The growth and development of children with β-thalassemia major one year after allogeneic hematopoietic stem cell transplantation
Highlight box
Key findings
• The β-thalassemia major (β-TM) children with normal nutritional levels at allogeneic hematopoietic stem cell transplantation (allo-HSCT) showed notable growth improvements within a year. The age at transplantation may impact post-transplant growth rates. Regular monitoring from diagnosis ensures optimal nutrition at allo-HSCT, improving outcomes.
What is known and what is new?
• Children with β-TM are susceptible to growth disorders due to various factors.
• Most β-TM children showed growth improvements within a year post-allo-HSCT, especially those with normal pre-transplant nutrition and younger age at transplantation.
What is the implication, and what should change now?
• Regular monitoring of growth and development from the time of diagnosis is essential to ensure optimal nutritional status before allo-HSCT, thereby enhancing the prognosis for these children post-transplantation.
Introduction
The β-thalassemia major (β-TM) is an inherited hemoglobinopathy characterized by reduced or absent synthesis of the beta globin chains in the hemoglobin tetramer. This condition leads to severe anemia, which requires lifelong red blood cell transfusions. Allogeneic hematopoietic stem cell transplantation (allo-HSCT) has proven to be an effective curative intervention for patients with transfusion-dependent β-TM (1). Children with β-TM are susceptible to growth disorders due to various factors, including chronic anemia, hypermetabolic state, excessive iron deposition, long-term transfusion, and iron chelation therapy. A previous study has demonstrated that a number of children were already malnourished, exhibiting stunted growth and being underweight prior to undergoing allo-HSCT (2). Throughout allo-HSCT, the nutritional status of these children tends to deteriorate substantially as a consequence of adverse effects stemming from drug utilization during conditioning regimens, such as graft-versus-host disease (GVHD) and oral mucositis (3), thereby exacerbating their growth and developmental impediments. Following allo-HSCT, anemia ameliorates independently of blood transfusions, and factors influencing physical development are correspondingly alleviated. However, whether malnutrition improves subsequent to allo-HSCT and whether growth retardation persists when compared to healthy children remains unclear. In China, little attention has been given to exploring the growth and development of children with β-TM following allo-HSCT. The aim of this study was to understand the longitudinal growth patterns of children with β-TM both before and after allo-HSCT, focusing on the one-year follow-up period, and to assess the potential improvement of their growth and development facilitated by allo-HSCT. We present this article in accordance with the STROBE reporting checklist (available at https://tp.amegroups.com/article/view/10.21037/tp-24-202/rc).
Methods
Patient selection
A total of 56 children diagnosed with β-TM at the Guangzhou Women and Children’s Medical Center who underwent allo-HSCT between December 2018 and August 2021 were included in this study. The study was conducted in accordance with the Declaration of Helsinki (as revised in 2013). This study was approved by the Ethics Committee of Guangzhou Women and Children’s Medical Center (No. 2019110113014196), and informed consent was obtained from the patients’ legal guardians. The conditioning regimen consisted of busulfan (BU), cyclophosphamide (CY), and antithymoglobulin (ATG). Inclusion criteria were as follows: (I) patients with confirmed genetic diagnosis of β-TM; (II) patients with normal cognitive function and communication abilities. Exclusion criteria included: (I) patients who underwent prior surgical procedures within six months prior to enrollment; (II) patients with the onset of pubertal development before transplantation.
All children were followed for one year. Disease-related and nutrition-related data were collected at six time points: one week before admission for allo-HSCT (T0), 1 month after allo-HSCT (T1), and every 3 months thereafter (T3, T6, T9, T12). A specialized dietitian monitored the patients throughout the allo-HSCT process, conducting nutritional status assessments and providing personalized guidance at each time point.
Transplantation procedures
Intravenous administration of conditioning regimens comprising BU + CY + ATG was performed. Prophylaxis against GVHD included treatment with cyclosporine (CsA) (1–3 mg/kg/day intravenously) and methotrexate (MTX) (15 mg/m2 on day 1 and 10 mg/m2 on days 3 and 6) as standard measures to prevent acute GVHD (aGVHD).
