Metabolic cardiomyopathy associated with a compound heterozygous variant in NAD(P)HX dehydratase: a case report and literature review
Highlight box
Key findings
• A patient with a novel NAD(P)HX dehydratase (NAXD) gene mutation experienced a sharp deterioration after febrile illness, causing heart failure, cardiogenic shock, and ultimately death. Other reports on a drastic worsening of condition attributable to the NAXD gene mutation are rare.
What is known and what is new?
• NAXD gene mutation can contribute to severe psychomotor regression with recurrent skin lesions and death.
• We encountered a case in which the NAXD gene mutation resulted in heart failure and cardiogenic shock leading to death.
What is the implication, and what should change now?
• Lactic acid elevation, myopathy, cardiomyopathy, and rapid death after febrile disease might be important indicators that could aid in the clinical diagnosis of congenital metabolic cardiomyopathy. In the case presented here, second-generation sequencing of the whole family gene in the peripheral blood should have been conducted as soon as possible to confirm the diagnosis and treatment.
Introduction
The heart is one of the most metabolically active organs in the human body, and thus metabolic disorders can lead to insufficient myocardial energy production, resulting in cardiomyopathy. Inborn metabolic defects are common causes of childhood cardiomyopathy, and the most common types are hypertrophic and dilated cardiomyopathy, leading to heart failure, cardiogenic shock, or even sudden death. There are more than 40 reported inborn metabolic defects that cause cardiomyopathy, including fatty-acid oxidation disorders, lysosomal storage diseases, mitochondrial diseases, creatinopathy, and inborn glycosylation disorders. Errors may occur in the metabolic process, and unwanted metabolites are often produced during metabolism, which can lead to spontaneous chemical damage in the body. Specific metabolic repair enzymes are needed to help remove accumulated metabolites and prevent damage to the body (1). The ATP-dependent metabolite repair enzyme NAD(P)HX dehydratase (NAXD) is a highly conserved metabolite repair enzyme that combats the accumulation of damaged metabolites (2,3) and specifically acts on the S exome of NAD(P)HX, restoring NADHX and NADPHX to the functional cofactors NADH and NADPH, respectively. NAD(P)H plays a central role in many biochemical processes, including key oxidation-reduction reactions related to energy production, such as glycolysis, the tricarboxylic acid cycle, fatty-acid β-oxidation, the mitochondrial electron transport chain, the pentose phosphate pathway, and fatty-acid synthesis, which shows the importance of metabolite repair enzymes. The NAXD protein is highly expressed in mitochondria. In 2019, Van Bergen et al. (1) were first to report that NAXD gene mutations are associated with mitochondrial disease. This condition can lead to serious multisystem damage throughout the body after fever; which is mainly characterized by severe psychomotor development regression accompanied by recurrent skin lesions. To date, there have been few studies and reports on metabolic cardiomyopathy caused by the NAXD gene mutation. Here, we reported a case of metabolic cardiomyopathy caused by an NAXD gene compound mutation during the course of an infectious disease, with clinical manifestations of heart failure and intractable shock leading to death. To improve clinicians’ understanding of the disease, the relevant literature was reviewed. We present this case in accordance with the CARE reporting checklist (available at https://tp.amegroups.com/article/view/10.21037/tp-24-476/rc).
Case presentation
A 47-month-old boy, the third child of nonconsanguineous Chinese parents, was born at full-term after a non-eventful pregnancy, with no history of asphyxia or birth injury. His birth weight was 3,600 g. The patient was breast-fed, without feeding problems, his development was normal during the newborn period, and he exhibited no difference in growth or development compared with his peers. However, the patient was hospitalized three times for repeated weakness of both lower extremities accompanied by decreased activity endurance. Physical examination revealed a positive Gower sign. His parents were examined and found to be free of similar symptoms, and he had elder sister who had reportedly died of myocarditis and a healthy second sister (Figure 1). Laboratory tests results were as follows: a creatine kinase (CK) concentration range of 6,592–7,057 U/L, a CK-myocardial band (CKMB) concentration range of 456.07–477.08 U/L, and an aspartate aminotransferase (AST) concentration range of 514–674 U/L. After treatment with creatine phosphate, coenzyme A, adenosine triphosphate, coenzyme Q10, and fructose diphosphate sodium, muscle weakness in both lower limbs was improved.
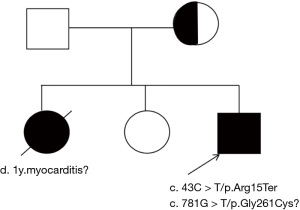
Nevertheless, due to coughing for 6 days and poor mental response for 1 hour, the patient subsequently consulted the pediatric emergency department at the Guizhou Branch of Shanghai Children’s Medical Center. On the first day of admission to the pediatric emergency department, which was the 6th day of illness, the patient exhibited a pale face and progressively decreased activity tolerance. Despite the treatment with azithromycin (5 mg/kg/d) for anti-infection, phlegm reduction, and cough relief by the emergency department physician, the patient’s condition was further worsened; therefore, who was hospitalized. Physical Examination Findings upon Admission, the patient exhibited irritability, poor mental response, a slightly pale mouth, increased heart rate and breathing, scattered medium-fine moist rales in both lungs, a low and dull heart sound, no heart murmur, grade 2 muscle strength in both upper limbs and grade 3 muscle strength in both lower limbs and no hyperplasia of the gastrocnemius muscle; weakened knee tendon and Achilles tendon reflexes. Laboratory examinations (Table 1) showed a significant increase in muscle enzymes, with a blood lactic acid concentration of 2.9 mmol/L and a positive Mycoplasma pneumoniae nucleic acid test. Echocardiography revealed left heart enlargement and an ejection fraction of 40%. Ultrasound evaluation of the kidneys indicated grade 2 renal blood flow, which was characterized by mild impairment in renal function; Moreover, the lung ultrasound showed posterior blue points on both sides (located in the area between the paravertebral line and the infrascapular line), along with numerous B-lines, which suggested the presence of pulmonary interstitial edema. Additionally, cerebral blood flow was basically normal. High-resolution computed tomography (CT) of the lung indicated bronchopneumonia. However, the cranial magnetic resonance imaging (MRI) was indeed normal. Finally, the patient was initially diagnosed with cardiomyopathy, cardiac insufficiency, bronchopneumonia, and hyperlacticaemia.
