Externally applied compression therapy for Fontan patients
Introduction
Pediatric and adult patients having single ventricle physiology are a small subset of patients with complex cardiac defects and congenital heart disease (CHD) (1). The current treatment paradigm for these patients involves a set of staged surgical cardiac procedures. These surgeries result in the creation of a Fontan physiology where the right side of the heart is bypassed and the single functioning ventricle draws and drives blood flow through the systemic and pulmonary circulations, respectively (Figure 1). These patients live with a compromised circulatory system and can develop late stage pathophysiology in the months and years after the Fontan operation, generally leading to a decompensation to congestive heart failure (CHF) (1,3). Significant progress in pharmacologic and novel surgical treatments has occurred over the years. However, new therapeutic options are needed since heart transplantation is a limited option due to donor numbers, pre-existing antibodies due to prior blood product exposure, and the waiting period (4,5).
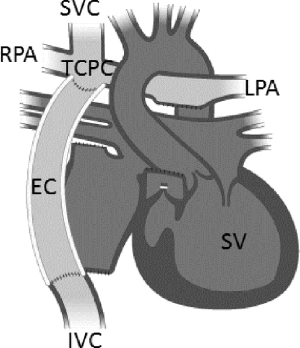
There is growing interest in the use of mechanical circulatory assistance for Fontan patients (6). By introducing a pressure boost to the pulmonary circulation analogous to the native right ventricle, it is conceivable that the deleterious complications of the Fontan palliation might be reversed, and the circulation might revert to more normal physiologic levels (7,8). Although mechanical circulatory support devices have been shown to mitigate the failing Fontan physiology prior to heart transplantation, current research indicates device success as only a short term bridge-to-transplant therapy, not for use over an extended period of time (9-11). Additionally, these devices require an invasive, open-heart procedure for implementation, and no clinically available device to-date has been designed to specifically augment the unique circulatory demands of a Fontan physiology. Although cavopulmonary assist devices are currently under development, all are far from reaching clinical use (4,12).
To address this unmet clinical need, we are investigating the feasibility of a non-invasive, at-home therapy to improve hemodynamic circulation and quality of life for these patients. Our hypothesis is that regularly applied, external compression therapy will augment systemic blood flow to the pulmonary arteries and improve cardiac output. The purpose of this research study is to evaluate the effectiveness of applying external pressure to the patient’s legs using a NormaTec pneumatic compression device (PCD) (NormaTec, Inc. Newton Center, MA, USA) and to compare at-home therapeutic findings to an exercise study. We measured how externally applied pressure over a 1-week time-period may improve cardiovascular health. This study involved three stages, which included an initial evaluation, at-home compression treatment using the NormaTec device for one week, and then a post-treatment treadmill test.
Methods
NormaTec pneumatic compression trousers
The NormaTec PCD (NormaTec, Inc.) has received U.S. Food and Drug Administration 510(k) clearance (PMA #013436) for use to treat patients having venous insufficiency, which Fontan patients exhibit as they decompensate to CHF. Figure 2 illustrates this device as composed of inflatable trouser compartments that facilitate circumferentially and uniformly applied pressure to a patient’s lower extremities. A controller is easily programmed to a target externally applied pressure level and to a rate of inflation and deflation of the trouser compartments, facilitating improved venous return, as displayed in Figure 3. Other programmable parameters include the duration of compression cycles and rest periods before compression cycles. The trousers have 5 separate compartments in which compression is initiated in the feet (i.e., compartment 1) and ends at the top of the thighs. For subjects with an inseam measurement less than 30 inches, only the lower 4 of the 5 compartments are required for successful compression treatment.
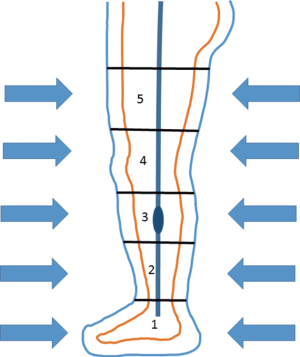
Compression study methods
The Institutional Review Board (HM# 13521) at Virginia Commonwealth University (VCU) reviewed and approved of this pilot clinical study. Figure 4 describes the clinical study methods and timeline of events with respect to the compression therapy as well as pre-study and post-study cardiorespiratory measurements. Two study subjects (n=2) were identified by pediatric cardiologists at the VCU Medical Center for enrollment (Table 1). Following an initial health screening, neither of the subjects met any of the exclusion criteria. After receiving informed consent, the test subjects were pre-evaluated with a treadmill exercise stress test based on a modified-Bruce protocol, and relevant cardiorespiratory baseline data was collected. They were then fitted and trained on how to use the NormaTec PCD at-home. The subjects returned to their residences and began their at-home compression treatment for 6 consecutive days.

Full table
Prior to initiation of compression, a baseline blood pressure reading was taken of the subject. The level of external pressure applied during compression was set to a pressure of +15 mmHg above the measured diastolic pressure. Subjects performed the compression treatments daily for 140 minutes on each of the first 4 days at-home and then 280 minutes over the last 2 days at-home based on the discretion of the subject. Full compression cycle time consisted of 30 minutes with a rest period of 5 minutes between each set. Each compression cycle began with inflation of the trouser compartment around the feet and ankles, following inflation of the next compartment and deflation of prior compartment in order to move blood volume back toward the heart; compression rates from lowest compartment 1 to 4 took approximately 165 seconds. Four compression cycles were completed to constitute a day of treatment. A member of the study team was present to assist with the compression treatments each day. On day 7, after the last at-home compression treatment, the subjects returned to the VCU Medical Center for a post-study evaluation, which involved a second treadmill exercise stress test and collection of another set of relevant cardiorespiratory data for comparison to the pre-study levels.