Anthropometric parameters, specifically weight and height, were measured at six designated time points by a trained investigator. Body mass index (BMI) was calculated by dividing weight in kilograms by height in meters squared. All measurements were conducted in the morning, after defecation and urination, and before breakfast. The children were instructed to wear lightweight clothing (a single shirt and pants) without shoes. A precalibrated Seca integrated electronic scale (704, Hangzhou, China) was used for accurate measurements, with height and weight rounded to the nearest 0.1 cm and 0.1 kg, respectively. Each measurement was repeated three times for reliability. The diagnosis of malnutrition in children was based on Z scores (4). Age- and sex-adjusted Z scores for height, weight, and BMI—height-for-age Z scores (HAZ), weight-for-age Z scores (WAZ), and BMI-for-age Z scores (BAZ)—were computed as outcome variables for nutritional status. These Z scores were determined according to the international reference standards for child growth established by the World Health Organization (WHO) in 2006, using WHO Anthro software (http://www.who.int/childgrowth/software/en/). According to the WHO definition, any indicator—weight, height, or BMI Z score—below −2 indicates malnutrition. Based on the Z scores obtained at T0, the nutritional status of the children was classified into two groups: the normal-nutrition group (−2≤ Z scores ≤2) and the malnutrition group (Z scores <−2 or >2). Malnutrition refers to undernutrition (wasting, stunting, underweight), with WAZ <−2 classified as underweight, BAZ <−2 classified as wasting, and HAZ <−2 classified as stunting.
Nutrition support
After allo-HSCT, children with β-TM were allowed oral intake ad libitum on a low-bacterial diet. If nasogastric tube (NGT) insertion was necessary, enteral nutrition (EN) was initiated. Continuous EN with isocaloric, lactose-free, and fiber-free feeds was administered, with adjustments made based on energy needs and gastrointestinal (GI) tolerance. If EN provided less than 75% of the estimated energy requirements for 3–7 consecutive days, parenteral nutrition (PN) was introduced. In stable patients, PN was cycled (administered for less than 24 hours per day), while EN could be temporarily paused or continued based on tolerance. Standardized PN bags containing protein, glucose, and micronutrients were prepared by the hospital pharmacy according to PN recommendations. Lipid emulsions were withheld if triglyceride levels exceeded 2.5 mmol/L or in cases of fungal sepsis. PN was gradually discontinued once oral or enteral intake reached 50–75% of requirements (5). Protein requirements were calculated using “The American Society for Parenteral and Enteral Nutrition (A.S.P.E.N.) Pediatric Nutrition Support Core Curriculum”, while energy requirements were determined using the Schofield equation to calculate the basal metabolic rate (BMR), adjusted with a factor of 1.4. The actual energy intake ratio was computed by dividing actual intake after allo-HSCT by the ideal intake during various periods.
A team of experienced dieticians closely monitored the oral dietary intake of all children at the six time points. A 24-hour diet questionnaire was employed to recall the food intake of the children.
Statistical analysis
Statistical analysis was conducted using SPSS version 26.0 software. Categorical variables were presented as frequencies and percentages. Continuous variables with a normal distribution were expressed as means and standard deviations (SD) and compared using Student’s t-test. Variables with a skewed distribution were described using medians and interquartile ranges (IQR). Categorical variables were compared using either the Chi-squared test or Fisher’s exact test. The generalized estimating equation (GEE) was used to analyze repeated measurement data between groups. The paired t-test was employed to assess differences in outcome variables between time points T0 and T12. A P value of less than 0.05 was considered statistically significant.
Results
Characteristics of the patient
A total of 56 children with β-TM underwent allo-HSCT at our hospital between December 2018 and August 2021. The mean age was 6.50±2.55 years (range, 2.67–13.58 years) (Table 1). Prior to allo-HSCT, serum ferritin levels were measured, with a median of 3,028.7 ng/mL (IQR, 2,196.1–3,588.8 ng/mL), ranging from 608.4 to 10,286.7 ng/mL. Among the 56 children, 4 (7.1%) died on days 145, 187, 233, and 248 due to respiratory failure from pulmonary infection (n=1), thrombotic microangiopathy (n=2), and cytomegalovirus encephalitis (n=1). Complications of acute GVHD, chronic GVHD, and infection occurred in 25%, 12.50%, and 58.92% of children after allo-HSCT, respectively. The incidence of infection was significantly higher in the malnutrition group compared to the normal nutrition group (85.71% vs. 50.0%, P=0.03). No significant differences were found in the distribution of acute (P=0.05, Table 1) and chronic GVHD (P=0.06, Table 1) between the groups. Additionally, 87.50% of the children developed oral mucositis, with no significant difference observed between the groups (92.86% vs. 85.71%, P=0.67, Table 1).