Table 1
Measure | Reference range | Outside inspection | Prehospital emergency | Hospital | |||
---|---|---|---|---|---|---|---|
Day 1 | Day 2 | Day 3 | Day 4 | ||||
Routine blood test | |||||||
White cell count (×109/L) | 6–18 | 7.38 | 3.11 | 15.73 | – | – | 17.94 |
Neutrophil count (×109/L) | 1.8–6.3 | 5.05 | 1.41 | 10.43 | – | – | 14.44 |
Neutrophile percentage (%) | 22–65 | 68.6 | 45.3 | 66.3 | – | – | 80.5 |
Platelet count (×109/L) | 125–350 | 391 | 290 | 394 | – | – | 427 |
Hemoglobin (g/L) | 95–145 | 127 | 124 | 123 | – | – | 107 |
Blood biochemistry | |||||||
C-reactive protein (mg/L) | 0–5 | <10 | 0.51 | 0.7 | – | – | 6.26 |
Procalcitonin (ng/mL) | 0–0.046 | – | – | 0.04 | – | – | – |
Alanine aminotransferase (U/L) | 7–40 | – | – | 329 | – | – | 273 |
Aspartate aminotransferase (U/L) | 13–35 | – | – | 381 | – | – | 560 |
Lactate dehydrogenase (U/L) | 110–295 | – | – | 1,622 | – | – | 2,198 |
Creatine kinase isoenzyme (U/L) | 50–310 | 2,128 | – | 9,140 | – | – | 13,198 |
α-hydroxybutyrate dehydrogenase (U/L) | 44–148 | 458 | – | 1,288 | – | – | 1,761 |
Troponin I (ng/mL) | 0–0.03 | 0.78 | – | – | – | – | – |
Troponin T (pg/mL) | 0–14 | – | – | – | 61 | – | 205 |
Myoglobin (µg/L) | <72 | – | – | – | >3,000 | – | 4,137 |
Creatine kinase isozyme (µg/L) | 0-25 | 117 | – | 611 | – | – | 278 |
Brain natriuretic peptide precursor (pg/mL) | <125 | – | – | 1,482 | – | – | >35,000 |
Urea (mmol/L) | 2.7–7 | 10.28 | |||||
Creatinine (µmol/L) | 19–44 | 60 | |||||
Arterial blood gas analysis | |||||||
pH | 7.35–7.45 | – | 7.47 | 7.30 | 7.23 | 7.45 | 7.00–7.31 |
PaCO2 (mmHg) | 35–45 | – | 35 | 34 | 33 | 55 | 47–77 |
PaO2 (mmHg) | 80–100 | – | 70 | 103 | 163 | 152 | 42–147 |
HCO3− (mmol/L) | 22–27 | – | 25.5 | 17.3 | 13.9 | 38.2 | 23.7–cannot be measured |
BE (mmol/L) | ±3 | – | 1.8 | −9.3 | −12.8 | 12.3 | −2.6–unmeasured |
Ca2+ (mmol/L) | 1.15–1.35 | – | 1.26 | 1.19 | 1.06 | 1.21 | 1.14–1.15 |
Lac (mmol/L) | 0.5–2.2 | – | 1.9 | 6.6 | 7.3 | 1.8 | 6.8–9.0 |
Glu (mmol/L) | 3.9–6.1 | – | 5.8 | 22.8 | 13.3 | 7.3 | 15.4–18.8 |
Other examinations: (I) blood ammonia, tuberculosis TSPOT, Mycoplasma pneumoniae antibody and nucleic acid, EBV antibody, sputum culture, and blood culture were negative; EB-DNA 3.32E+03 IU/mL. (II) Hearing screening: failure (AABR bilateral pass; acoustic conduction resistance test bilateral pass, left ear failure). (III) Electromyogram: signs of myogenic damage in both legs. (IV) High-resolution lung CT: double pulmonary bronchopneumonia; head MRI: no significant abnormal signal or space occupying focus in the brain parenchyma. (V) ECG: sinus tachycardia (157 bpm), rV2 < rV1, ST V1–V5 uplift and T wave fusion, QTc shortening (330 ms); cardiac ultrasound: no obvious abnormalities in heart structure, morphology or valves. PaCO2, partial pressure of carbon dioxide in artery; PaO2, arterial partial oxygen pressure; BE, base excess; lac, lactate; glu, glucose; TSPOT, tuberculosis-specific T-cell detection; EBV, Epstein-Barr virus; AABR, automatic auditory brainstem response; EB-DNA, Epstein-Barr virus deoxyribonucleic acid test; CT, computed tomography; MRI, magnetic resonance imaging; ECG, electrocardiogram.
