul Pept 1986;15:55-61. [Crossref] [PubMed]
- Zagorski OA, Maman AL, Yaffe A, et al. Insulin in milk-a comparative study. Int J Anim Sci 1998;13:241-4.
- Knivsberg AM, Reichelt KL, Høien T, et al. A randomised, controlled study of dietary intervention in autistic syndromes. Nutr Neurosci 2002;5:251-61. [Crossref] [PubMed]
- Al-Ayadhi LY, Elamin NE. Camel Milk as a Potential Therapy as an Antioxidant in Autism Spectrum Disorder (ASD). Evid Based Complement Alternat Med 2013;2013:602834. [Crossref] [PubMed]
- Hasen IJ. The Importance of Camel Milk and Its Health Benefits. Technium BioChemMed 2024;8:91-103.