Table 1
Clinical characteristics | Whole group (n=56) | Normal-nutrition group (n=42) | Malnutrition group (n=14) | P value |
---|---|---|---|---|
Gender | 0.52 | |||
Male | 36 (64.29) | 28 (66.67) | 8 (57.14) | |
Female | 20 (35.71) | 14 (33.33) | 6 (42.86) | |
Age at allo-HSCT (years) | 6.50±2.55 | 5.80±0.34 | 8.60±0.63 | <0.001 |
Donor type | 0.44 | |||
Related | 27 | 19 | 8 | |
Unrelated | 29 | 23 | 6 | |
Graft type | 0.67 | |||
PBSC | 46 | 35 | 11 | |
PBSC + UCB | 8 | 5 | 3 | |
UCB | 2 | 2 | 0 | |
HLA matching status | 0.93 | |||
10/10 matched | 38 | 29 | 9 | |
9/10 matched | 12 | 8 | 4 | |
8/10 matched | 4 | 3 | 1 | |
6/10 matched | 1 | 1 | 0 | |
5/10 matched | 1 | 1 | 0 | |
Conditioning regimen (ATG + CY + Bu) | 56 | 42 | 14 | – |
Serum ferritin (ng/mL) | 2,977.15 (2,196.05, 3,577.30) |
2,977.15 (2,200.15, 3,576.90) |
3,064.10 (2,131.48, 4,362.90) |
0.78 |
Glucocorticoids | 49 (87.50) | 38 (90.48) | 11 (78.57) | 0.35 |
Acute GVHD, grade | 14 | 13 | 1 | |
1 | 3 | 2 | 1 | 0.19 |
2–4 | 13 | 13 | 0 | |
Skin | 14 | 13 | 1 | >0.99 |
Gastrointestinal tract | 5 | 5 | 0 | |
Chronic GVHD, grade | 7 (12.50) | 3 (7.14) | 4 (28.57) | >0.99 |
Skin | 6 | 3 | 3 | |
Gastrointestinal tract | 1 | 1 | 0 | |
Oral cavity | 1 | 0 | 1 | |
Infection | 33 (58.92) | 21 (50.00) | 12 (85.71) | 0.03 |
Oral mucositis | 49 (87.50) | 36 (85.71) | 13 (92.86) | 0.67 |
Data are presented as mean ± SD, median (IQR), n or n (%). Mann-Whitney U test. β-TM, β-thalassemia major; allo-HSCT, allogeneic hematopoietic stem cell transplantation; PBSC, peripheral blood stem cell; UCB, umbilical cord blood; HLA, human leukocyte antigen; ATG, antithymoglobulin; CY, cyclophosphamide; BU, busulfan; GVHD, graft-versus-host disease; SD, standard deviation; IQR, interquartile range.
Growth and development of children before allo-HSCT
Before allo-HSCT, there were 42 children in the normal-nutrition group with a mean age of 5.80±0.34 years, and 14 children in the malnutrition group with a mean age of 8.60±0.63 years (Table 1). Among them, 13 children were classified as underweight (23.2%), 10 as stunting (17.9%), and 6 as wasting (10.7%). Among all the children, the mean WAZ, HAZ, and BAZ were −0.97±1.00, −1.17±0.99, and −0.59±1.04, respectively (Figure 1, Table S1). In the normal-nutrition group, the mean WAZ, HAZ, and BAZ were −0.61±0.76, −0.84±0.87, and −0.22±0.77, respectively (Figure 2, Table S1). In the malnutrition group, the mean WAZ, HAZ, and BAZ were −2.33±0.44, −2.16±0.59, and −1.71±0.96, respectively (Figure 2, Table S2).
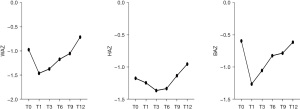
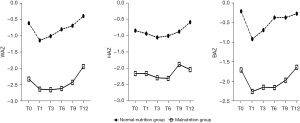
Actual ratio of energy intake after allo-HSCT
Table S3 presents the actual ratio of energy intake during different periods as percentages: (I) T0: 94.92 (84.33, 101.84); (II) T1: 74.62 (71.13, 79.42); (III) T3: 81.36 (68.43, 90.66); (IV) T6: 87.34 (77.66, 103.76); (V) T9: 95.11 (89.43, 103.67); (VI) T12: 117.08 (104.6, 126.91).
General changes in the growth and development of children after allo-HSCT
At time point T12, WAZ (n=33), HAZ (n=38), and BAZ (n=26) showed improvement compared to levels at T0 (Table S1). Most children demonstrated an improvement in nutritional status after allo-HSCT.