Repeated bedside cardiac ultrasound evaluation (Figure 2) showed left heart enlargement; the systolic and diastolic functions of the heart were significantly decreased, cardiac output was decreased, and cardiac strain capacity was significantly decreased. According to ultrasound characteristics and the absence of other causes of abnormal load (hypertension, valvular disease, etc.) or dysfunction of overall contraction due to coronary artery disease, dilated cardiomyopathy (DCM) was considered. The patient had hospitalized for myopathy three times, and during hospitalization, CK, CKMB, and AST levels were significantly increased, the lactic acid level was high, and electromyography indicated myogenic damage. Given the patient’s family history, DCM caused by congenital heredity or inborn errors of metabolism (IEM) was considered. However, the patient’s family refused to provide blood and urine samples for tandem mass spectrometry or peripheral blood for whole-exome gene detection.
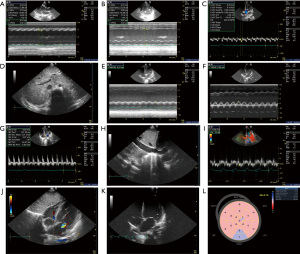
During treatment, liquid management, including fluid restriction, diuresis, continuous renal replacement therapy (CRRT) which aimed to remove excess fluid and metabolic waste from the body; enhancement myocardial contractility by using positive inotropic agents, etc. Three to four days after hospitalized, the child experienced recurrent high fever, and followed by a progressive increase in lactic acid level without the factors such as hypoxia caused by severe infection, shocking, poisoning, and other conditions. Subsequently, the diastolic and systolic functions of the heart deteriorated progressively, and thus metabolic cardiomyopathy was considered. Given this clinical suspicion, a “mitochondrial antioxidant cocktail” therapy of biotin at 10 mg/kg/d, vitamin B1 at 10 mg/kg/d, vitamin B2 at 5 mg/kg/d, vitamin C at 20 mg/kg/d, vitamin E at 10 mg/kg (QOD), coenzyme Q10 at 5 mg/kg/d, and L-carnitine at 200 mg/kg/d was commenced, and a cooling blanket was provided. Meanwhile, the patient exhibited an increase in serum troponin level. As the electrocardiogram showed extensive ST-T changes, fulminant myocarditis needed to be considered, and methylprednisolone sodium succinate (1–2 mg/kg/d) and intravenous human immunoglobulin (300–500 mg/kg/d) were added. Despite active treatment, the patient’s condition did not improve, even intractable shock developed. Extracorporeal membrane oxygenation or left ventricular assist replacement therapy was recommended, but the patient’s family refused the treatment due to poor economic conditions. Eventually, the patient died due to heart failure and cardiogenic shock (intractable). During the hospitalization, the main treatment and prognosis of the patient in the pediatric intensive care unit were summarized in Table 2, and laboratory tests and examination results were listed in Table 1.
Table 2
Day of illness | Significant clinical findings or positive auxiliary test results | Treatment or/and outcome |
---|---|---|
Illness | ||
Day 1 | Paroxysmal cough, dry cough, fever, chills | Ibuprofen, fluids |
Day 3 | Bronchopneumonia and mycoplasma infection were considered in the emergency department of our hospital | Azithromycin, vitamin C, coenzyme A, adenosine |
Day 6 | Laryngeal spasm, pale face, low spirits | Nasal duct oxygen |
Hospital | ||
Day 1 (illness day 7) | Vital signs: normal body temperature, HR 171 bpm, RR 45 bpm | Sedation, oxygen, limited fluid intake, piperacillin, and tazobactam to enhance anti-infection, diuresis. After treatment: HR 115 bpm, BP 82/40 mmHg, RR 23 bpm, CRT 2 s, lac 1.9 mmol/L, resulted in some improvement in muscle strength |
General situation: acute illness, poor mental response | ||
Breathing: slightly pale lips, thick double-breathing sound, medium-fine moist rales | ||
Circulation: jugular vein and liver jugular reflux sign negative, no murmur, no oliguria, capillary refill time 3 s, lac 2.9 mmol/L, EF 40% in bedside ultrasound | ||
Digestion: liver and spleen not palpable | ||
Nervous system and limbs: irritability, grade 2 double upper limb muscle strength, no bilateral gastrocnemius muscle hypertrophy, grade 3 double lower limb muscle strength, decrease in bilateral knee tendon and Achilles tendon reflex | ||
Day 2 (illness day 8) | Blurred consciousness; HR: 45 bpm; RR: 0 bpm; BP: 53/35 mmHg; SPO2: 58%; lac: 7.3 mmol/L | Cardiopulmonary resuscitation, tracheal intubation, mechanical ventilation, adrenaline (0.03 μg/kg/min) pumped intravenously |
Myocardial infarction indexes: myoglobin >3,000 μg/L, CKMB >300 μg/L, cTnT 61 pg/mL | CRRT | |
ECG: V2–V5 elevation with ST-T fusion | Nitroglycerin (0.2 μg/kg/min) improved coronary oxygen supply | |
Day 3 (illness day 9) | Ultrasound assessment: total systolic and diastolic failure, decreased CO (VTI 2.05 cm, no obstruction in left ventricular outflow tract), poor renal perfusion, and volume overload; BP: 71/54–83/62 mmHg; T: 37.4–39 ℃ | Active control of temperature and regulation of immunity via human immunoglobulin and hormone intravenous injection and strengthening of anti-infection effect |
Vasoactive drugs: epinephrine, deoxyadrenalin | ||
Diuretic: furosemide, tolasemide | ||
Energy support: levocarnitine, coenzyme Q10, B vitamins | ||
After treatment: HR 150 bpm, BP 87/60 mmHg, CRT 2 s, lac 2.1–1.5 mmol/L | ||
Day 4 (illness day 10) |
Ultrasound evaluation: persistently low cardiac output, recurrent fever, unstable BP, oliguria (0.67 mL/h) | Temperature control, vasoactive drugs to maintain circulation, diuretics to relieve cardiac load, CRRT |
Outcome: BP progressively decreased, HR decreased, BP and SPO2 were at 0, respectively, and death occurred after unsuccessful rescue |
CKMB, creatine kinase-myocardial band; HR, heart rate; bpm, beats per minute; RR, respiratory rate; BP, blood pressure; SPO2, pulse oxygen saturation; cTnT, cardiac troponin T; CO, cardiac output; VTI, velocity time integral; CRRT, continuous renal replacement therapy; lac, lactate; EF, ejection fraction; CRT, capillary refill time.
