Long-term changes in the gut microbiota before and after bismuth quadruple therapy or concomitant therapy in children with peptic ulcers receiving Helicobacter pylori eradication
Highlight box
Key findings
• Both bismuth quadruple therapy and concomitant therapy affect gut microbiota in the short term, but are safe in the long term.
What is known and what is new?
• Both antibiotics and proton pump inhibitors can affect gut microbiota.
• Bismuth quadruple therapy and concomitant therapy do not produce serious long-term effects on the gut microbiota in pediatric and peptic ulcer conditions.
What is the implication, and what should change now?
• For children with peptic ulcer caused by Helicobacter pylori infection, the two therapies can be safely selected.
Introduction
Helicobacter pylori (H. pylori) is a gram-negative spiral bacterium that colonizes the gastric mucosa, commonly causing chronic infection. Ninety-five percent of duodenal ulcers and 70% of gastric ulcers are attributed to Helicobacter pylori (1). Elimination of H. pylori can promote ulcer healing and is beneficial for preventing recurrence (2). The latest guideline of ESPGHAN/NASPGHAN [2023] strongly recommends that testing for H. pylori be performed in children with gastric or duodenal ulcers and/or erosions (3).
Since 1996, the recommendation of standard triple therapy [STT, a proton-pump inhibitor (PPI) and two antibiotics] as the standard first-line eradication therapy has been proposed (4). However, due to increasing resistance to clarithromycin, the efficacy of this combination is declining globally. Hence, novel regimens, such as bismuth quadruple therapy and concomitant therapy, have been developed. It also recommends that if H. pylori clarithromycin susceptibility is unknown, bismuth-containing quadruple therapy of bismuth, PPI, amoxicillin, metronidazole for 14days is suggested (3). In the guideline of ESPGHAN/NASPGHAN (update 2016) referred that concomitant therapy (PPI-amoxicillin-metronidazole-clarithromycin) for 14 days can also be used when clarithromycin susceptibility is unknown (5).
Most H. pylori eradication regimens involve the use of multiple antibiotics as well as auxiliary drugs, including PPIs and bismuth salts. Broad-spectrum antibiotic administration may cause side effects such as an imbalance in the gut microbiota composition by affecting the target strains and the commensal microbiota in the digestive tract. The auxiliary drug PPI weakens the gastric acid barrier and may cause dysbiosis by altering the gastrointestinal pH and delaying the gastric emptying rate. The direct effects on the survival environment of enteric pathogens lead to changes in the composition of the gut microbiota (6). Therefore, regardless of which regimen is used, concerns about potentially negative effects on the gut microbiota have been raised. Recently, several studies have reported that bacterial diversity significantly decreases shortly after STT (7,8). Nevertheless, studies addressing the long-term effects of H. pylori eradication therapies involving the gut microbiota are limited. We identified ten publications that used high-throughput sequencing to concern long-term changes in the gut microbiota related to H. pylori eradication (7,9-17). Most studies focused on adult patients (n=8). Although there have been reports about microbiota involvement in peptic ulceration, for children with peptic ulcers, the impacts of bismuth quadruple therapy and concomitant therapy on fecal ecology remain largely unknown. Comparing with adults, antibiotics like levofloxacin, furazolidone and rifabutin can’t be used in young children. As bismuth-containing quadruple therapy and concomitant therapy are both quadruple therapies, and both widely used in pediatric clinical practice (3,18). We conducted a 16S ribosomal RNA (rRNA)-based analysis to investigate the impacts of these two therapies on the gut microbiota 12 months post-eradication. We present this article in accordance with the STROBE reporting checklist (available at https://tp.amegroups.com/article/view/10.21037/tp-24-207/rc).
Methods
Patients and study design
This was a prospective, cross-sectional, comparative study. We conducted the study at the Children’s Hospital of Fudan University from September 2017 to December 2020. The study was conducted in accordance with the Declaration of Helsinki (as revised in 2013). The study was approved by the Ethics Committee of the Children’s Hospital of Fudan [No. (2017) 186], and informed consent was taken from all the patients. Prior to study participation, the guardians of all the recruited patients signed written informed consent forms.
