Value of cystatin C for Kawasaki disease with coronary artery aneurysm
Highlight box
Key findings
• Cystatin C (Cys C) acquired within five days of disease onset may theoretically provide better prediction power for the coronary artery aneurysm (CAA) development in Kawasaki disease (KD).
What is known and what is new?
• There are bidirectional relationships between cardiovascular and kidney disease out of shared biological factors (hemodynamic regulatory mechanisms and renin-angiotensin-aldosterone system axis). Cys C may have an impact on the process of myocardial remodeling through suppressing the activity of endogenous cysteine protease. CAA is a severe cardiac consequence of KD. However, the relationship between Cys C and CAA is not yet clear.
• The potential of Cys C as a biomarker to predict CAA in KD patients.
What is the implication, and what should change now?
• KD patients within five days of disease onset with a level of Cys C more than 0.71 mg/L may require closer monitoring for the formation of CAAs at the one-month follow-up.
Introduction
Since Kawasaki disease (KD) was first reported in 1967, over 50 years have passed, and it is predominantly seen in Asian children younger than five years old (1). KD is characterized as an acute, self-limiting vasculitis of unidentified etiology, and its incidence has increased yearly in recent years (2). In developed countries, KD has risen to prominence as the principal cause of acquired cardiovascular disorders in children (3). Besides, KD may serve as a notable risk for the development of myocardial infarction during young adult years (4). The aetiology of KD, which is not yet clear, currently includes infection, immunity, and genetic susceptibility (5), and infection may trigger KD in genetic susceptible individuals (6). After the acute phase of KD, coronary artery damage, such as coronary artery aneurysms (CAAs) (3), and thrombosis, may remain. Therefore, reducing inflammatory injuries to the coronary arteries and avoiding the development of coronary thrombosis are the main objectives of treating KD in the acute phase. Intravenous immunoglobulin (IVIG) in combination with aspirin is currently a standard treatment for KD, and other treatments include glucocorticoids, infliximab, cyclosporine (3), urinastatin (7), plasma exchange (8) and so on. Given the absence of a certain pathognomonic test in patients with KD, they might be misdiagnosed, resulting in missing the best time to administer IVIG therapy. Regrettably, approximately 25% of untreated KD patients’ coronary arteries may be damaged and develop into coronary artery lesion (CAL), including CAA and even coronary artery thrombus (CAT) (9). More seriously, acute myocardial infarction or even sudden death may occur (10). Therefore, it is crucial to find risk factors that can predict KD with CALs early.
Although there is plenty of research on risk factors for CAA and prognosis of KD, research (11,12) has mainly focused on laboratory indicators before IVIG therapy, with few comparisons of pre- and post-treatment indicators. At the same time, common laboratory indicators, such as complete blood count, C-reactive protein (CRP), kidney and liver function, electrolyte tests, are nearly available at all medical institutions. Especially for renal function, it has received very little attention in KD. In 2020, Junji Azuma’s team (13) have linked KD to kidney involvement and found that the maximum value of urinary β2-microglobulin before IVIG treatment was significantly associated with CALs in KD. But the pathophysiology of β2-microglobulin associated with KD was not mentioned in their study (13). However, there are bidirectional relationships between cardiovascular and kidney disease out of shared biological factors (14), such as hemodynamic regulatory mechanisms and renin-angiotensin-aldosterone system axis (15). Previous studies on cystatin C (Cys C) in cardiovascular disease have mainly focused on adult’s coronary artery damage (16), coronary artery calcification (17,18), and vasospastic angina (19,20). In a study about children with sickle cell disease and β-thalassemia, Diana Hanna’s team (21) found that Cys C could be a promising biomarker for subclinical cardiovascular dysfunction because Cys C may have an impact on the process of myocardial remodeling through suppressing the activity of endogenous cysteine protease. In a clinical trial [2003] (22) between patients with KD and patients without infections showed that the levels of Cys C decreased in the former. However, the research on the relationship between Cys C and CAA is not yet clear. Besides, prealbumin and prognostic nutritional index (PNI) are both associated with humans’ statuses of nutrition, immunology, and inflammation. These indicators are all easy to detect. For some underdeveloped countries and regions, to predict the presence of CAA in children with KD via common laboratory indicators may reduce the healthcare burden on families. Thus, further exploration of common laboratory indicators is economical, simple, and easy to perform, with high adoption rate for rapid and repeated measurements. Therefore, this study was designed to investigate the predictive value of common indicators before and after IVIG treatment for CAA and prognosis, so that intensive treatment can be given to the patients at an early stage as soon as possible. We present this article in accordance with the STARD reporting checklist (available at https://tp.amegroups.com/article/view/10.21037/tp-2024-516/rc).
Methods
Study design and population
Patients with KD of this study were admitted to The First Affiliated Hospital of Guangxi Medical University between January 1, 2014, and December 31, 2022. A total of 122 KD children who received IVIG therapy less than 10 days after fever onset were enrolled in this retrospective study. The KD diagnostic criteria are based on the 2017 adaptation of the American Heart Association (AHA) guidelines (23), and the Japanese diagnostics guide, sixth revision (24). The diagnosis of KD was determined by at least two pediatric cardiologists, and CAAs were measured by no less than two experienced echocardiograph physicians. CAAs were defined based on Z-scores ≥2.5 which were adjusted for the body surface area. Only those cases with a maximum Z-scores ≥2.5 persisting for over one month after fever onset were considered CAA cases. As described in the AHA guidelines (23), CAA regression of patients with follow-up echocardiogram was considered of a normal size (Z-scores of <2.5), as well as normal appearance of each coronary artery on echocardiography, and normal cardiac function. Then, in accordance with the AHA guidelines (23), all KD patients were treated appropriately. First, all patients were divided into the no CAA (NCAA) group (n=94) and CAA group (CAA, n=28), based on the presence or absence of CAA. It was vital for patients to have necessary echocardiography follow-up data. Later, from the 28 patients with CAA, 12 patients who had CAA regression within a year after the acute phase of diseases were allocated to the normal group, while the remaining 16 patients were classified as the persistent CAA group because their CAA persisted for one year (Figure 1). This research was approved by the Medical Ethic Committee of The First Affiliated Hospital of Guangxi Medical University [No. 2021(KY-E-240)]. The study was conducted in accordance with the Declaration of Helsinki and its subsequent amendments. An informed consent was obtained from the parents of each subject.