While hospitalized, after repeated communication with the patient’s parents, genetic analysis was performed. All procedures performed in this study were in accordance with the ethical standards of the institutional and/or national research committee(s) and with the Declaration of Helsinki (as revised in 2013). Written informed consent was obtained from the patient’s legal guardian for the publication of this case report and accompanying images. A copy of the written consent is available for review by the editorial office of this journal. Genomic DNA was extracted from peripheral white blood cells from the patient and his parents using standard techniques. Exon deletion and duplication in the DMD gene were not found (see https://cdn.amegroups.cn/static/public/tp-24-476-1.pdf), ruling out pseudomuscular dystrophy. Mitochondrial DNA was analyzed, and no suspect candidate sites were found. Exome sequencing was performed on the patient and his parents, and targeted variants were validated via Sanger sequencing (Figure 3). The patient was found to harbor two heterozygous mutations in the NAXD gene that were highly consistent with the phenotype and genetic pattern of the proband: a nonsense variant, c.43C>T (p.Arg15Ter; NM_001242882.2) in exon 1; and a missense variant, c.781G>T (p.Gly261Cys; NM_001242882.2) in exon 9. The variant c.43C>T (p.Arg15Ter) was not located in the general population database (GnomAD: http://www.gnomad-sg.org/), suggesting it is rare in the general population (frequency code: PM2_Supporting). This variant is expected to cause degradation of the encoded transcript (NMD), a gene known to be associated with a loss-of-function (LOF) variant disease. Sequencing results showed that the proband was inherited from his mother. In accordance with the American College of Medical Genetics and Genomics (ACMG: https://www.acmg.net/) guidelines, the mutation was suspected to be pathogenic. The phenotype of c.781G>T (p.Gly261Cys) was highly consistent with that of the progenitor, and the genetic pattern was consistent. Although the evidence related to c.781G>T (p.Gly261Cys) is insufficient to confirm its pathogenicity, greater attention to this gene is recommended (https://cdn.amegroups.cn/static/public/tp-24-476-2.pdf). c.781G>T (p.Gly261Cys) is not included in the Genome Aggregation Database (GnomAD) (PM2_Supporting). The rare exome variant ensemble learner (REVEL) value generated from REVEL bioinformatics prediction software was 0.857, indicating the mutation was harmful (frequency code: PP3_Moderate). This variant and the suspected pathogenic variant c.43C>T (p.Arg15Ter) detected at this time formed a compound heterozygous form (PM3). In the ACMG guidelines, the mutation is of unknown significance. Given the genetic test results, the cause of death was thus updated to metabolic cardiomyopathy associated with novel NAXD variants.
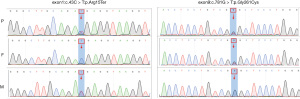
International multidisciplinary team (iMDT) discussion
Definition and diagnosis
NAXD is a key enzyme involved in the nicotinamide nucleotide repair system. Pathogenic variants of NAXD can lead to neurodegeneration caused by fever. The typical clinical features of NAXD deficiency are neurological deterioration, skin lesions, and motor abnormalities after fever, accompanied by subsequent hypotonia, nystagmus, and death (1,4). Human NAXD (formerly CARKD) has recently been associated with severe multisystem neurodegenerative diseases that can lead to death early in life (1). Van Bergen et al. reported six individuals with an NAXD pathogenic variant that led to neurodegeneration and early death following a febrile illness (1). A similar phenotype was reported for biallelic pathogenic variants of the NAXD gene in one Chinese patient and one Japanese patient (5,6). Here, the article reported another Chinese patient with a compound pathogenic variant that came from his mother who had no corresponding phenotype, which would be that the NAXD gene mutation was a recessive mutation. Additionally, gene expression regulation was a complex process influenced by many factors, such as environmental factors, lifestyle, and interactions with other genes. These factors might have led to the mutated gene in his mother not being expressed or expressed at levels insufficient to cause corresponding phenotype. Ultimately, the patient developed refractory heart failure and cardiogenic shock after lower respiratory tract infection. Tachycardia, ventricular damage, and cardiomyopathy associated with this condition have also been reported previously (6). This case expands the spectrum of NAXD phenotypes.