The subjects included in this study were 6- to 18-year-old patients with peptic ulcer disease (PUD) who were diagnosed with active H. pylori infection by gastroscopy. Only positive cultures or positive cultures on both the rapid urease test (RUT) and histology were used to diagnose active H. pylori infection. The exclusion criteria were as follows: (I) had previously received H. pylori eradication therapy; (II) had severe concurrent diseases; (III) had contraindications or previous allergic reactions to the study drugs; and (IV) had used specific drugs or bacteriological products, including antibiotics, PPIs, histamine-2 receptor antagonists, bismuth or probiotics, within the previous 4 weeks. The healthy control group (HC group) included children who had a negative urea breath test (UBT), who were without digestive or other systemic diseases, and who were taking no drugs within 4 weeks. According to the physician’s decision, eligible patients were assigned to receive bismuth-containing quadruple therapy (BT group) or concomitant therapy (CT group) for 14 days. The following drugs were used in this study: omeprazole [(AstraZeneca Pharmaceutical Company, London, UK), 1.0 mg/(kg·d), once or twice a day, maximum daily dose 40 mg]; amoxicillin [(Zhuhai United Laboratories, Zhuhai, China), 50 mg/(kg·d), three times or four times a day, maximum daily dose 2,000 mg]; metronidazole [(Shanghai Xinyi Wanxiang Pharmaceutical Industry Company, Shanghai, China), 20 mg/(kg·d), two or three times a day, maximum daily dose 1,000 mg]; clarithromycin [(Abbott Laboratories Limited), 15–20 mg/(kg·d), twice a day, maximum daily dose 1,000 mg]; and bismuth potassium citrate [(Livzon Pharmaceutical Group, Zhuhai, China, maximum daily dose 330 mg), elemental bismuth, 6–8 mg/(kg·d), twice or three times a day]. Patients in the BT group took omeprazole, amoxicillin, metronidazole and bismuth potassium citrate. Patients in the CT group were given omeprazole and three antibiotics (amoxicillin, clarithromycin and metronidazole). Patients continued to use PPIs for 4 weeks and then underwent gastroscopy to assess ulcer healing after eradication therapy. At least 8 weeks after treatment, eradication success was confirmed. We performed 4 visits for patients in the study group and 1 visit for patients in the HC group. For H. pylori-positive patients, fecal samples at baseline (week 0), at the end of therapy (week 2), at 6 weeks post eradication (week 6), and at 1 year post eradication (week 52) were collected and immediately preserved at −80 ℃ until DNA extraction.
DNA extraction, polymerase chain reaction (PCR) amplification and MiSeq sequencing
As described previously (15), we used the same method. Briefly, the FastDNA SPIN Kit for Soil (MP Biomedicals, USA) was first used to extract DNA, and the primers 338F (5'-ACTCCTACGGGAGGCAGCAG-3') and 806R (5'-GGACTACHVGGGTWTCTAAT-3') were used to amplify the 16S rRNA gene. Purified amplicons were sequenced on an Illumina MiSeq platform (Illumina, San Diego, USA).
Taxonomy determination
Raw fastq files were quality-filtered by Trimmomatic and merged by FLASH to ensure that the average quality score was <20, with a mismatch of no more than 2 bp, and reads containing ambiguous bases were removed. Operational taxonomic units (OTUs) were clustered with 97% similarity using UPARSE (version 7.1 http://drive5.com/uparse/). We used the RDP Classifier algorithm (http://rdp.cme.msu.edu/) to analyze the taxonomy of the 16S rRNA gene sequence against Silva (SSU128) using a confidence threshold of 0.8.
Identification of differentially abundant OTUs
Linear discriminant analysis effect size (LEfSe) was applied to identify differential taxa between baseline and 2 weeks after therapy. The effect size threshold was 4.
Statistical analysis
We used Stata 11 (Stata Corporation, College Station, TX, USA) to analyze the data. Baseline continuous data were shown as the mean ± standard deviation (SD). Depending on the distribution, we used one-way ANOVA or the Kruskal-Wallis test to compare these data among different groups. Categorical data were presented as percentages, and the Chi-squared test or Fisher’s exact test was used for comparisons. Mothur software was used to calculate community diversity parameters (Shannon index) and community richness parameters (Chao 1). At different time points in certain groups or between different groups at the same time point, we used the Wilcoxon rank-sum test and Kruskal‒Wallis test to compare among groups. Considering the phylogenetic tree and thus phylogenetic distances between community members, principal coordinate analysis (PCoA) based on weighted UniFrac distance metrics was used to determine beta diversity, and the microbial communities were compared between the study groups. Statistical significance was calculated by ANOSIM. To compare the differences in the relative abundances of bacterial taxa within and between groups, we used the Wilcoxon rank-sum test and Kruskal-Wallis test. P values were adjusted by the false discovery rate (FDR). A two-tailed P value <0.05 was considered to indicate statistical significance.