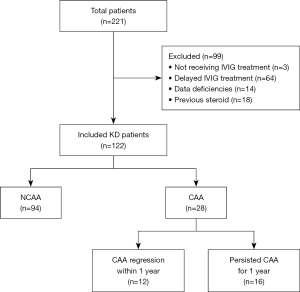
Data collection
The following information was logged for demographic, clinical, and laboratory parameters: (I) general demographic information, such as gender, body mass index (BMI), and months of age at disease onset; (II) clinical features, including the proportion of small CAAs, conjunctival injection, changes in the lips and oral cavity, polymorphous exanthem, changes in the extremities, cervical lymphadenopathy, duration of fever before admission, total days of fever, illness day at diagnosis (considering the first day of fever to be the first day of illness onset), illness day at IVIG treatment, hospital days, as well as the proportion of IVIG-refractory cases; (III) laboratory parameters obtained before and 24–36 hours after IVIG, and one month after KD onset, including white blood cell count (WBC), plateletocrit (PCT), haemoglobin concentration (Hb), neutrophil count (N), lymphocyte count (L), monocyte count (M), platelet count (PLT), CRP concentration, sodium concentrations (Na+), total bilirubin level (TB), aspartate aminotransferase (AST) level, alanine aminotransferase (ALT) level, prealbumin (PA), globulin (GLB), serum albumin (ALB), endogenous creatinine clearance (Ccr), uric acid (UA), Cys C, detected by immunoturbidimetry assays, and more detailed procedures referred to this website (https://www.immunodiagnostics.com.hk/), creatine kinase MB isoenzyme to creatine kinase ratio (CK-MB/CK) and PNI [PNI = ALB (g/L) + 5 × L (×109/L)]. Indicators for each patient were reviewed by at least two fellowship-trained laboratory technicians, who were blinded to all patient information, including the final clinical diagnosis and prognosis (4). In the acute phase of KD, before IVIG therapy, echocardiography was regularly performed, and it was repeated at months 1, 3, 6 and 12 after fever onset or till the CAAs finally shrank back to their normal size. In this study, the Z-scores of the internal diameter of the coronary arteries were calculated by the Lambda-Mu-Sigma (LMS) method (25).
Statistical analysis
In this study, the normality of the data distribution was confirmed by the homogeneity test and Shapiro-Wilk test. Data that were normally distributed were presented as mean ± standard deviation. To compare data from different subgroups, one-way analysis of variance or two-independent-sample t-tests were performed. Skewed continuous data were expressed in the form of medians (interquartile ranges), and then comparisons between groups were performed by rank-sum tests. Categorical data were represented by percentages (%). Intergroup data were compared by Fisher’s exact test, Chi-squared test, or Pearson’s Chi-squared test. The presence of multicollinearity was examined by variance inflation factor (VIF), and a multivariate logistic regression model was then employed to assess potential risk factors. The critical thresholds values for some selected variables were determined through receiver operating characteristic (ROC) curve analysis. All missing values of the variables we included were less than 10% and we disregarded this missing. SPSS 27.0 for Windows (IBM Corporation, Armonk, NY, USA) was used for all statistical analyses, with a P value of <0.05 considered significant.
Results
Baseline characteristics
Initially, this retrospective study included a total of 221 children with a diagnosis of KD. Among these, three were excluded due to not receiving IVIG treatment, 64 due to receiving delayed IVIG treatment, 14 because of significant data deficiencies, and 18 due to previous steroid or IVIG therapy at other medical institutions. Finally, a total of 122 KD children who received IVIG therapy less than 10 days after fever onset were included in this retrospective study (Figure 1). Of the 122 patients included finally, 28 (23.0%) patients developed CAA, which persisted for one year in 16 patients (13.1%). During the one-year median follow-up time frame, there were no major adverse cardiovascular events, mainly referring to recurrent angina, acute myocardial infarction, severe arrhythmia, or heart failure among all patients. The participants ranged in age from 3 to 117 months, with a mean age of 29.6 months. There were 92 males and 30 females, in a 3.1:1 gender ratio. During this period, no child in this study received any additional medication, including cyclophosphamide, infliximab, cyclosporine, anakinra, or plasmapheresis. All patients received initial IVIG treatment (2 g/kg) and appropriate doses of oral aspirin (firstly 30–50 mg/kg/d and gradually decreased to 3–5 mg/kg/d after the body temperature returned to normal) based on the 2017 AHA guidelines (23). Among all KD patients, there were 26 IVIG-refractory cases (26/122, 21.3%); in the KD subgroups, five IVIG-refractory cases were in the CAA group (n=28), 21 in the NCAA group (n=94) [5/28 (17.9%) vs. 21/94 (22.3%), P=0.61], two in the normal group (n=12) and three in the persistent CAA group (n=16) [2/12 (16.7%) vs. 3/16 (18.8%), P>0.99, Table 1]. All these refractory cases required treatment with corticosteroids. Methylprednisolone was injected intravenously at a dosage of 2 mg/kg/d. Then, the drug dosage was gradually decreased and tapered off until the patients’ CRP level returned to normal.
Table 1
Baseline characteristics | CAA group (n=28) |
NCAA group (n=94) |
P value | Normal group (n=12) | Persistent CAA group (n=16) | P value |
---|---|---|---|---|---|---|
Demographic characteristics | ||||||
Male gender | 24 (85.7) | 68 (72.3) | 0.15 | 11 (91.7) | 13 (81.3) | 0.61 |
Age (months) | 25.5 (10.1, 38.3) | 22.0 (14.0, 41.5) | 0.70 | 22.0 (9.0, 39.5) | 25.5 (10.6, 38.3) | 0.66 |
BMI (kg/m2) | 15.3 (14.5, 16.7) | 15.5 (14.5, 16.5) | 0.89 | 15.8 (15.3, 17.8) | 14.7 (14.1, 15.4) | 0.008 |
Clinical characteristics | ||||||
Proportion of small CAAs | – | – | – | 12 (100) | 9 (56.3) | 0.03 |
Conjunctival injection | 18 (64.3) | 70 (74.5) | 0.29 | 7 (58.3) | 11 (68.8) | 0.57 |
Changes in the lips and oral cavity | 18 (64.3) | 62 (66.0) | 0.87 | 7 (58.3) | 11 (68.8) | 0.57 |
Polymorphous exanthem | 15 (53.6) | 69 (73.4) | 0.047 | 7 (58.3) | 8 (50.0) | 0.66 |
Changes in the extremities | 5 (17.9) | 36 (38.3) | 0.040 | 2 (16.7) | 3 (18.8) | >0.99 |
Cervical lymphadenopathy | 14 (50.0) | 50 (53.2) | 0.77 | 7 (58.3) | 7 (43.8) | 0.45 |
Fever duration before admission (days) | 5.5 (4.0, 7.0) | 6.0 (4.0, 7.0) | 0.47 | 5.2±1.9 | 5.4±2.2 | 0.73 |
Total fever days | 8.0 (7.0, 9.0) | 8.0 (7.0, 10.0) | 0.64 | 8.6±1.6 | 8.3±1.6 | 0.66 |
Days of illness at diagnosis | 7.0 (5.0, 8.0) | 7.0 (5.0, 8.0) | 0.55 | 6.6±2.3 | 7.4±2.6 | 0.38 |
Days of illness at primary treatment | 7.0 (6.0, 8.0) | 6.0 (5.0, 8.0) | 0.27 | 6.6±1.8 | 7.1±1.8 | 0.49 |
Hospital days | 7.5 (5.0, 10.5) | 7.0 (4.0, 8.0) | 0.15 | 8.2±4.8 | 7.8±3.6 | 0.83 |
Proportion of IVIG-refractory cases | 5 (17.9) | 21 (22.3) | 0.61 | 2 (16.7) | 3 (18.8) | >0.99 |
Data are presented as number (percentage), median (interquartile range), or mean ± SD. BMI, body mass index; CAA, coronary artery aneurysm; IVIG, intravenous immunoglobulin; NCAA, no coronary artery aneurysm; SD, standard deviation.