Current status of NAXD mutations
Searching the PubMed database (https://pubmed.ncbi.nlm.nih.gov/) using the terms “NAXD gene”, “NAD(P)HX dehydratase”, and “NAXD” for English-language literature on patients with defective NAXD expression published. A total of 11 eligible English-language articles were retrieved, among which 1 was a report of an adult patient (Table 3). The following information from the 11 papers and this case were included: sex, causative variant at the NAXD site, evidence of lack of NAXD expression, age of severe symptom onset, current condition, prior febrile illness (or other causes), skin lesions, epilepsy, myopathy, cardiac manifestations, neurodegeneration, gastroenteritis, anemia or pancytopenia, elevated CK levels, increased cerebrospinal fluid or plasma lactate levels, and mitochondrial enzyme deficiency (1,4-16). The data of the 13 NAXD gene variant were summarized in Table 3. Five female and eight male patients were included in records, all were from nonconsanguineous families who harbored eight different locus variants. The median age at onset this patient group was 2 years 10 months (from 3 months to 32 years), and 84.6% (11/13) of the patients died (the median age at death in the patient group was 2 years and 11 months). Fever before the progression of the disease occurred in 92.3% (12/13) of patients; skin lesions, epilepsy, myopathy, or heart disease were reported for 25% (3/12). The more common manifestations of the condition included neuropathy (7/12, 58%) and anemia or pancytopenia (5/8, 63%). CK and lactic acid levels were elevated in most patients, and some patients had mitochondrial enzyme defects. All patients in the review had preconditions, such as febrile illness, acute respiratory disease, gastroenteritis, or vomiting (1,5,6). Head trauma (7) could also trigger the neurometabolic crises associated with pathogenic NAXD variants. The patient did not experience epilepsy, developmental regression, or skin damage. so as a previously reported adult patient (7), and oral ulcerations, encephalitis, and right-hand paresis did not appear until the age of 4 years. Regarding the case, he had myopathy (abnormal gait and movement, such as ataxia and dystonia, accompanied by decreased activity tolerance, increased CK and CKMB levels, and increased myoglobin levels), cardiomyopathy, and fever who was followed by disease deterioration and early death. According to this literature review, approximately 25% of individuals had cardiac involvement (1,9), and 67% of patients with myopathy have gait and movement abnormality (12). Therefore, the possibility that the symptoms were associated with a variant of NAXD with incomplete penetrance can be considered.
Table 3
Feature | Patients with defects (predicted or experimentally verified) in mitochondrial expression of NAXD | Patients with NAXD deficiency at the whole-cell level | ||||||
---|---|---|---|---|---|---|---|---|
Present study | Adulthood (7) | Case 11 (8) | Case 5 (Case 3 in 9) | Case 7 (Case 5 in 9) | Case 2 (5) | 13 cases | ||
Gender | Male | Male | Male | Female | Female | Male | 8 males, 5 females | |
Pathogenic variant in the NAXD locus | c.43C>T(p.Arg15Ter) and c.781G>T (p.Gly261Cys), encompassing exons 1 and 9 of NAXD | c.441+3A>G:p.(?) | c.46G>A: (p.Val16Ile) and microdeletion encompassing exons 1 and 2 of NAXD | c.51_54delAGAA: (p.Ala20Phefs*9) | c.54_57delAAGA: (p.Ala20Phefs*9) | c.44delG (p.Arg15GlnfsTer3) and c.51_54delAGAA: (p.Ala20Phefs*9) | – | |
Evidence for the absence of NAXD expression in mitochondria | Computational prediction | Computational prediction | Computational prediction | Computational prediction | Computational prediction | Experimentally verified | – | |
Age at severe presentation | 2 years 11 months | 32 years | 16 years | 1 year 7 months | 2 years 10 months | 7 years | From 3 months to 32 years (median of all NAXD cases is 2 years and 10 months) | |
Current status/outcome | Deceased (2 years, 11 months) |
Deceased | Alive with ongoing niacin treatment | Deceased (1 year, 7 months) |
Deceased (2 years, 10 months) |
Deceased (7 years, 3 months) |
2 alive, 11 deceased (median of all NAXD cases is 2 year 11 months) | |
Febrile illness (or other trigger) prior to deterioration | Yes | No (mild head trauma) | Yes | Yes | Yes | Yes | Yes; for 12/13 reported cases | |
Skin lesions | No | Yes (oral ulceration) | No | No | No | No | Yes; 8/13 reported cases | |
Seizures | No | Yes | No | No | No | No | Yes; 5/9 reported cases | |
Myopathy | Yes | Not reported | Yes | Not reported | Yes | Yes | Yes; 4/6 reported cases | |
Cardiac presentation | Yes; myocarditis dilated, cardiomyopathy, and heart failure | No | No | No | Yes; tachycardia, dilated cardiomyopathy, and impaired ventricular function | Yes; tachycardia, dilated cardiomyopathy, and impaired ventricular function | Yes; 3/12 reported cases | |
Neurodegeneration | No | Yes | No | No | Abnormal MRI scan | Yes | Yes; 7/12 reported cases | |
Gastroenteritis | No | No | No | Yes | No | Yes | Yes; 4/8 reported cases | |
Anemia or pancytopenia | No | Not reported | Not reported | Mild anemia | Mild anemia | Mild anemia | Yes; 5/8 reported cases (3 pancytopenia, 1 leukopenia and 1 microcytic anemia) |
|
Elevated creatine kinase levels | Yes | Not reported | Yes | Not reported | Yes | Yes | Yes; 5/5 reported cases | |
Elevated lactate in cerebrospinal fluid or plasma | Yes | Not reported | Not reported | Yes | Yes | Yes | Yes; 6/10 reported cases | |
Mitochondrial enzyme defect | No | No | Yes | Not reported | No | Yes | Yes; 5/9 reported cases |
Clinical laboratory and radiology
Changes in routine blood test results of varying degrees, such as mild anemia, neutropenia, and pancytopenia, were observed in previous cases, but in our patient, the routine blood test results were normal. However, serum lactate levels were increased, which is consistent with findings in previously reported individuals (9). Past brain MRI findings include brain atrophy, abnormal white matter signaling, corpus callosum thinness, basal ganglia changes, and pontine, medullary, and cerebellar edema (1,6). However, head MRI in our patient was normal, although it should be noted that brain imaging is normal in the early stage of the disease, and brain atrophy, brain edema, and other changes may occur in the later stage.