Results
Characteristics of the subjects
In total, 42 patients with PUD were enrolled. Twenty-three patients in the BT group and 19 patients in the CT group were recruited. Twenty-three participants composed the HC group. The first (baseline), the second (immediately after eradication) and the third (6 weeks post-therapy) fecal sample collection were completed for all patients. In the BT group, 17 patients (73.9%), and in the CT group, 14 patients (73.7%) completed the fourth (12 months post-therapy) stool sample collection. Eventually, 180 stool samples were subjected to microbiota analysis (Figure 1). The baseline characteristics of all the enrolled patients and healthy controls are shown in Table S1. Compared with the negative controls, there was a greater age and greater frequency of male subjects in each of the two anti-H. pylori treatment groups. There was no significant difference in body mass index among the BT, CT and HC groups (P=0.98). We obtained 9,327,861 quality-filtered reads from all the fecal samples, with an average of 51,821±10,792 reads per sample.
Diversity analysis before and after H. pylori eradication
For alpha diversity estimation, the Shannon index, which represents community diversity, and the Chao 1 index, which represents community richness, were used for measurement and comparison at the OTU level (Table S2). Comparisons of alpha diversity among different time points are shown in Figure 2. Compared with those at baseline, both indices of alpha diversity (Shannon and Chao 1 indices) were markedly lower at week 2 (P<0.001, P<0.001) and week 6 (P<0.001, P<0.001) but recovered at week 52 (P=0.15, P=0.26) after bismuth quadruple therapy (Figure 2A,2B). PCoA generated from weighted UniFrac distance metrics was used to further evaluate differences in microbial community structures. Significant differences were shown at week 2 (R=0.5162, P=0.001) compared with baseline. However, the microbial community structures were restored at week 6 (R=0.0025, P=0.42) and week 52 (R=0.0079, P=0.47) (Figure 2C).
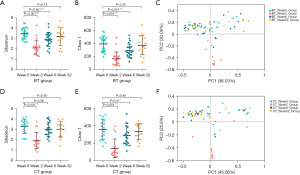
Compared with that at baseline, the alpha diversity of CT group at week 2 markedly decreased (P<0.001, P<0.001). At week 6, after concomitant therapy, the Shannon index returned to the basal state (P=0.26), but the Chao 1 index did not (P=0.01). Two alpha diversity indices were restored at week 52 (P=0.46, P=0.46) (Figure 2D,2E). Compared with those at baseline, significant differences in the fecal microbiota structure were observed at week 2 (R=0.4248, P=0.001). It recovered at week 6 (R=0.0265, P=0.16) and week 52 (R=0.0123, P=0.51) (Figure 2F).
Compositional analysis before and after H. pylori eradication
The relative abundances of different phyla (mean relative abundance >1%) were compared before and after H. pylori eradication. The composition of the gut microbiota could be significantly disturbed by H. pylori eradication. Table S3 shows a detailed description of these changes. The most dominant phyla with relative abundances >5% were Firmicutes and Bacteroidetes in the BT and CT groups at baseline, accounting for 93.3% and 92.1%, respectively, of the total bacteria. Compared with those at baseline in the BT group, at the end of eradication, the relative abundances of Firmicutes (47.37%±18.33% vs. 19.42%±18.60%, P<0.001), Bacteroidetes (45.90%±19.62% vs. 10.20%±19.33%, P<0.001), Actinobacteria (3.21%±5.06% vs. 2.88%±7.67%, P=0.03) and Fusobacteria (0.28%±0.80% vs. 0.14%±0.55%, P=0.048) significantly decreased, while that of Proteobacteria (2.55%±2.44% vs. 67.24%±32.78%, P<0.001) increased (Figure 3A). Compared with those at baseline in the CT group, at the end of eradication, the relative abundances of Firmicutes (44.69%±18.84% vs. 17.25±14.43%, P<0.001), Bacteroidetes (47.41%±18.97% vs. 22.02%±29.82%, P=0.02) and Fusobacteria (2.03±7.33% vs. 4.94E−4%±2.15E−3%, P=0.02) significantly decreased, while those of Proteobacteria (2.60%±2.87% vs. 57.83%±34.19%, P<0.001) and Actinobacteria (2.50±2.68% vs. 2.73±5.74%, P=0.04) increased (Figure 3B). At week 6, the relative abundance of Proteobacteria (P=0.02) in the BT group and Actinobacteria (P=0.01) in the CT group had not yet returned to baseline (Figure 3A,3B). In both the BT group and CT group, the relative abundance of phyla at the 1-year follow-up was comparable to that at baseline.