Comparisons of baseline characteristics
The analysis results of baseline characteristics between subgroups are shown in Table 1. Between the CAA group and NCAA group, the former had less patients with polymorphous exanthem (P=0.047) and changes in the extremities (P=0.040) than the NCAA group, and no statistically significant differences were observed in the remaining characteristics (P>0.05). Between the normal group and the persistent CAA group, the former had higher BMI and higher proportion of small CAAs than the latter (P=0.008).
Laboratory parameters pre- and post-IVIG treatment and after one month of KD onset between groups
In pre-IVIG treatment, the CAA group had higher Cys C (P=0.02) but lower Ccr (P=0.048) than the NCAA group, but no significant differences were found in any of the measured indicators between groups with or without CAA recovery (P>0.05, Table 2). Unexpectedly, no statistically significant differences were observed in any of the markers (all P>0.05) neither after IVIG therapy (Table 3), nor after one month of KD onset (Table 4). No adverse events occurred during every blood sampling.
Table 2
Laboratory parameters | CAA group (n=28) | NCAA group (n=94) | P value | Normal group (n=12) | Persistent CAA group (n=16) | P value |
---|---|---|---|---|---|---|
WBC (×109/L) | 15.3±6.8 | 14.5±5.7 | 0.54 | 18.1±6.3 | 13.2±6.6 | 0.06 |
N (×109/L) | 10.5±6.2 | 9.8±5.1 | 0.57 | 12.4 (8.4, 17.1) | 7.7 (6.3, 11.8) | 0.08 |
L (×109/L) | 3.2 (1.9, 4.7) | 2.8 (1.6, 4.6) | 0.88 | 3.6±2.1 | 3.0±1.8 | 0.36 |
M (×109/L) | 0.7 (0.2, 1.2) | 0.9 (0.5, 1.4) | 0.44 | 1.1±0.9 | 0.7±0.6 | 0.25 |
Hb (g/L) | 104.4±15.7 | 105.8±13.8 | 0.65 | 100.4±13.6 | 107.4±16.9 | 0.25 |
PLT (×1012/L) | 305.6 (237.4, 429.4) | 319.7 (267.7, 433.1) | 0.19 | 365.1±142.1 | 269.7±137.9 | 0.09 |
PCT | 0.24 (0.18, 0.34) | 0.26 (0.21, 0.35) | 0.10 | 0.29±0.11 | 0.21±0.11 | 0.07 |
CRP (mg/L) | 90.3 (39.8, 151.2) | 86.3 (51.1, 148.1) | 0.82 | 139.1 (29.7, 192.0) | 59.1 (43.7, 105.0) | 0.21 |
Na+ (mmol/L) | 135.0 (133.5, 137.2) | 135.1 (132.7, 137.4) | 0.63 | 134.8 (134.0, 135.9) | 136.0 (132.8, 138.0) | 0.35 |
CKMB/CK | 0.39 (0.20, 0.64) | 0.38 (0.23, 0.62) | 0.89 | 0.54±0.31 | 0.36±0.20 | 0.09 |
Ccr (mL/min) | 86.0 (69.4, 104.2) | 92.0 (81.5, 112.6) | 0.048 | 85.4±29.9 | 93.3±27.3 | 0.48 |
UA (µmol/L) | 202.0 (138.0, 229.5) | 182.0 (146.0, 239.0) | 0.91 | 189.0 (132.0, 241.0) | 202.0 (136.0, 217.0) | 0.63 |
Cys C (mg/L) | 0.93±0.31 | 0.81±0.20 | 0.02 | 0.99 (0.75, 1.11) | 0.86 (0.73, 1.01) | 0.24 |
ALT (U/L) | 46.0 (22.5, 88.5) | 55.0 (19.5, 112.0) | 0.39 | 80.3±60.5 | 41.9±28.5 | 0.06 |
AST (U/L) | 36.0 (25.0, 63.5) | 37.0 (26.0, 60.5) | >0.99 | 40.0 (23.8, 54.5) | 31.0 (25.0, 71.0) | 0.93 |
TB (µmol/L) | 6.7 (5.1, 14.2) | 6.6 (3.5, 13.5) | 0.48 | 10.5 (6.0, 18.3) | 5.2 (4.6, 9.5) | 0.07 |
ALB (g/L) | 34.3±5.4 | 36.1±5.3 | 0.12 | 34.4±4.4 | 34.2±6.2 | 0.93 |
GLB (g/L) | 25.6±5.9 | 25.9±7.1 | 0.81 | 25.4±4.8 | 25.7±6.8 | 0.88 |
PA (mg/L) | 73.3 (40.1, 100.5) | 76.2 (55.7, 109.7) | 0.26 | 70.9 (31.2, 94.1) | 75.7 (49.2, 100.9) | 0.43 |
PNI | 47.8 (42.3, 60.9) | 51.8 (44.2, 58.7) | 0.44 | 52.6±13.2 | 49.0±14.2 | 0.50 |
Data are presented as median (interquartile range), or mean ± SD. ALB, serum albumin; ALT, alanine aminotransferase; AST, aspartate aminotransferase; CAA, coronary artery aneurysm; CKMB/CK, creatine kinase MB isoenzyme to creatine kinase ratio; CRP, C-reactive protein; Ccr, endogenous creatinine clearance; Cys C, cystatin C; GLB, globulin; Hb, haemoglobin concentration; IVIG, intravenous immunoglobulin; KD, Kawasaki disease; L, lymphocyte count; M, monocyte count; Na+, sodium concentrations; N, neutrophil count; NCAA, no coronary artery aneurysm; PA, prealbumin; PCT, plateletocrit; PLT, platelet count; PNI, prognostic nutritional index; SD, standard deviation; TB, total bilirubin; UA, uric acid; WBC, white blood cell count.