Changes in weight of children after allo-HSCT
One month after allo-HSCT, 88.46% (46/52) of the children experienced weight loss, with an average reduction of 8.3%. The child with GVHD affecting both skin and GI tract had the highest weight loss, with a maximum reduction of 20.16%. The mean WAZ for all children was −0.97 at T0 and decreased by 0.49 at T1 (Table S1). However, their weight gradually increased, reaching a mean WAZ of −0.71 at T12 (Table S1). The most significant increase in WAZ was seen in one child whose score improved from −2.11 at T0 to −0.3 at T12. Compared to T0, the mean WAZ at T12 showed a significant increase for all children (P=0.01, Figure 3).
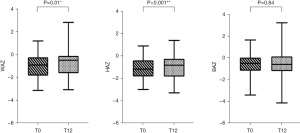
A noticeable difference in growth trends was observed between the normal-nutrition group and the malnutrition group. Children in the normal-nutrition group consistently gained weight from T1, resulting in a mean WAZ increase to −0.39 at T12 (Table S3). This difference in mean WAZ between T0 and T12 in the normal-nutrition group was statistically significant (P=0.04, Figure 4). Conversely, the malnutrition group began to gain weight at T3, with mean WAZ increasing to −1.94 at T12 (Table S3); however, the difference between T0 and T12 was not statistically significant (P=0.12, Figure 5).
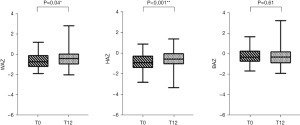
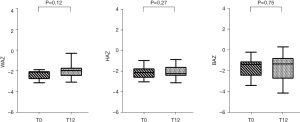
Changes in height of children after allo-HSCT
During the first three months following allo-HSCT, approximately 83% (47/56) of the children experienced minimal height growth. Height growth began to increase three months after allo-HSCT in the normal-nutrition group, while in the malnutrition group, it started to improve six months after the procedure (Figure 2). The mean HAZ for all children increased from −1.17 at T0 to −0.95 at T12, with the highest increase of 0.96 (Table S1). The increase in HAZ at 6 months after allo-HSCT was more significant in the normal-nutrition group compared to the malnutrition group. In the normal-nutrition group, HAZ improved from −0.84 at T0 to −0.58 at T12, while in the malnutrition group, HAZ increased from −2.19 at T0 to −2.04 at T12 (Table S3). Two children whose HAZ scores were above −3 at T0 fell below this threshold at T12 due to GVHD. The difference in mean HAZ at T0 and T12 was statistically significant (P<0.001, Figure 3). Furthermore, the normal-nutrition group showed a substantial increase in mean HAZ at T12 compared to T0 (P<0.001, Figure 4), while there were no significant changes in mean HAZ for the malnutrition group (P=0.27, Figure 5).
Changes in BMI of children after allo-HSCT
The mean BAZ for all children exhibited a significant decrease from −0.59 to −1.26 within one month after allo-HSCT, followed by a gradual increase (Table S1). The increase in mean BAZ from T1 to T6 was more pronounced in the normal-nutrition group compared to the malnutrition group, with both groups eventually returning to pre-allo-HSCT levels (Figure 2). In the normal-nutrition group, mean BAZ changed from −0.22 at T0 to −0.27 at T12, while in the malnutrition group, it changed from −1.73 at T0 to −1.64 at T12 (Table S3). However, no significant differences were observed in mean BAZ for all children between T0 and T12 (P=0.84, Figure 3). Similarly, there were no significant differences in mean BAZ between T0 and T12 for either the normal nutrition group (P=0.61, Figure 4) or the malnutrition group (P=0.75, Figure 5).
Incidence of increased WAZ, HAZ, and HAZ before and after allo-HSCT between groups receiving allo-HSCT aged >7 and ≤7 years
During the follow-up, four children died—two older than 7 years and two younger. Among the remaining 52 children, 19 were older than 7 years and 33 were younger. In the cohort older than 7 years, 47.4% (9/19) showed an increase in WAZ, 52.6% (10/19) in HAZ, and 47.4% (9/19) in BAZ at T12 compared to T0 (Figure S1). In contrast, 72.7% (24/33) of children younger than 7 years had a higher WAZ, 87.9% (29/33) had a higher HAZ, and 51.5% (17/33) had a higher BAZ at T12 compared to T0 (Figure S1). The incidence of increased HAZ was significantly higher in children younger than 7 years compared to those older than 7 years (P=0.01, Figure S1).