Treatment
So far, we have no effective treatment for disorders of the nicotinamide nucleotide repair system. As the function of this system is in converting NAD(P)HX to NAD(P)H abnormality may result in lack of NAD(P)H, the active form of nicotinic acid (17). A chronic lack of nicotinic acid (vitamin B3) is known to cause pellagra, a disease presenting with skin lesions and ataxia, as similarly reported in this patient (18,19). For these reasons, vitamin B3 supplementation was considered to be of potential benefit to this patient and high dose vitamin B3 (500 mg/d) therapy was commenced upon relapse of the skin lesions. Although at this time it is too early to determine whether these are truly treatable disorders, we conclude that vitamin B3 holds promise in alleviating skin lesions and stabilizing clinical course without side effect in NAXD defect. As the episodic symptoms characteristically follow fever, early fever control also presents a simple measure for relapse prevention. Moreover, the reported (6) surviving patient with NAXD defect, leading to speculation that ‘mitochondria antioxidant cocktail’ therapy may be of benefit.
Discussion among physicians from the Guizhou Branch of Shanghai Children’s Medical Center
Pediatric intensive care unit
Mechanisms associated with NAXD
The NAXD gene encodes ATP-dependent NAD(P)HX dehydratase, which is one of the key enzymes in the nicotinamide nucleotide repair system. This enzyme targets several subcellular compartments, including mitochondria, and is universally distributed in all tissues (14). NAD(P)H is an essential cofactor in anabolic and catabolic cellular reactions and plays an important role in mitochondrial energy production. These proteins are prone to hydration under stressful conditions, such as increases in GAPDH enzyme abundance, temperature, or acidic pH (20,21). The accumulation of these NAD(P)HX [the hydrated form of NAD(P)H] derivatives has been found to inhibit several key mitochondrial dehydrogenases (21), and these hydration cofactors are thought to be cytotoxic. NAXD plays an important role in converting the S exome of the cofactor NAD(P)HX to the active form NAD(P)H (3). Van Bergen et al. (1) reported reduced expression of complex I and IV subunits and reduced respiratory chain activity in patient-derived fibroblasts, providing evidence for impaired mitochondrial function in NAXD-deficient individuals (1).
Clinical manifestations of NAXD mutations
The proband in this study developed cardiomyopathy, which rapidly progressed to heart failure, cardiogenic shock, and sudden death under the stress of increased temperature. The heart muscle relies on a high level of aerobic metabolism to pump blood to the organs of the body, providing sufficient oxygen and energy. Carbohydrates, free fatty acids (FFAs), amino acids, pyruvate, and ketone bodies are used as metabolic substrates to generate adenosine triphosphate (22). NAXD mutations lead to IEM, which is caused by a mutated enzyme or the cofactor necessary for maintaining normal metabolism in the body. IEM deficiency or abnormality can cause metabolic disorders and lead to the accumulation or deficiency of specific metabolites. These harmful metabolites accumulate in cardiomyocytes, and disturbance of myocardial energy production or utilization leads to cardiac involvement through complex regulation of gene expression, enzyme activity, and signaling pathways (22). Although we administered mitochondrial antioxidant cocktail therapy, the patient’s condition did not improve. On the fourth day of admission, the patient’s cardiac systolic and diastolic functions, blood pressure and organ perfusion were decreased, and his condition further deteriorated until death.
Pediatric cardiology department
Despite the initial treatment for patients with NAXD deficiency—niacin-based n-of-1 intervention—exhibiting early success, the exact pathway that is severely affected during crisis and disease progression remains unknown. Therefore, it would be of considerable value to explore the molecular and cellular consequences of NAXD deficiency in suitable disease models. These findings will provide new and unique insights into the most vulnerable organs involved in NAD(P)H damage and repair, such as the brain and heart.
Several issues regarding the diagnosis and treatment of this patient were further discussed as follows
- Attempts have been made to associate symptoms with the mutation sites, and there are reported splicing variants for cytosolic and mitochondrial distributions. What are the implications of c.43C>T:p.Arg15Ter on the putative mitochondrial distribution.
Antônio da Silva Menezes Júnior: the c.43C>T (p.Arg15Ter) variant in the NAXD gene, resulting in a premature stop codon, has significant implications for mitochondrial function, particularly given NAXD’s critical role in metabolite repair and energy metabolism. NAXD encodes NAD(P)HX dehydratase, an enzyme responsible for the repair of damaged forms of NADH and NADPH, collectively known as NAD(P)HX, which are essential for multiple biochemical processes, including mitochondrial energy production (1).