Table 3
Laboratory parameters | CAA group (n=28) |
NCAA group (n=94) |
P value | Normal group (n=12) |
Persistent CAA group (n=16) | P value |
---|---|---|---|---|---|---|
WBC (×109/L) | 10.2 (7.9, 13.5) | 9.3 (7.7, 13.6) | 0.78 | 9.4 (8.0, 15.7) | 11.0 (7.9, 12.9) | 0.59 |
N (×109/L) | 5.5 (2.9, 7.0) | 3.9 (2.4, 7.3) | 0.62 | 4.8±2.3 | 5.1±3.5 | 0.80 |
L (×109/L) | 4.3 (3.3, 5.4) | 4.0 (3.2, 5.4) | 0.71 | 4.6±1.4 | 4.2±1.8 | 0.62 |
M (×109/L) | 0.9 (0.6, 1.4) | 0.8 (0.6, 1.2) | 0.50 | 0.9 (0.7, 1.4) | 0.9 (0.5, 1.5) | 0.70 |
Hb (g/L) | 99.4±14.2 | 101.2±13.0 | 0.54 | 96.8±11.3 | 101.4±16.2 | 0.41 |
PLT (×1012/L) | 479.8 (388.3, 589.1) | 499.0 (367.5, 659.5) | 0.45 | 511.1±144.6 | 456.0±169.0 | 0.38 |
CRP (mg/L) | 17.5 (10.0, 39.8) | 16.3 (10.0, 49.2) | 0.97 | 12.0 (10.0, 44.1) | 28.0 (11.9, 38.8) | 0.70 |
ALT (U/L) | 24.0 (14.3, 54.5) | 26.0 (17.5, 44.0) | 0.71 | 24.0 (13.3, 54.5) | 25.0 (15.5, 56.8) | 0.89 |
AST (U/L) | 38.5 (30.8, 50.8) | 37.0 (30.0, 46.0) | 0.29 | 35.5 (30.0, 54.8) | 40.0 (31.8, 44.8) | 0.57 |
TB (µmol/L) | 4.3 (2.7, 10.0) | 4.9 (2.8, 7.3) | 0.53 | 5.5 (3.8, 11.9) | 3.7 (2.6, 7.0) | 0.10 |
ALB (g/L) | 34.7±7.4 | 34.3±6.0 | 0.77 | 36.6±6.6 | 33.0±8.0 | 0.24 |
GLB (g/L) | 37.2±8.4 | 39.9±8.6 | 0.17 | 37.3±10.3 | 37.1±6.6 | 0.97 |
PA (mg/L) | 161.4±64.8 | 149.1±59.2 | 0.37 | 148.1 (112.6, 221.1) | 147.1 (122.6, 162.4) | 0.85 |
PNI | 56.6 (50.1, 65.0) | 54.4 (48.5, 62.9) | 0.3 | 59.4±8.7 | 53.8±12.5 | 0.21 |
Data are presented as mean ± SD, or median (interquartile range). ALB, serum albumin; ALT, alanine aminotransferase; AST, aspartate aminotransferase; CAA, coronary artery aneurysm; CRP, C-reactive protein; GLB, globulin; Hb, haemoglobin concentration; IVIG, intravenous immunoglobulin; KD, Kawasaki disease; L, lymphocyte count; M, monocyte count; NCAA, no coronary artery aneurysm; N, neutrophil count; PA, prealbumin; PLT, platelet count; PNI, prognostic nutritional index; SD, standard deviation; TB, total bilirubin; WBC, white blood cell count.
Table 4
Laboratory parameters | CAA group (n=28) |
NCAA group (n=94) |
P value | Normal group (n=12) |
Persistent CAA group (n=16) | P value |
---|---|---|---|---|---|---|
WBC (×109/L) | 8.1 (7.0, 11.0) | 9.0 (6.9, 11.2) | 0.88 | 7.9 (6.9, 9.4) | 9.1 (7.0, 11.4) | 0.29 |
N (×109/L) | 2.7 (1.9, 4.2) | 2.8 (2.1, 4.1) | 0.91 | 2.6±1.2 | 3.5±1.8 | 0.14 |
L (×109/L) | 4.5 (3.8, 5.7) | 4.3 (3.1, 5.8) | 0.38 | 4.6±1.2 | 5.0±1.8 | 0.50 |
M (×109/L) | 0.7 (0.5, 1.0) | 0.6 (0.5, 0.8) | 0.68 | 0.7 (0.5, 0.8) | 0.7 (0.6, 1.1) | 0.29 |
Hb (g/L) | 111.3±12.7 | 1107.7±11.9 | 0.18 | 110.8±13.3 | 111.8±12.7 | 0.84 |
PLT (×1012/L) | 382.7 (304.3, 499.1) | 414.5 (326.8, 532.3) | 0.22 | 376.0 (298.0, 520.0) | 382.7 (304.3, 456.0) | 0.96 |
CRP (mg/L) | 10.0 (10.0, 10.0) | 10.0 (10.0, 10.0) | 0.34 | 10.0 (10.0, 10.0) | 10.0 (10.0, 10.0) | 0.20 |
Data are presented as mean ± SD, or median (interquartile range). CAA, coronary artery aneurysm; CRP, C-reactive protein; Hb, haemoglobin concentration; KD, Kawasaki disease; L, lymphocyte count; M, monocyte count; N, neutrophil count; NCAA, no coronary artery aneurysm; PLT, platelet count; SD, standard deviation; WBC, white blood cell count.
Threshold values of some selected indicators
There is no uniform definition of PA and PNI. Thus, this study used ROC curves to determine the threshold values of PA and PNI. PA values were missing from three patients in the CAA group and eight patients in the NCAA group. In the CAA group, there were 23 patients with a PA value of more than 107.2 mg/L and two patients with a PA value of less than 107.2 mg/L. Then in the NCAA group, there were 60 patients with a PA value of more than 107.2 mg/L and 26 patients with a PA value of less than 107.2 mg/L. Besides, three patients in the CAA group and six patients in the NCAA group lacked PNI values. In the CAA group, there were 18 patients with a PNI value of more than 54.7 and seven patients with a PNI value of less than 54.7. Then in the NCAA group, there were 42 patients with a PNI value of more than 54.7 and 46 patients with a PNI value of less than 54.7. Based on Little’s Missing Completely at Random (MCAR) test, we obtained a P value of 0.930, indicating that our data satisfy the MCAR assumption, meaning the missing data is entirely random. The missing data for PA >107.2 mg/L and PNI >54.7 fall under MCAR and can be directly ignored. In terms of predicting KD with CAA, the area under the ROC curve (AUC) of PA >107.2 mg/L (determined by Youden’s index) after IVIG was 0.544, with a sensitivity of 92% and a specificity of 30.2%; and the AUC of PNI >54.7 (determined by Youden’s index) after IVIG was 0.556, with a sensitivity of 72% and a specificity of 52.3%. This meant that a post-IVIG PA >107.2 mg/L and a post-IVIG PNI >54.7 are of fair values in predicting KD with CAA. As shown in Table 5, there were more patients with PA >107.2 mg/L (P=0.01) after IVIG and PNI >54.7 (P=0.03) after IVIG in the CAA group.