Changes in serum ferritin level of children after allo-HSCT
At baseline (T0), serum ferritin levels were 2,977.15 ng/mL in the normal-nutrition group and 3,064.10 ng/mL in the malnutrition group, with no significant difference (P=0.78, Table 2). Within the first three months, both the normal-nutrition group and the malnutrition group exhibited a significant increase in ferritin levels compared to pre-transplant values, followed by a gradual decline. By twelve months post-transplant, the normal-nutrition group had decreased to 2,109 ng/mL, and the malnutrition group had decreased to 2,544.2 ng/mL, again with no significant difference (P=0.28). Throughout the observation period, although there were changes in serum ferritin levels in both groups, these changes did not show statistically significant differences. In terms of normal-nutrition group, there is a difference between ferritin levels at T0 and T12 (P=0.007, Figure 6). However, in the group with malnutrition group, there is no statistically significant difference in ferritin levels between T0 and T12 (P=0.92, Figure 6).
Table 2
Timepoints | Normal-nutrition group (n=42) | Malnutrition group (n=14) | Z | P |
---|---|---|---|---|
T0 | 2,977.15 (2,200.15, 3,576.90) | 3,064.10 (2,131.48, 4,362.90) | −0.274 | 0.78 |
T1 | 9,572.90 (6,082.80, 11,407.35) | 8,401.10 (7,059.38, 16,026.18) | −0.504 | 0.61 |
T3 | 5,629.00 (3,994.65, 8,416.20) | 4,404.60 (4,050.05, 11,013.65) | −0.212 | 0.83 |
T6 | 3,282.50 (2,576.60, 5,146.75) | 6,144.80 (2,536.85, 9,464.10) | −1.550 | 0.12 |
T9 | 2,283.10 (1,800.6, 3,544.50) | 4,514.90 (2,042.05, 8,739.33) | −1.932 | 0.053 |
T12 | 2,109.00 (1,555.60, 3,287.20) | 2,544.20 (1,530.75, 6,313.50) | −1.088 | 0.28 |
Data are presented as median (IQR). Mann-Whitney U test. T0, prior to allo-HSCT; T1, 1 month; T3, 3 months; T6, 6 months; T9, 9 months; T12, 12 months. IQR, interquartile range; allo-HSCT, allogeneic hematopoietic stem cell transplantation.
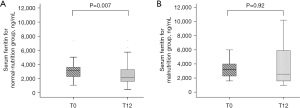
Serum ferritin level of children before and after allo-HSCT between groups receiving allo-HSCT aged >7 and ≤7 years
Initial serum ferritin levels were similar (T0) between children ≤7 years old (median 2,973.3 ng/mL) and those >7 years old (median 3,179.6 ng/mL), with no significant difference noted (P=0.56, Table 3). Following the transplant, a significant trend was observed by 12 months post-transplant (T12). The younger group demonstrated a decline in ferritin levels to a median of 1,857.40 ng/mL, which was significantly lower than that of the older group, with a median of 3,067.00 ng/mL (P=0.008). This significant difference at T12 suggests a more pronounced decrease in ferritin levels in children ≤7 years old compared to their older counterparts. In patients aged 7 years or younger, the ferritin levels significantly decreased after transplantation, which is statistically significant compared to T0 (P=0.002, Figure 7). As for those older than 7 years, the ferritin levels also decreased after transplantation, but this decrease is not statistically significant compared to T0 (P=0.72, Figure 7).
Table 3
Timepoints | Children ≤7 years old (n=34) | Children >7 years old (n=20) | Z | P value |
---|---|---|---|---|
T0 | 2,973.30 (2,198.1, 3,585.00) | 3,179.60 (2,578.60, 3,988.30) | −0.551 | 0.58 |
T1 | 9,241.70 (5,813.98, 10,765.38) | 10,306.35 (7,271.13, 16,134.68) | −1.291 | 0.20 |
T3 | 5,431.90 (4,098.70, 8,027.80) | 5,481.20 (3,996.50, 8,848.00) | −0.110 | 0.91 |
T6 | 3,036.05 (2,404.50, 4,353.90) | 5,247.55 (2,915.68, 7,142.15) | −2.486 | 0.01 |
T9 | 2,189.60 (1,667.43, 3,457.83) | 3,120.60 (2,150.80, 7,201.00) | −2.669 | 0.008 |
T12 | 1,857.40 (1,378.00, 2,916.65) | 3,067.00 (2,109.00, 5,905.80) | −2.670 | 0.008 |
Data are presented as median (IQR). Mann-Whitney U test. T0, prior to allo-HSCT; T1, 1 month; T3, 3 months; T6, 6 months; T9, 9 months; T12, 12 months. IQR, interquartile range; allo-HSCT, allogeneic hematopoietic stem cell transplantation.