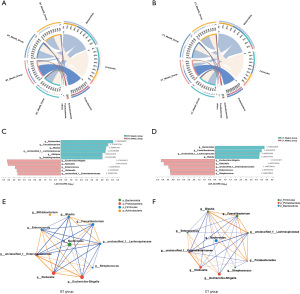
Next, we investigated the dynamic changes in bacterial genera. In the BT group, 5 bacterial taxa were enriched, while 6 bacterial taxa were depleted two weeks after therapy compared to the baseline. The enriched bacterial taxa included Escherichia-Shigella, Klebsiella, Enterococcus, Veillonella, and Enterobacteriaceae, while the depleted taxa included Bacteroides, Faecalibacterium, Blautia, Lachnospiraceae, Alistipes, and Subdoligranulum (Figure 3C). In the CT group, 5 taxa were increased, and 4 taxa were decreased. The increased taxa included Escherichia-Shigella, Klebsiella, Enterobacteriaceae, Enterococcus, and Streptococcus, and the decreased taxa included Bacteroides, Faecalibacterium, Lachnospiraceae and Blautia (Figure 3D). According to the genera abundance before and 2 weeks after treatment, interactions among the top 10 genera were further explored by microbial network analysis with R≥0.5 and P<0.05. Faecalibacterium was positively correlated with Bacteroides and Blautia, and Enterococcus was positively correlated with Escherichia-Shigella and Klebsiella in both the anti-H. pylori treatment groups. In contrast, the abundance of Faecalibacterium was negatively correlated with the abundances of Enterococcus, Escherichia-Shigella and Klebsiella (Figure 3E,3F).
Diversity analysis among the BT, CT and HC groups at certain time points
Taxonomic analysis of the gut microbiota to explore microbiota composition and diversity alterations was also performed at the same time points. Prior to treatment, there was no significant difference in the Shannon or Chao 1 index among these three groups (P>0.05, Table S4, Figure 4A,4B). Immediately after eradication, these two indices were significantly lower in the BT group (P<0.001, P<0.001) and CT group (P<0.001, P<0.001) than in the HC group (Figure 4C,4D). No significant difference was found between the two treatment groups (all P>0.05). Although there was a trend for the alpha diversity to increase with time, the two indices in the BT group (P=0.02, P=0.008) and the Chao 1 index (P=0.02) in the CT group were still lower than those in the healthy control group at week 6 (Figure 4E,4F). At the 1-year time point, the alpha diversity of the BT and CT groups was not significantly different from that of the HC group (all P>0.05) (Figure 4G,4H).
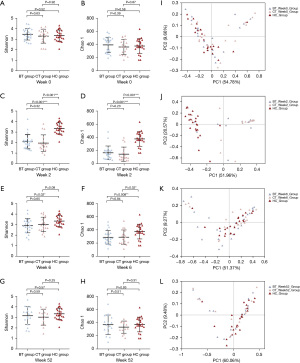
We used PCoA to analyze the structure of the gut microbiota. No significant differences were found among the three groups at the beginning of this study (R=0.0132, P=0.22, Figure 4I). At week 2, both PCoA and ANOSIM showed significant differences among the three groups (R=0.4156, P=0.001; Figure 4J). They reverted at week 6 (R=0.0173, P=0.18; Figure 4K) and remained there for one year (R=0.0427, P=0.11; Figure 4L).
Compositional analysis among the BT, CT and HC groups at certain time points
The relative abundances of the top 5 different phyla and the top 20 genera among these three groups at each time point were compared further (Tables S5,S6). No significant difference was found at the phylum level among the three groups before therapy (all P>0.05) (Figure 5A). Significant differences were observed in the relative abundances of Proteobacteria (P<0.001), Bacteroidetes (P<0.001), Firmicutes (P<0.001), Actinobacteria (P=0.007) and Desulfobacterota (P<0.001) among the three groups at week 2 (Figure 5B), and no significant difference was found between the two treatment groups (all P>0.05). In subsequent follow-up, significant differences were detected in the relative abundance of Actinobacteria at week 6 (P=0.007) (Figure 5C) and Proteobacteria at week 52 (P=0.02) (Figure 5D).