Table 5
Threshold values | Sensitivity (%) | Specificity (%) | AUC (95% CI) | CAA group (n=28) | NCAA group (n=94) | P value |
---|---|---|---|---|---|---|
PA >107.2 mg/L after IVIG | 92 | 30.2 | 0.544 (0.418–0.670) | 23/25 (92.0) | 60/86 (69.8) | 0.01 |
PNI >54.7 after IVIG | 72 | 52.3 | 0.556 (0.422–0.690) | 18/25 (72.0) | 42/88 (47.7) | 0.03 |
Data are presented as number (percentage) unless otherwise stated. AUC, area under the receiver operating characteristic curve; CAA, coronary artery aneurysm; CI, confidence interval; IVIG, intravenous immunoglobulin; KD, Kawasaki disease; NCAA, no coronary artery aneurysm; PA, prealbumin; PNI, prognostic nutritional index.
Results of the multivariable logistic analysis
In this study, binary logistic regression analysis was performed to identify risk factors in KD with CAA. As there were few significantly different indicators in the difference analysis, the indicators with P<0.10 were included in the multivariable analysis in this study. Ccr had collinearity with Cys C; thus, it was excluded. Ultimately, the variables that were entered into the multivariate logistic regression analysis included polymorphous exanthem and changes in the extremities, PCT and Cys C before IVIG, PA >107.2 mg/L and PNI >54.7 after IVIG. There was no collinearity between the five parameters that were analysed. Besides, outcome events per predictor variable (EPV) was 5.6 (28 CAAs/5 variables) which was within acceptable levels of 5–10 EPV based on the simulation study of Vittinghoff and McCulloch (26). The Cys C before IVIG [odds ratio (OR), 10.183; 95% confidence interval (CI): 1.158–89.525] was finally deemed as the independent risk factor for developing CAA among KD patients (Table 6). Due to the small sample size of patients with CAA, the risk factors for persistent CAA in KD could not be identified by multivariate logistic analysis.
Table 6
Indicators with P<0.10 in the difference analysis | Univariable | Multivariable | VIF | |||
---|---|---|---|---|---|---|
Odds ratios (95% CI) | P value | Odds ratios (95% CI) | P value | |||
Polymorphous exanthem | 0.418 (0.175–1.000) | 0.050 | 0.410 (0.136–1.238) | 0.11 | 1.076 | |
Changes in the extremities | 0.350 (0.122–1.004) | 0.051 | 0.751 (0.212–2.656) | 0.66 | 1.208 | |
Cys C before IVIG | 8.667 (1.364–55.079) | 0.02 | 10.183 (1.158–89.525) | 0.04 | 1.051 | |
PCT before IVIG | 0.034 (0.001–2.052) | 0.11 | 0.014 (0.000–2.835) | 0.12 | 1.076 | |
PA >107.2 mg/L after IVIG | 4.983 (1.094–22.703) | 0.04 | 3.899 (0.663–22.926) | 0.13 | 1.297 | |
PNI >54.7 after IVIG | 2.816 (1.070–7.415) | 0.04 | 2.310 (0.707–7.543) | 0.17 | 1.283 |
CAA, coronary artery aneurysm; CI, confidence interval; Cys C, cystatin C; IVIG, intravenous immunoglobulin; KD, Kawasaki disease; PA, prealbumin; PCT, plateletocrit; PNI, prognostic nutritional index; VIF, variance inflation factor.
Predictive value of Cys C for KD with CAA
For predicting CAA development in KD, the contribution of Cys C was calculated by ROC curve. The 0.71 Cys C cut-off value (determined by Youden’s index) had an 88.9% sensitivity and a 35.6% specificity (AUC =0.626, 95% CI: 0.503–0.750, P=0.048, Figure 2). For the specific sampling-time to predict CAA development, Cys C values could not be calculated per day due to the small size. In fact, the analysis was carried out with a fever cut-off of five days. When sampling within five days from fever onset, significant variations were found in Cys C levels between patients with and without CAA in KD (1.02±0.31 vs. 0.80±0.22 mg/L, P=0.03, Figure 3) (power value 0.384), but there were no significant differences between them when sampling beyond five days (0.88±0.30 vs. 0.81±0.19 mg/L, P=0.24, Figure 3). In addition, the 0.71 Cys C cut-off value sampled within five days had a 100% sensitivity and a 42.9% specificity (AUC =0.752, 95% CI: 0.577–0.928, P=0.03, Figure 2). Besides, we made a comparison with established predictive scores such as Kobayashi (27) (AUC =0.522, 95% CI: 0.399–0.646, P=0.72, Figure 2), Egami (28) (AUC =0.544, 95% CI: 0.425–0.662, P=0.48, Figure 2), and Sano (29) risk scores (AUC =0.521, 95% CI: 0.397–0.644, P=0.74, Figure 2).
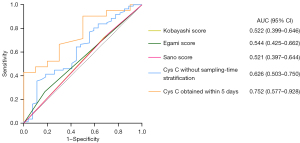
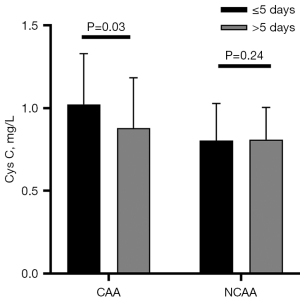
Discussion
Acquired heart diseases in children are increasingly being caused by CAA, which is a severe cardiac consequence of KD (23). As a result, a focus of research on KD is now investigating the risk factors for CAA development. In this study, the incidence of CAA development in KD patients was 23.0%, which is comparable to the typical range in China (20.6%) (30). All patients with KD included in this study received early intervention in accordance with the 2017 AHA guidelines (23) and the sixth revision of Japanese diagnostics guide (24), and there were no statistically significant differences in days at diagnosis, days at primary treatment and total fever days. However, in developed countries such as Europe and the United States, CAL is not observed as frequently in patients with KD who receive appropriate treatment. This could be attributed to the significant role of environmental factors and genetic background in the pathogenesis of KD development. Environmental factors such as geography, climate, water quality, atmosphere, living conditions, dietary habits, and lifestyle may be related to the pathogenesis of KD. Especially in the case of the continuously increasing incidence in East Asia, environmental problems caused by pollutants in the process of industrialization should be concerned. Furthermore, the elevated incidence rate observed in East Asian populations implies that racial and genetic factors may contribute to the pathogenesis of KD. Therefore, the research on genetic susceptibility needs to be emphasized. A study from Beijing Children’s Hospital (31) pointed out that certain gene polymorphisms such as ITPKC and CD40 may be involved in the formation of CAA. Furthermore, a previous study of our team (32) demonstrated that the ITPKC rs7251246 T>C polymorphism might be a risk factor for the formation of CAA in the Han Chinese in southwest China. The above research findings have provided us with a deeper understanding of the genetic susceptibility of children with KD, and have provided a theoretical basis for pediatricians to take more active treatment for children with a susceptibility to KD.