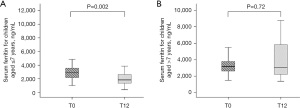
Discussion
This study assessed the growth and development of 56 children with severe β-TM in southern China over one year, aiming to evaluate their growth status before and after allo-HSCT. The results indicated that approximately 25% of the children were malnourished before transplantation. One year post-transplant, follow-up assessments revealed that about 50% of the children showed significant improvements in their Z scores. Notably, children younger than 7 years exhibited a remarkable increase in HAZ, with an improvement rate of 87.9%. This suggests that growth retardation was significantly reduced following transplantation.
Growth and developmental disorders are common among children with β-TM. In this study, the pre-transplant incidence of malnutrition was around 25%, with 23.2% classified as underweight, 17.9% experiencing stunted growth, and 10.7% being wasted. These findings align with other international studies, though the incidence of malnutrition in this study was lower than in some other countries. For instance, a study in India found that all 328 children aged 11–12 years with β-TM were underweight, and 48.2% were malnourished (6). A study in Greece reported that among 200 children with β-TM, 39.1% were underweight, 23.2% were stunted, and 6.0% were wasted (7). A. In France, the proportions of underweight and stunted patients with severe thalassemia were 20.1% and 16.1%, respectively (8).
The impact of β-TM on children’s height is a key focus of this research. Soliman et al. described the prevalence of growth in adolescents and young adults with β-TM after 2020, from 10 countries, the results indicated that growth impairment and growth hormone deficiency occurred from 31% to 49% (9). Additionally, a meta-analysis found that 48.9% of 14-year-old children experienced growth retardation, while 26.6% had growth hormone deficiency (10). In our study, 50% of the 14 growth-retarded children over 7 years old were affected, compared to only 7.1% of the 42 children under 7. A study in North America involving 361 patients with various thalassemia syndromes revealed that 25% had growth retardation or short stature (11). Children with β-TM typically start to show slower growth compared to their peers around ages 3 to 4, with this discrepancy becoming more pronounced as they age (11). The exact causes of growth retardation are unclear, but chronic anemia, the effects of extramedullary hematopoiesis on bones, and endocrine dysfunction due to excess iron deposition may all contribute, exacerbating growth disorders. Most studies suggest that chronic iron overload is the primary cause of growth retardation (12,13).
The allo-HSCT is a landmark treatment for β-TM, liberating patients from the need for long-term transfusions. However, alongside alleviating anemia symptoms, healthcare providers and parents are concerned about the potential improvement in children’s growth and development post-transplant. In particular, the first year after transplantation is critical, as the surgery and its complications can negatively impact the nutritional status of these children, an area that requires further investigation. In the early stages of allo-HSCT, GI symptoms caused by conditioning regimens—such as nausea, vomiting, diarrhea, appetite loss, and oral mucositis—significantly reduce nutritional intake. Additionally, the treatment for aGVHD with steroid hormones may lead to growth stagnation. Our study observed that 80% of children experienced weight loss within one month after allo-HSCT, with height growth nearly ceasing within 3 months post-transplant. Monitoring during this period revealed that their nutrient intake was only 70–80% of recommended levels. Growth after allo-HSCT is influenced by various factors. Alongside the aforementioned issues, early transfusions can lead to elevated serum ferritin levels, exacerbating iron overload (IO), which may be more severe than pre-transplant levels. This aligns with our findings of significant increases in serum ferritin levels within 1–3 months post-transplant. IO adversely affects the nutritional status of patients after allo-HSCT, making assessment and management of IO both before and after the procedure crucial.
Despite these challenges, our study found that most children experienced a notable alleviation of growth stagnation within 3 months post-transplant, with growth rates gradually increasing thereafter. Approximately 9 months to one year after transplantation, about half of the children had weight and height growth rates that matched or exceeded those of their healthy peers. An annual growth assessment at Prince of Wales Hospital in Hong Kong of 32 β-TM patients who survived more than 2 years post-transplant indicated that height status began improving within the first year, particularly during the first three years, leading to an average increase of 0.59 in Height Standard Deviation Score by the fifth year (13). A retrospective study in France analyzing 99 β-TM patients also reported significant improvements in weight and height post-transplant (14). A study by Jitpirasakun (15) observed distinct longitudinal growth patterns in children with β-TM treated with long-term blood transfusion and allo-HSCT, observing HSCT significantly improved height outcomes of children and adolescents with TD Hb E/β-thalassemia (15). In our study, nearly 90% of children younger than 7 years at transplantation exhibited clear catch-up growth thereafter. The findings from these studies closely align with the growth and development improvements we observed 1 year post-transplant. However, they did not delve as deeply into the changes in physical development at various time points within the first year. The follow-up periods in these studies ranged from 1–12 years post-transplant, indicating that catch-up growth in height is most significant in the initial years, with potential long-term negative impacts on height growth. This underscores the importance of long-term follow-up to fully assess the effects of transplantation on physical development. Additionally, in this study, approximately 90% of the children under the age of 7 at the time of transplantation showed significant catch-up growth post-transplant. In contrast, among children who underwent transplantation after the age of 7, only about 50% exhibited catch-up growth. Notably, HAZ demonstrated a more frequent increase in the group of children aged 7 years compared to those older than 7 years, suggesting that height improvement following allo-HSCT was associated with the recipient’s age at the time of transplantation. Another study (16), tracking 47 patients who underwent bone marrow transplantation for β-TM, identified a strong correlation between the age at transplantation and final adult height. Specifically, children who received transplantation before the age of 7 surpassed their genetic target height in adulthood, whereas those transplanted after the age of 7 failed to reach their full genetic potential. This study’s findings are similar to our results. These results highlighted a prominent occurrence of short stature among children with β-TM at the time of transplantation and emphasized the significant influence of transplantation age on post-transplantation growth rate.