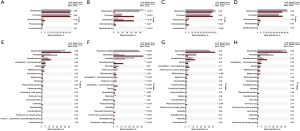
At the genus level, no significant differences were found among the three groups before treatment (all P>0.05, Figure 5E). At week 2, there were significant differences in the relative abundance of 90% (18/20) of the bacterial genera (Figure 5F). Among the top 20 bacterial genera, the relative abundances between the BT and CT groups were not significantly different at week 2. At week 6, 15% (3/20) of the bacterial genera exhibited significant differences in relative abundance (Figure 5G). At week 52, only 10% (2/20) of the bacterial genera existed differences (Figure 5H). The change trends were similar in both the BT and CT groups at each follow-up time point at both the phylum and genus levels.
Discussion
In a previous study, our results showed that, in children with H. pylori-associated gastritis, triple therapy, sequential therapy, bismuth quadruple therapy and concomitant therapy can all result in transient gut microbiota dysbiosis, and at 1 year post-eradication, the gut microbiota almost returns to the pre-eradication level. This may indicate the long-term safety of H. pylori therapy. However, among these regimens, the speed and extent of restoration of the gut microbiota are different (7). Because more than 70% of peptic ulcers are associated with H. pylori infection, few reports have investigated changes in the microbiota of PUD patients receiving H. pylori therapy. Current evidence indicates that H. pylori infection does not cause symptoms in children in the absence of PUD and/or erosions. So we pay special attention to PUD patients (3).
However, this study revealed that immediately after eradication, the alpha diversity (Shannon and Chao 1 indices) was strikingly reduced in the BT and CT groups. Although longitudinal analysis revealed a sustained increase in alpha diversity, the alpha diversity indices in both the BT and CT groups completely recovered until week 52. These results are in accordance with our previous results in patients with gastritis (7).
In the healthy gut, the dominant phyla Bacteroidetes and Firmicutes make up >90% of the microbiota, while Proteobacteria and Actinobacteria are present as minor players. Among these four phyla, the phylum Proteobacteria is the most unstable over time. Various studies have reported an increase in Proteobacteria in the stool after H. pylori infection (11,19). Our findings also showed that the high proportions of Firmicutes and Bacteroidetes were soon replaced by Proteobacteria during the 2 weeks of H. pylori eradication. This may be because antibiotics such as amoxicillin, clarithromycin and metronidazole have limited ability to inhibit Proteobacteria, but they can also efficiently inhibit other commensal bacteria. A high abundance of Proteobacteria has been shown to be associated with several intestinal disorders, such as inflammatory bowel disease and necrotizing enterocolitis (20,21). A bloom of Proteobacteria in the gut has been proposed as a microbial signature of gut dysbiosis representing an unstable microbial community structure (22).
The relative abundances of Escherichia-Shigella, Klebsiella, Enterococcus and Enterobacteriaceae increased; however, the abundances of Bacteroides, Faecalibacterium, Lachnospiraceae and Blautia decreased in the BT and CT groups at 2 weeks after therapy. Although some researches think these bacterial genera maybe related to gut inflammation, only specific species would cause these results. Like Bacteroides spp. are known to maintain eubiosis in the human gastrointestinal tract, however, some species are opportunistic pathogen (23). In this study we didn’t analyse the abundance at specie-level. So we can’t definitely hypothesize the effects of changes in genus-level is positive or negative.
In this study, H. pylori-negative asymptomatic children were enrolled in the healthy control group. We compared alpha diversity and beta diversity between H. pylori-positive patients (including the BT group and CT group) and H. pylori-negative healthy control children. We found no significant difference at baseline, which is in accordance with the findings of Gao et al. (24). In contrast, in Cornejo-Pareja et al.’s research, a lower diversity and significant alterations in the microbiota composition were detected in patients with H. pylori infection (25). Such divergences may be caused by different variables, such as age, diet, lifestyle and age at infection onset. A larger sample is needed to validate the baseline results.