In this study, the BMI and the proportion of small CAAs of the group that achieved CAA regression was significantly higher. To some extent, our study further confirmed that the frequency of CAA regression varies greatly depending on the severity of coronary arteries and nutritional status. Recent clinical studies from several countries [European: La Vecchia’s team (33), Japanese: Iio’s team (34) and USA: Son’s team (35)] have shown that KD patients with a high Z score at diagnosis (Z-score +2 or +2.5 or higher) have an independent risk of developing CAA. Besides, our study indicated that a higher BMI level, which represented a better nutritional status at admission, may contribute to the prognosis of CAAs. In addition to paying attention to the BMI at admission, children with CAAs would tend to consciously reduce their physical activity, which is very likely to cause an increase in BMI in the later stage of the disease. Therefore, we should also pay attention to evaluating the changes in BMI during the follow-up period.
As for the laboratory features before and after treatment, our study identified that a higher Cys C level before IVIG was the independent risk factors in KD with CAA. Patients with KD sometimes present trace proteinuria and sterile pyuria. However, kidney and urinary severe involvement of KD is uncommon (36). First in 2003, a study of Gupta-Malhotra’s team (22) showed that the Cys C levels of children with KD (n=17) were significantly decreased compared to patients without infections (n=18, eight adults and 10 children). In 2020, Junji Azuma and his colleagues (13) found that the maximum value of urinary β2-microglobulin before IVIG treatment was significantly associated with CALs in KD, which has linked KD to kidney and urinary involvement. However, as a serum renal function indicator, the connection between Cys C and CAA has received little attention. Cys C is generated by all nucleated cells; therefore, it is expected to be greater under situations of rapid cell turnover, such as inflammatory disorders. According to Emil Carlsson’s study (37), Cys C was crucial for immunological control and viral and microbial infection resistance. As a systemic vasculitis, Cys C could be elevated in KD patients, especially those with CAA information. Much less, the onset of KD cannot currently rule out infectious factors. CRP levels were not significantly different between the CAA and NCAA groups, nor between the normal group and the persistent CAA group in this study. Therefore, we speculated that the systemic inflammatory levels among the subgroups of KD were comparable. According to a 10-year follow-up cohort study which included 7,745 patients aged 31 to 75 years, Cys C was associated with long-term cardiovascular mortality risk (38). Besides, higher Cys C is related to major cardiac adverse events (39). According to a case-control study among pediatric patients, Cys C may have an impact on the process of myocardial remodeling through suppressing the activity of endogenous cysteine protease (21). Therefore, for children with KD who had a higher level of Cys C, when coronary artery inflamed, the coronary artery remodeling process may be inhibited, thereby resulting in the formation of CAAs. In some extent, the result of our study that Cys C levels increased in CAA patients, was inconsistent with Monesha Gupta-Malhotra’s. In addition to racial, regional, and genetic factors, the small sample size may contribute to the inconsistency. These investigations highlighted the potential involvement of Cys C in the progression of cardiovascular diseases, including coronary artery complications in KD.
In the current work, we retrospectively examined the predictive value of sampling-time specific Cys C for CAA patients in KD. Cys C may be a promising predictor for the development of CAA in KD, even though its predictive value in KD with CAA was insufficient. Encouragingly, after sampling-time stratification, it was found that, Cys C ≥0.71 obtained within five days after fever, may prove to be a useful predictor of CAA development in KD, which had a high sensitivity (100%) and a relatively low specificity (42.9%), as evidenced by a moderate AUC (0.752) in our study. Based on our work, evaluating serum Cys C concentration within five days of fever may help predict the formation of CAA. Given its high sensitivity (100%) and low specificity (42.9%), Cys C could work as a potential supplementary predictor in the acute phrase of KD to reduce the underdiagnosis of CAA, for in the echocardiography, the coronary arteries are usually normal in the acute phrase and the lesions of coronary arteries appear in the subacute period. KD patients within five days of disease onset with a level of Cys C more than 0.71 mg/L may require closer monitoring for the formation of CAAs at the one-month follow-up. To provide better insight into the value of Cys C, a comparison with established predictive scores (Kobayashi, Egami, and Sano risk scores) was made. However, the predictive values of the above scoring were not so ideal due to racial and regional factors. Junji Azuma’s model (13) combined urinary β2-microglobulin with other five indicators (age, gender, IVIG-refractory, frequency of steroid pulse therapy, CRP level) and was robust with a sensitivity and specificity of 73% and 99%. However, urine-related protein testing was not performed in the KD patients of our retrospective study. It may be an inspiration that combining urine-related proteins or other indicators in further prospective research to improve the robustness of the model. The relevance of sampling-time-specific (for example, day 1, day 2, …, day 10) Cys C cut-off values towards the prediction of CAA was exactly what we were interested in. Due to the small sample size (CAA group) and some missing data, we roughly divided the sampling time of Cys C into within five days and more than five days instead, which prohibited us from further exploring the association between the value of sampling-time-specific Cys C and CAA and may influence the estimation of P value (power value 0.384). At least, for CAA development in KD patients, these results offer crucial proof of the differences between the prediction values by the same parameter. However, to predict CAA in KD patients, future randomized controlled trials are required to determine various Cys C cut-off values based on sampling-times. To confirm our findings, a large sample size from various populations and fundamental research are also necessary.
Notably, significant differences were observed in polymorphous exanthem, changes in the extremities and Ccr levels between the CAA group and NCAA group, suggesting that individuals with KD who had lower Ccr or lacked the clinical manifestation of polymorphous exanthem and changes in the extremities may be more susceptible to developing CAA. As both are indicators of renal function, why could Cys C be a risk factor for KD with CAA after multivariate regression analysis, while Ccr only demonstrated statistical significance in the difference test? Actually, for most of children with KD included in this study, their renal function tests before initial IVIG treatment were also conducted in the clinical laboratory of our institution, whose Ccr was calculated from Cys C [Ccr = (80.35/Cys C) − 4.32]. The remaining part of patients with KD had their renal function evaluated in other medical institutions at the early stage of the disease. This might partially explain the loss of the statistical power of Ccr. This finding of Ccr is in line with previous research (40), which observed slight renal function impairment in some children with KD. Uncertainty surrounds the underlying causes of renal failure in KD patients, which may be brought on by dehydration, inflammatory mediators, infectious agents, or a mix of them. Luckily, in the children with KD included in this study, dehydration was not observed. If there was renal function impairment, the possibility of prerenal kidney injury was small. Therefore, based on our observations, KD patients with investigations that show lower Ccr and higher Cys C, or without the clinical manifestation of polymorphous exanthem and changes in the extremities, need constant monitoring and more frequent follow-ups. We need more research as we learn more about the pathophysiology of CAA development and regression to better comprehend KD.