Numerous studies have shown that height growth in patients with β-TM undergoing allo-HSCT may be limited, especially in those with higher serum ferritin levels. These factors highlight the potential impact of IO on growth and development. Our study indicated that younger children experienced more significant height growth post-transplant, likely due to fewer blood transfusions and lower levels of IO, which allows for greater post-transplant growth potential. Although many thalassemia-related issues, including endocrine problems, persist post-transplant—except for hemoglobin levels—growth impairments are expected to improve over time. This recovery can be attributed to several factors: first, reduced transfusion dependency decreases iron accumulation and its toxic effects. Second, proactive chelation therapy alleviates pre-transplant IO, with many children showing significant decreases in ferritin levels one year post-transplant, indicating its effectiveness. Finally, improvements in emotional and social functioning are also crucial. After transplantation, children no longer require frequent transfusions, which enhances their academic and social activities and boosts their emotional well-being (17,18). This psychosocial improvement may further support growth and development. Our data and observations align with this perspective, reflected in the assessments conducted 1 year post-transplant. Therefore, performing transplantation as early as possible and implementing effective chelation therapy to address chronic IO are critical for the subsequent recovery of growth and development in post-transplant children.
This study had a few limitations. Firstly, the sample size was relatively small, and the follow-up period after allo-HSCT was insufficient to assess long-term growth and development. Additionally, we regret not conducting growth hormone and thyroid function tests before and after transplantation in all patients, which limited our ability to determine if these children have growth hormone deficiency. Future research will include more extensive follow-up evaluations and comprehensive assessments of the endocrine profiles of these children.
Conclusions
In conclusion, most children with β-TM showed improved growth and development within 1 year after allo-HSCT, particularly those with normal nutritional status (2< WAZ, HAZ and BMZ <2) prior to transplantation and those transplanted at younger ages. This improvement is significant for their future academic and social lives. Regular monitoring of growth and development from the time of diagnosis is essential to ensure optimal nutritional status before allo-HSCT, thereby enhancing the prognosis for these children post-transplantation.
Acknowledgments
We thank the staff of Hematology and Oncology Department for their kind cooperation.
Funding: None.
Footnote
Reporting Checklist: The authors have completed the STROBE reporting checklist. Available at https://tp.amegroups.com/article/view/10.21037/tp-24-202/rc
Data Sharing Statement: Available at https://tp.amegroups.com/article/view/10.21037/tp-24-202/dss
Peer Review File: Available at https://tp.amegroups.com/article/view/10.21037/tp-24-202/prf
Conflicts of Interest: All authors have completed the ICMJE uniform disclosure form (available at https://tp.amegroups.com/article/view/10.21037/tp-24-202/coif). The authors have no conflicts of interest to declare.
Ethical Statement: The authors are accountable for all aspects of the work in ensuring that questions related to the accuracy or integrity of any part of the work are appropriately investigated and resolved. The study was conducted in accordance with the Declaration of Helsinki (as revised in 2013). This study was approved by the Ethics Committee of Guangzhou Women and Children’s Medical Center (No. 2019110113014196), and informed consent was obtained from the patients’ legal guardians.
Open Access Statement: This is an Open Access article distributed in accordance with the Creative Commons Attribution-NonCommercial-NoDerivs 4.0 International License (CC BY-NC-ND 4.0), which permits the non-commercial replication and distribution of the article with the strict proviso that no changes or edits are made and the original work is properly cited (including links to both the formal publication through the relevant DOI and the license). See: https://creativecommons.org/licenses/by-nc-nd/4.0/.