There were some limitations in this study. Only 73.8% of patients completed this 1-year follow-up. Additionally, the 16S rRNA method has limitations at the species level. To overcome these limitations, further in-depth sequencing, such as whole-genome shotgun sequencing, is needed. Diet was another important influencing factor. However it was a single center study, most patients came from Shanghai and around area. Dietary factors are unlikely to make a big difference. So we didn’t analyse it. With the population mobility and diversification of diet, we still need to know the birthplace and dietary preferences of the children in future studies. To the best of our knowledge, this is the first prospective trial investigating the short-term and long-term effects of bismuth-containing quadruple therapy and concomitant therapy on the gut microbiota in PUD patients. A self-comparison study before and after treatment was performed, which can effectively control for individual differences and complex confounding factors.
Conclusions
This study revealed that bismuth quadruple therapy and concomitant therapy can cause transient changes of the gut microbiota in Chinese children, and at one year post-eradication, these changes almost completely returned to the pre-eradication level, which indicated that H. pylori therapy may have long-term safety in PUD patients.
Acknowledgments
We would like to express our sincere gratitude to all the patients and their families who participated in this study and the Children’s Hospital of Fudan University staff who assisted with sample collection.
Funding: None.
Footnote
Reporting Checklist: The authors have completed the STROBE reporting checklist. Available at https://tp.amegroups.com/article/view/10.21037/tp-24-207/rc
Data Sharing Statement: Available at https://tp.amegroups.com/article/view/10.21037/tp-24-207/dss
Peer Review File: Available at https://tp.amegroups.com/article/view/10.21037/tp-24-207/prf
Conflicts of Interest: All authors have completed the ICMJE uniform disclosure form (available at https://tp.amegroups.com/article/view/10.21037/tp-24-207/coif). The authors have no conflicts of interest to declare.
Ethical Statement: The authors are accountable for all aspects of the work in ensuring that questions related to the accuracy or integrity of any part of the work are appropriately investigated and resolved. The study was conducted in accordance with the Declaration of Helsinki (as revised in 2013). The study was approved by the Ethics Committee of the Children’s Hospital of Fudan [No. (2017) 186], and informed consent was taken from all the patients.
Open Access Statement: This is an Open Access article distributed in accordance with the Creative Commons Attribution-NonCommercial-NoDerivs 4.0 International License (CC BY-NC-ND 4.0), which permits the non-commercial replication and distribution of the article with the strict proviso that no changes or edits are made and the original work is properly cited (including links to both the formal publication through the relevant DOI and the license). See: https://creativecommons.org/licenses/by-nc-nd/4.0/.
References
- Peitz U, Leodolter A, Wex T, et al. Diagnostics of Helicobacter pylori infection in patients with peptic ulcer bleeding. Z Gastroenterol 2004;42:141-6. [Crossref] [PubMed]
- Ford AC, Gurusamy KS, Delaney B, et al. Eradication therapy for peptic ulcer disease in Helicobacter pylori-positive people. Cochrane Database Syst Rev 2016;4:CD003840. [Crossref] [PubMed]
- Homan M, Jones NL, Bontems P, et al. Updated joint ESPGHAN/NASPGHAN guidelines for management of Helicobacter pylori infection in children and adolescents (2023). J Pediatr Gastroenterol Nutr 2024;79:758-85. [Crossref] [PubMed]
- Current European concepts in the management of Helicobacter pylori infection. The Maastricht Consensus Report. European Helicobacter Pylori Study Group. Gut 1997;41:8-13. [Crossref] [PubMed]
- Jones NL, Koletzko S, Goodman K, et al. Joint ESPGHAN/NASPGHAN Guidelines for the Management of Helicobacter pylori in Children and Adolescents (Update 2016). J Pediatr Gastroenterol Nutr 2017;64:991-1003. [Crossref] [PubMed]