There are some limitations in this study as follows. First, a selection bias could have occurred because of our retrospective study and the small sample from a single institution, which could limit the relevance of our findings to other racial populations. Second, the lack of a gold standard for diagnosing KD may have led to incorrect classification of participants, due to the possibility that some of the individuals in our research with incomplete KD had another condition which was extremely similar to KD instead of KD that cannot be fully ruled out. This is especially important during the coronavirus disease 2019 pandemic because multisystem inflammatory syndrome in children (41) has several clinical characteristics of KD. By carefully excluding patients who had other infectious diseases with a more probable cause, multisystem inflammatory syndrome for example, we hoped to reduce the probability of misdiagnosis. Besides, we have not conducted fundamental research on the relationship between Cys C, KD, and CAA. Finally, due to the small sample size and the lack of data throughout the sampling period, the risk factors for persistent CAA in KD could not be identified by multivariate logistic analysis and the statistical power was constrained. Thus, our results should be interpreted cautiously. To validate our findings, additional research including large sample sizes, a variety of demographics and basic research are needed in the future.
Conclusions
Higher pre-IVIG Cys C levels may be associated with increased CAA risk, but further large-scale prospective studies are needed to confirm its predictive utility. Furthermore, Cys C acquired within five days of disease onset may theoretically provide better prediction power for the CAA development in KD.
Acknowledgments
We thank Yong Han, Cheng Chen, Yanyun Huang, Suyuan Qin, Dongli Liu, Yuqin Huang, and Piaoliu Yuan from the Pediatrics Department of The First Affiliated Hospital of Guangxi Medical University for their advice and manuscript preparation assistance.
Footnote
Reporting Checklist: The authors have completed the STARD reporting checklist. Available at https://tp.amegroups.com/article/view/10.21037/tp-2024-516/rc
Data Sharing Statement: Available at https://tp.amegroups.com/article/view/10.21037/tp-2024-516/dss
Peer Review File: Available at https://tp.amegroups.com/article/view/10.21037/tp-2024-516/prf
Funding: This study was supported by
Conflicts of Interest: All authors have completed the ICMJE uniform disclosure form (available at https://tp.amegroups.com/article/view/10.21037/tp-2024-516/coif). Y.P. received fundings from the Guangxi Medical and Health Key Discipline Construction Project [No. 2019 (19)] and Guangxi Clinical Research Center for Pediatric Disease (No. GUI KE AD22035219). The other authors have no conflicts of interest to declare.
Ethical Statement: The authors are accountable for all aspects of the work in ensuring that questions related to the accuracy or integrity of any part of the work are appropriately investigated and resolved. This research was approved by the Medical Ethic Committee of The First Affiliated Hospital of Guangxi Medical University [No. 2021(KY-E-240)]. The study was conducted in accordance with the Declaration of Helsinki and its subsequent amendments. An informed consent was obtained from the parents of each subject.
Open Access Statement: This is an Open Access article distributed in accordance with the Creative Commons Attribution-NonCommercial-NoDerivs 4.0 International License (CC BY-NC-ND 4.0), which permits the non-commercial replication and distribution of the article with the strict proviso that no changes or edits are made and the original work is properly cited (including links to both the formal publication through the relevant DOI and the license). See: https://creativecommons.org/licenses/by-nc-nd/4.0/.
References
- Odingo M, Rutter M, Bowley J, et al. The incidence of Kawasaki disease using hospital admissions data for England 2006-2021. Rheumatology (Oxford) 2023;62:3117-25. [Crossref] [PubMed]
- Watts RA, Hatemi G, Burns JC, et al. Global epidemiology of vasculitis. Nat Rev Rheumatol 2022;18:22-34. [Crossref] [PubMed]
- Jone PN, Tremoulet A, Choueiter N, et al. Update on Diagnosis and Management of Kawasaki Disease: A Scientific Statement From the American Heart Association. Circulation 2024;150:e481-500. [Crossref] [PubMed]
- Daniels LB, Gordon JB, Burns JC. Kawasaki disease: late cardiovascular sequelae. Curr Opin Cardiol 2012;27:572-7. [Crossref] [PubMed]
- Banday AZ, Bhattacharya D, Pandiarajan V, et al. Kawasaki disease in siblings in close temporal proximity to each other-what are the implications?. Clin Rheumatol 2021;40:849-55. [Crossref] [PubMed]
- Nakamura A, Ikeda K, Hamaoka K. Aetiological Significance of Infectious Stimuli in Kawasaki Disease. Front Pediatr 2019;7:244. [Crossref] [PubMed]
- Kanai T, Ishiwata T, Kobayashi T, et al. Ulinastatin, a urinary trypsin inhibitor, for the initial treatment of patients with Kawasaki disease: a retrospective study. Circulation 2011;124:2822-8. [Crossref] [PubMed]
- Sawada M, Ogino K, Hayashi T, et al. Therapeutic plasma exchange for refractory Kawasaki disease in children weighing less than 10 kg. Ther Apher Dial 2024;28:424-31. [Crossref] [PubMed]
- Miura M, Kobayashi T, Kaneko T, et al. Association of Severity of Coronary Artery Aneurysms in Patients With Kawasaki Disease and Risk of Later Coronary Events. JAMA Pediatr 2018;172:e180030. [Crossref] [PubMed]
- Kobayashi T, Ayusawa M, Suzuki H, et al. Revision of diagnostic guidelines for Kawasaki disease (6th revised edition). Pediatr Int 2020;62:1135-8.