Mitochondrial dysfunction resulting from NAXD mutations has been implicated in a range of pathologies characterized by high energy demand, such as cardiomyopathy and neurological deficits. The heart, with its significant metabolic demands, is especially vulnerable to disruptions in energy supply, which is evident in patients presenting with cardiomyopathy, as seen in the present case. Furthermore, the accumulation of toxic metabolites could lead to direct cellular damage, increased apoptosis, and impaired contractile function of cardiomyocytes. This aligns with findings by Van Bergen et al., where NAXD mutations were associated with reduced respiratory chain activity in patient-derived fibroblasts, highlighting the systemic implications of impaired mitochondrial function (1).
The mutation’s impact on the mitochondrial distribution of NAXD could lead to an imbalance between cytosolic and mitochondrial repair mechanisms. There are reported splicing variants of NAXD that influence its distribution between the cytosol and mitochondria, suggesting that specific variants may have differential effects on cellular compartments. Since the heart relies heavily on mitochondria for energy, a loss of mitochondrial-specific NAXD activity could result in significant cardiac dysfunction, as demonstrated by the rapid progression to heart failure in this patient. The high metabolic rate and dependency on oxidative phosphorylation for ATP in cardiomyocytes means that even slight perturbations in redox balance and energy production can lead to functional deficits, which was observed as left ventricular systolic dysfunction and DCM in the current patient (1). - Is the p.Gly261Cys variant considered to be pathogenic?
Antônio da Silva Menezes Júnior: the p.Gly261Cys variant in NAXD involves substituting glycine for cysteine at position 261, a highly conserved site indicating its functional importance. The glycine residue is likely critical to maintaining the structural stability or flexibility of the NAXD protein, and substitution with cysteine, a bulkier amino acid with different chemical properties, could interfere with the protein’s function (13).
The computational prediction of pathogenicity (REVEL score of 0.857) and its absence in population databases such as GnomAD support the view that p.Gly261Cys may be pathogenic. A REVEL score above 0.5 generally suggests that a variant is likely to be damaging, and the specific score here indicates a strong likelihood of harmful effects. However, the classification as a variant of uncertain significance (VUS) by ACMG reflects the lack of sufficient functional or familial segregation evidence to definitively label it as pathogenic (13).
The available evidence does suggest that the p.Gly261Cys variant may impair the function of NAXD, particularly in conjunction with the other identified variant (c.43C>T). The patient presented with clinical symptoms consistent with NAXD deficiency, including metabolic cardiomyopathy and neurological symptoms, both of which can be exacerbated by mitochondrial dysfunction. The complex heterozygous nature of the detected variants (p.Arg15Ter and p.Gly261Cys) suggests that each contributes to the observed phenotype, possibly through a combination of LOF and partial function effects. Additionally, findings from Qz Tuncer et al. have shown that biallelic pathogenic variants of NAXD are associated with multisystemic effects, including neurodegeneration and myopathy, further supporting the pathogenic potential of p.Gly261Cys (13). - Did so many images (Figures 2,3Figures 2,322).
Moreover, metabolic cardiomyopathy can present with varying severity, and its progression can be episodic, often exacerbated by stressors such as febrile illness. The detailed imaging thus helped correlate clinical events, such as febrile episodes, with changes in cardiac function, strengthening the diagnosis of a metabolic component to the cardiomyopathy. This is particularly important given that the clinical presentation included multiple systems, with both cardiac and muscular involvement, which necessitated a thorough, documented evaluation of the heart’s function over time.
Although it could be argued that representative images might suffice for initial diagnosis, the comprehensive imaging approach adopted in this case was clinically justified. It provided important information for both diagnosis and management, particularly given the rapid progression of heart failure and the patient’s poor response to treatment. Multiple imaging sessions allowed for the evaluation of the left ventricular function’s decline and the onset of DCM, contributing to the overall assessment that the underlying cause was likely related to metabolic impairment due to the NAXD mutations. Nishida and Otsu have emphasized the importance of continuous monitoring in metabolic cardiomyopathies to better understand disease progression and response to intervention, further supporting the utility of multiple imaging studies in this case (22).
Conclusions
We reported a case of a compound heterozygous individual with novel nonsense and missense variants in the NAXD gene. Lactic acid level increase, myopathy, cardiomyopathy, and rapid death after febrile disease might be important indicators that could aid in the clinical diagnosis of this disease. NAXD deficiency can be classified as a metabolite repair disorder, in which the accumulation of damaged metabolites has a devastating effect on highly metabolic organs such as the heart, ultimately leading to early death in childhood. The possibility of metabolic cardiomyopathy should be considered in pediatric patients with heart failure who exhibited biochemical abnormalities, such as elevated lactic acid levels, acidosis, and increased muscle enzymes.
Acknowledgments
We thank all the participants involved in this research, the patient, and his family.
Funding: None.
Footnote
Reporting Checklist: The authors have completed the CARE reporting checklist. Available at https://tp.amegroups.com/article/view/10.21037/tp-24-476/rc
Peer Review File: Available at https://tp.amegroups.com/article/view/10.21037/tp-24-476/prf
Conflicts of Interest: All authors have completed the ICMJE uniform disclosure form (available at https://tp.amegroups.com/article/view/10.21037/tp-24-476/coif). The authors have no conflicts of interest to declare.
Ethical Statement: The authors are accountable for all aspects of the work in ensuring that questions related to the accuracy or integrity of any part of the work are appropriately investigated and resolved. All procedures performed in this study were in accordance with the ethical standards of the institutional and/or national research committee(s) and with the Helsinki Declaration (as revised in 2013). Written informed consent was obtained from the patient’s legal guardian for the publication of this case report and accompanying images. A copy of the written consent is available for review by the editorial office of this journal.