- Jackson MA, Goodrich JK, Maxan ME, et al. Proton pump inhibitors alter the composition of the gut microbiota. Gut 2016;65:749-56. [Crossref] [PubMed]
- Zhou Y, Ye Z, Wang Y, et al. Long-term changes in the gut microbiota after triple therapy, sequential therapy, bismuth quadruple therapy and concomitant therapy for Helicobacter pylori eradication in Chinese children. Helicobacter 2021;26:e12809. [Crossref] [PubMed]
- Yang C, Liang L, Lv P, et al. Effects of non-viable Lactobacillus reuteri combining with 14-day standard triple therapy on Helicobacter pylori eradication: A randomized double-blind placebo-controlled trial. Helicobacter 2021;26:e12856. [Crossref] [PubMed]
- Jakobsson HE, Jernberg C, Andersson AF, et al. Short-term antibiotic treatment has differing long-term impacts on the human throat and gut microbiome. PLoS One 2010;5:e9836. [Crossref] [PubMed]
- Yap TW, Gan HM, Lee YP, et al. Helicobacter pylori Eradication Causes Perturbation of the Human Gut Microbiome in Young Adults. PLoS One 2016;11:e0151893. [Crossref] [PubMed]
- Hsu PI, Pan CY, Kao JY, et al. Helicobacter pylori eradication with bismuth quadruple therapy leads to dysbiosis of gut microbiota with an increased relative abundance of Proteobacteria and decreased relative abundances of Bacteroidetes and Actinobacteria. Helicobacter 2018;23:e12498. [Crossref] [PubMed]
- Liou JM, Chen CC, Chang CM, et al. Long-term changes of gut microbiota, antibiotic resistance, and metabolic parameters after Helicobacter pylori eradication: a multicentre, open-label, randomised trial. Lancet Infect Dis 2019;19:1109-20. [Crossref] [PubMed]
- Hsu PI, Pan CY, Kao JY, et al. Short-term and long-term impacts of Helicobacter pylori eradication with reverse hybrid therapy on the gut microbiota. J Gastroenterol Hepatol 2019;34:1968-76. [Crossref] [PubMed]
- Gudra D, Pupola D, Skenders G, et al. Lack of significant differences between gastrointestinal tract microbial population structure of Helicobacter pylori-infected subjects before and 2 years after a single eradication event. Helicobacter 2020;25:e12748. [Crossref] [PubMed]
- Zhou Y, Ye Z, Lu J, et al. Long-term changes in the gut microbiota after 14-day bismuth quadruple therapy in penicillin-allergic children. Helicobacter 2020;25:e12721. [Crossref] [PubMed]
- Suzuki S, Gotoda T, Takano C, et al. Long term impact of vonoprazan-based Helicobacter pylori treatment on gut microbiota and its relation to post-treatment body weight changes. Helicobacter 2021;26:e12851. [Crossref] [PubMed]
- Liou JM, Jiang XT, Chen CC, et al. Second-line levofloxacin-based quadruple therapy versus bismuth-based quadruple therapy for Helicobacter pylori eradication and long-term changes to the gut microbiota and antibiotic resistome: a multicentre, open-label, randomised controlled trial. Lancet Gastroenterol Hepatol 2023;8:228-41. [Crossref] [PubMed]
- Malfertheiner P, Megraud F, Rokkas T, et al. Management of Helicobacter pylori infection: the Maastricht VI/Florence consensus report. Gut 2022; Epub ahead of print. [Crossref]
- Oh B, Kim BS, Kim JW, et al. The Effect of Probiotics on Gut Microbiota during the Helicobacter pylori Eradication: Randomized Controlled Trial. Helicobacter 2016;21:165-74. [Crossref] [PubMed]
- Pammi M, Cope J, Tarr PI, et al. Intestinal dysbiosis in preterm infants preceding necrotizing enterocolitis: a systematic review and meta-analysis. Microbiome 2017;5:31. [Crossref] [PubMed]
- Andoh A, Nishida A. Alteration of the Gut Microbiome in Inflammatory Bowel Disease. Digestion 2023;104:16-23. [Crossref] [PubMed]
- Shin NR, Whon TW, Bae JW. Proteobacteria: microbial signature of dysbiosis in gut microbiota. Trends Biotechnol 2015;33:496-503. [Crossref] [PubMed]
- Shin JH, Tillotson G, MacKenzie TN, et al. Bacteroides and related species: The keystone taxa of the human gut microbiota. Anaerobe 2024;85:102819. [Crossref] [PubMed]
- Gao JJ, Zhang Y, Gerhard M, et al. Association Between Gut Microbiota and Helicobacter pylori-Related Gastric Lesions in a High-Risk Population of Gastric Cancer. Front Cell Infect Microbiol 2018;8:202. [Crossref] [PubMed]
- Cornejo-Pareja I, Martín-Núñez GM, Roca-Rodríguez MM, et al. H. pylori Eradication Treatment Alters Gut Microbiota and GLP-1 Secretion in Humans. J Clin Med 2019;8:451. [Crossref] [PubMed]