References
- Algeri M, Lodi M, Locatelli F. Hematopoietic Stem Cell Transplantation in Thalassemia. Hematol Oncol Clin North Am 2023;37:413-32. [Crossref] [PubMed]
- Delvecchio M, Cavallo L. Growth and endocrine function in thalassemia major in childhood and adolescence. J Endocrinol Invest 2010;33:61-8. [Crossref] [PubMed]
- Habibi S, Ghoreishy SM, Imani H, et al. The effect of oral nutrition supplement (ONS) on the nutritional and clinical status of patients undergoing autologous hematopoietic stem cell transplantation: study protocol for a randomized controlled clinical trial. BMC Nutr 2024;10:83. [Crossref] [PubMed]
- Bouma S. Diagnosing Pediatric Malnutrition: Paradigm Shifts of Etiology-Related Definitions and Appraisal of the Indicators. Nutr Clin Pract 2017;32:52-67. [Crossref] [PubMed]
- Becker P, Carney LN, Corkins MR, et al. Consensus statement of the Academy of Nutrition and Dietetics/American Society for Parenteral and Enteral Nutrition: indicators recommended for the identification and documentation of pediatric malnutrition (undernutrition). Nutr Clin Pract 2015;30:147-61. [Crossref] [PubMed]
- Biswas B, Naskar NN, Basu K, et al. Malnutrition, Its Attributes, and Impact on Quality of Life: An Epidemiological Study among β-Thalassemia Major Children. Korean J Fam Med 2021;42:66-72. [Crossref] [PubMed]
- Elalfy MS, Ebeid FSE, El Gendy YG, et al. Body Composition in Egyptian Children With Transfusion-dependent Thalassemia: The Impact of Nutrition and Metabolic Profile. J Pediatr Hematol Oncol 2020;42:e334-9. [Crossref] [PubMed]
- Thuret I, Pondarré C, Loundou A, et al. Complications and treatment of patients with β-thalassemia in France: results of the National Registry. Haematologica 2010;95:724-9. [Crossref] [PubMed]
- Soliman AT, De Sanctis V, Yassin M. Focus on prevalence of endocrinopathies in β-thalassemia major (TDT), intermedia (NTDT) and sickle cell disease (SCD). Italian Journal of Medicine 2020;18:104-8.
- Arab-Zozani M, Kheyrandish S, Rastgar A, et al. A Systematic Review and Meta-Analysis of Stature Growth Complications in β-thalassemia Major Patients. Ann Glob Health 2021;87:48. [Crossref] [PubMed]
- Vogiatzi MG, Macklin EA, Trachtenberg FL, et al. Differences in the prevalence of growth, endocrine and vitamin D abnormalities among the various thalassaemia syndromes in North America. Br J Haematol 2009;146:546-56. [Crossref] [PubMed]
- Almahmoud R, Hussein A, Khaja FA, et al. Growth and endocrinopathies among children with β-Thalassemia major treated at Dubai Thalassemia centre. BMC Pediatr 2024;24:244. [Crossref] [PubMed]
- Li CK, Chik KW, Wong GW, et al. Growth and endocrine function following bone marrow transplantation for thalassemia major. Pediatr Hematol Oncol 2004;21:411-9. [Crossref] [PubMed]
- Rahal I, Galambrun C, Bertrand Y, et al. Late effects after hematopoietic stem cell transplantation for β-thalassemia major: the French national experience. Haematologica 2018;103:1143-9. [Crossref] [PubMed]
- Jitpirasakun S, Pooliam J, Sriwichakorn C, et al. Differences in longitudinal growth patterns of children and adolescents with transfusion-dependent hemoglobin E/β-thalassemia and those achieving successful hematopoietic stem-cell transplantation. Int J Hematol 2022;115:575-84. [Crossref] [PubMed]
- De Simone M, Verrotti A, Iughetti L, et al. Final height of thalassemic patients who underwent bone marrow transplantation during childhood. Bone Marrow Transplant 2001;28:201-5. [Crossref] [PubMed]
- Mulas O, Efficace F, Orofino MG, et al. Health-Related Quality-of-Life Profile of Pediatric Patients with β Thalassemia after Hematopoietic Stem Cell Transplantation. J Clin Med 2023;12:6047. [Crossref] [PubMed]
- Zhang X, Wang J, Liu Y, et al. Long-term survivors demonstrate superior quality of life after haploidentical stem cell transplantation to matched sibling donor transplantation. J Transl Med 2022;20:596. [Crossref] [PubMed]