- Hu J, Ren W. Analysis of the Risk Factors in Prognosis of Kawasaki Disease With Coronary Artery Lesions. Front Pediatr 2021;9:798148. [Crossref] [PubMed]
- Wang Y, Wang W, Gong F, et al. Evaluation of intravenous immunoglobulin resistance and coronary artery lesions in relation to Th1/Th2 cytokine profiles in patients with Kawasaki disease. Arthritis Rheum 2013;65:805-14. [Crossref] [PubMed]
- Azuma J, Yamamoto T, Nitta M, et al. Structure equation model and neural network analyses to predict coronary artery lesions in Kawasaki disease: a single-centre retrospective study. Sci Rep 2020;10:11868. [Crossref] [PubMed]
- Ndumele CE, Neeland IJ, Tuttle KR, et al. A Synopsis of the Evidence for the Science and Clinical Management of Cardiovascular-Kidney-Metabolic (CKM) Syndrome: A Scientific Statement From the American Heart Association. Circulation 2023;148:1636-64. [Crossref] [PubMed]
- Rangaswami J, Bhalla V, Blair JEA, et al. Cardiorenal Syndrome: Classification, Pathophysiology, Diagnosis, and Treatment Strategies: A Scientific Statement From the American Heart Association. Circulation 2019;139:e840-78. [Crossref] [PubMed]
- Abid L, Charfeddine S, Kammoun S, et al. Cystatin C: A prognostic marker after myocardial infarction in patients without chronic kidney disease. J Saudi Heart Assoc 2016;28:144-51. [Crossref] [PubMed]
- Xiong H, Wang L, Jin F, et al. Association of cystatin C with coronary artery calcification in patients undergoing multidetector computed tomography. Medicine (Baltimore) 2021;100:e26761. [Crossref] [PubMed]
- Sugiyama H, Miyoshi T, Osawa K, et al. Serum cystatin C levels are associated with coronary artery calcification in women without chronic kidney disease. J Cardiol 2017;70:559-64. [Crossref] [PubMed]
- Teragawa H, Oshita C, Akazawa R, et al. Clinical characteristics and prognosis in patients with multi-vessel coronary spasm–comparison with those in patients with single-vessel coronary spasm. European Heart Journal 2021;42:ehab724.1073.
- Lee SN, Shin DI, Jung MH, et al. Impact of cystatin-C level on the prevalence and angiographic characteristics of vasospastic angina in Korean patients. Int Heart J 2015;56:49-55. [Crossref] [PubMed]
- Hanna D, Beshir M, Khalifa N, et al. Assessment of cystatin C in pediatric sickle cell disease and β-thalassemia as a marker of subclinical cardiovascular dysfunction: a case-control study. Pediatr Hematol Oncol 2021;38:620-32. [Crossref] [PubMed]
- Gupta-Malhotra M, Levine DM, Cooper RS, et al. Decreased levels of cystatin C, an inhibitor of the elastolytic enzyme cysteine protease, in acute and subacute phases of kawasaki disease. Cardiology 2003;99:121-5. [Crossref] [PubMed]
- McCrindle BW, Rowley AH, Newburger JW, et al. Diagnosis, Treatment, and Long-Term Management of Kawasaki Disease: A Scientific Statement for Health Professionals From the American Heart Association. Circulation 2017;135:e927-99. [Crossref] [PubMed]
- Fukazawa R, Kobayashi J, Ayusawa M, et al. JCS/JSCS 2020 Guideline on Diagnosis and Management of Cardiovascular Sequelae in Kawasaki Disease. Circ J 2020;84:1348-407. [Crossref] [PubMed]
- Kobayashi T, Fuse S, Sakamoto N, et al. A New Z Score Curve of the Coronary Arterial Internal Diameter Using the Lambda-Mu-Sigma Method in a Pediatric Population. J Am Soc Echocardiogr 2016;29:794-801.e29. [Crossref] [PubMed]
- Vittinghoff E, McCulloch CE. Relaxing the rule of ten events per variable in logistic and Cox regression. Am J Epidemiol 2007;165:710-8. [Crossref] [PubMed]
- Kobayashi T, Inoue Y, Takeuchi K, et al. Prediction of intravenous immunoglobulin unresponsiveness in patients with Kawasaki disease. Circulation 2006;113:2606-12. [Crossref] [PubMed]
- Egami K, Muta H, Ishii M, et al. Prediction of resistance to intravenous immunoglobulin treatment in patients with Kawasaki disease. J Pediatr 2006;149:237-40. [Crossref] [PubMed]
- Sano T, Kurotobi S, Matsuzaki K, et al. Prediction of non-responsiveness to standard high-dose gamma-globulin therapy in patients with acute Kawasaki disease before starting initial treatment. Eur J Pediatr 2007;166:131-7. [Crossref] [PubMed]
- Xie LP, Yan WL, Huang M, et al. Epidemiologic Features of Kawasaki Disease in Shanghai From 2013 Through 2017. J Epidemiol 2020;30:429-35. [Crossref] [PubMed]
- Guo K, Qiu L, Xu Y, et al. Single-Nucleotide Polymorphism LncRNA AC008392.1/rs7248320 in CARD8 is Associated with Kawasaki Disease Susceptibility in the Han Chinese Population. J Inflamm Res 2021;14:4809-16. [Crossref] [PubMed]
- Liu J, Yuan P, Pang Y, et al. ITPKC polymorphism (rs7251246 T > C), coronary artery aneurysms, and thrombosis in patients with Kawasaki disease in a Southern Han Chinese population. Front Immunol 2023;14:1184162. [Crossref] [PubMed]
- La Vecchia A, Stracquadaino R, Mauri L, et al. Risk factors and scores for prediction of coronary artery aneurysms in Kawasaki disease: a European monocentric study. BMC Pediatr 2024;24:139. [Crossref] [PubMed]
- Iio K, Morikawa Y, Miyata K, et al. Risk Factors of Coronary Artery Aneurysms in Kawasaki Disease with a Low Risk of Intravenous Immunoglobulin Resistance: An Analysis of Post RAISE. J Pediatr 2022;240:158-163.e4. [Crossref] [PubMed]
- Son MBF, Gauvreau K, Tremoulet AH, et al. Risk Model Development and Validation for Prediction of Coronary Artery Aneurysms in Kawasaki Disease in a North American Population. J Am Heart Assoc 2019;8:e011319. [Crossref] [PubMed]
- Watanabe T. Kidney and urinary tract involvement in kawasaki disease. Int J Pediatr 2013;2013:831834. [Crossref] [PubMed]
- Carlsson E, Supharattanasitthi W, Jackson M, et al. Increased Rate of Retinal Pigment Epithelial Cell Migration and Pro-Angiogenic Potential Ensuing From Reduced Cystatin C Expression. Invest Ophthalmol Vis Sci 2020;61:9. [Crossref] [PubMed]
- Stewart RAH, Robledo KP, Tonkin AM, et al. Plasma Protein Biomarkers and Long-Term Cardiovascular Mortality Risk in Patients With Chronic Coronary Heart Disease. J Am Heart Assoc 2024;13:e034367. [Crossref] [PubMed]
- Budano C, Andreis A, De Filippo O, et al. A single cystatin C determination before coronary angiography can predict short and long-term adverse events. Int J Cardiol 2020;300:73-9. [Crossref] [PubMed]
- Sanchez C, Bujdud EM, Martinez RO. Kawasaki Disease and Acute Kidney Injury in Pediatric Patients. Indian J Pediatr 2021;88:167-8. [Crossref] [PubMed]
- Consiglio CR, Cotugno N, Sardh F, et al. The Immunology of Multisystem Inflammatory Syndrome in Children with COVID-19. Cell 2020;183:968-981.e7. [Crossref] [PubMed]