Open Access Statement: This is an Open Access article distributed in accordance with the Creative Commons Attribution-NonCommercial-NoDerivs 4.0 International License (CC BY-NC-ND 4.0), which permits the non-commercial replication and distribution of the article with the strict proviso that no changes or edits are made and the original work is properly cited (including links to both the formal publication through the relevant DOI and the license). See: https://creativecommons.org/licenses/by-nc-nd/4.0/.
References
- Van Bergen NJ, Guo Y, Rankin J, et al. NAD(P)HX dehydratase (NAXD) deficiency: a novel neurodegenerative disorder exacerbated by febrile illnesses. Brain 2019;142:50-8. [Crossref] [PubMed]
- Linster CL, Van Schaftingen E, Hanson AD. Metabolite damage and its repair or pre-emption. Nat Chem Biol 2013;9:72-80. [Crossref] [PubMed]
- Marbaix AY, Noël G, Detroux AM, et al. Extremely conserved ATP- or ADP-dependent enzymatic system for nicotinamide nucleotide repair. J Biol Chem 2011;286:41246-52. [Crossref] [PubMed]
- Rafter GW, Chaykin S, Krebs EG. The action of glyceraldehyde-3-phosphate dehydrogenase on reduced diphosphopyridine nucleotide. J Biol Chem 1954;208:799-811.
- Kremer LS, Danhauser K, Herebian D, et al. NAXE Mutations Disrupt the Cellular NAD(P)HX Repair System and Cause a Lethal Neurometabolic Disorder of Early Childhood. Am J Hum Genet 2016;99:894-902. [Crossref] [PubMed]
- Zhou J, Li J, Stenton SL, et al. NAD(P)HX dehydratase (NAXD) deficiency: a novel neurodegenerative disorder exacerbated by febrile illnesses. Brain 2020;143:e8. [Crossref] [PubMed]
- Van Bergen NJ, Gunanayagam K, Bournazos AM, et al. Severe NAD(P)HX Dehydratase (NAXD) Neurometabolic Syndrome May Present in Adulthood after Mild Head Trauma. Int J Mol Sci 2023;24:3582. [Crossref] [PubMed]
- Yu D, Zhao FM, Cai XT, et al. Clinical and genetic features of early-onset progressive encephalopathy associated with NAXE gene mutations. Zhongguo Dang Dai Er Ke Za Zhi 2018;20:524-258. [Crossref] [PubMed]
- Borna NN, Kishita Y, Abe J, et al. NAD(P)HX dehydratase protein-truncating mutations are associated with neurodevelopmental disorder exacerbated by acute illness. Brain 2020;143:e54. [Crossref] [PubMed]
- Van Bergen NJ, Walvekar AS, Linster CL, et al. Reply: Niacin therapy improves outcome and normalizes metabolic abnormalities in an NAXD-deficient patient. Brain 2022;145:e41-e42. [Crossref] [PubMed]
- Manor J, Calame DG, Gijavanekar C, et al. Niacin therapy improves outcome and normalizes metabolic abnormalities in an NAXD-deficient patient. Brain 2022;145:e36-40. [Crossref] [PubMed]
- Majethia P, Mishra S, Rao LP, et al. NAD(P)HX dehydratase (NAXD) deficiency due to a novel biallelic missense variant and review of literature. Eur J Med Genet 2021;64:104266. [Crossref] [PubMed]
- Qz Tuncer G, Randa NC, Aydin S, et al. A Case with NAD(P)HX Dehydratase (NAXD) Deficiency: A Newly Defined Mutation in a Novel Neurodegenerative Disorder. Mol Syndromol 2022;13:332-6. [Crossref] [PubMed]
- Marbaix AY, Tyteca D, Niehaus TD, et al. Occurrence and subcellular distribution of the NADPHX repair system in mammals. Biochem J 2014;460:49-58. [Crossref] [PubMed]
- Cepress M, Grund E, Leng T, et al. Progressive encephalopathy after routine 4-month immunizations in a patient with NAXD genetic variant. Am J Med Genet A 2024;194:e63519. [Crossref] [PubMed]
- Xu C, Zhou L, Jiang H, et al. Establishment of an iPSC line (BCHNDi001-A) from a patient with nicotinamide nucleotide repair system deficiency caused by biallelic NAXD mutations. Stem Cell Res 2024;76:103346. [Crossref] [PubMed]
- Ying W. NAD+/NADH and NADP+/NADPH in cellular functions and cell death: regulation and biological consequences. Antioxid Redox Signal 2008;10:179-206. [Crossref] [PubMed]
- Hegyi J, Schwartz RA, Hegyi V. Pellagra: dermatitis, dementia, and diarrhea. Int J Dermatol 2004;43:1-5. [Crossref] [PubMed]
- Malik MU, Nadir H, Jessop ZM, et al. Cutaneous manifestations of NAXD deficiency - A case report. Ann Med Surg (Lond) 2020;60:352-5. [Crossref] [PubMed]
- Yoshida A, Dave V. Inhibition of NADP-dependent dehydrogenases by modified products of NADPH. Arch Biochem Biophys 1975;169:298-303. [Crossref] [PubMed]
- Tril VE, Burlutskaya AV, Polischuk LV. Metabolic cardiomyopathy in pediatrics. Rev Cardiovasc Med 2019;20:73-80. [Crossref] [PubMed]
- Nishida K, Otsu K. Inflammation and metabolic cardiomyopathy. Cardiovasc Res 2017;113:389-98. [Crossref] [PubMed]