Results
Data from two test subjects are displayed in Table 2. Both subjects demonstrated the following: (I) an improvement in exercise duration time, peak oxygen volume, and ventilation threshold; (II) an ability to exercise to exhaustion without adverse events; and (III) a 10% improvement their total exercise duration time based on a peak respiratory exchange rate (RER peak) ≥1.0 as indicative of maximal performance. During the graded stress test, oxygen consumption was measured to increase linearly as exercise intensity increased. In general, total aerobic capacity (VO2 peak) was achieved at a lower level than normally found in a healthy subject with a biventricular circulation. No cardiopulmonary or vascular complications were noted by either subject. Neither subject complained of a headache that could have been contributed by retrograde blood flow into the SVC. Also, neither subject complained of any discomfort during the study.
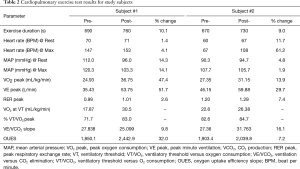
Full table
In consideration of the first subject, Figure 5 illustrates the RER as a function of time for the pre- and post-compression treatment stress test. As seen in Figure 5, the RER peak for the first subject occurred slightly after the exercise phase. A positive impact from the compression treatment was measured due to the significant improvement in aerobic capacity (47%), as shown in Figure 6. Since the RER peak was not reached until after the exercise phase, it was difficult to discern if this measured improvement is due primarily to increased exercise duration or the 6-day long compression treatment.
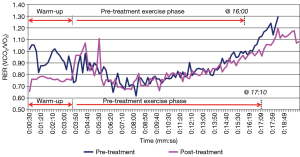
The second subject was able to achieve maximal exercise levels during the stress test. The RER peak occurred at 8 minutes and 20 seconds during the pre-treatment test (170 seconds before the end of the exercise phase) and at 9 minutes during the post-treatment test (190 seconds before the end of the exercise). A modest improvement in VO2 peak occurred for the second subject, increasing capacity by 13.9%, as shown in Figure 7.
Ventilatory threshold (VT) is an important parameter to measure as it can be used to predict the ability of a subject to sustain a given work rate for a prolonged period without metabolic (lactic) acidosis. In our study, we measured VT noninvasively by analyzing the continuous measurements of ventilatory and gas exchange variables during the stress test. Since peak respiratory exchange rate was only reached after the exercise test for the first subject, it is a not a reliable indication of improved exercise capacity for this subject. The second subject was able to achieve maximal exercise levels during testing, and thus the maximal exercise parameters were included in the analysis of the second subject. Although the heart rate response was blunted, positive trends in the ventilation threshold data were observed.
Ventilatory equivalent ratios were measured to assess if the subjects reached their anaerobic threshold later after going through the treatment regimen. Here we investigated the point where a marked change in slope occurred between VE/VO2 and VE/VCO2. Before treatment this threshold was reached around 8 minutes and 10 seconds into the stress test. This VO2 of 22.6 mL/kg/min represents 82.6% of the VO2 peak, pre-treatment. By the end of the week, the subjects were able to reach their aerobic capacity later, around 8 minutes and 50 seconds, improving their time by 8%. The subjects increased the VO2 at which VT occurred by 3.7 mL/kg/min. The first subject demonstrated a modest improvement in VE/VCO2 slope of 9.8%. The second subject had an increase of VE/VCO2 slope after the compression therapy of 16%.
Post-treatment, both subjects were found to achieve more efficient oxygen consumption as indicated by a steeper slope in the oxygen uptake efficiency slope (OUES). OUES measurements were started at 90 seconds until 90% of the test phase had been reached (13). The first subject experience an increase in OUES of approximately 32%, and the second subject showed an increase as well, although to a lesser degree (7.2%).
Discussion
The absence of the right ventricle as a preloader for the systemic circulation leads to a reduced pulmonary blood volume being driven through the lungs to the left atrium. This condition leads to chronically elevated central venous pressure, which is a hallmark of the Fontan physiology and contributes to limited systemic venous return, liver congestion and cardiac arrhythmias (14,15). Following the Fontan surgery, single ventricle patients have a lower exercise capacity and tolerance than individuals with two ventricles. At rest, these patients will have normal or slightly subnormal levels of cardiac index, stroke index, and systolic blood pressure as compared to healthy subjects. However, during dynamic exercise, notable differences are observed, showing that these patients are unable to adequately respond to exercise.
Beyond adolescence, exercise tolerance continues to fall at a rate of about 2.6% per year. It is well known that during intense exercise Fontan patients reach exhaustion much faster than their healthy counterparts with a biventricular circulation. Respiratory and ventilation rates are known to spike as they exhale more CO2 and reach anaerobic metabolism much faster. This effect is primarily driven by a low cardiac output response during intense exercise resulting in a lower VO2 max among Fontan patients. They have a limited ability to increase stroke volume with exercise, due to impaired ventricular function and an inability to increase ventricular preload exercise (16). Cardiac Index has been measured to be 22% lower in Fontan patients than those with healthy biventricular circulations (14); in consideration of older adults with Fontan physiology, clinical reports indicate myocardial dysfunction and Grade II failure in approximately 70% of patients (15). As Fontan patients age, the single ventricle becomes dilated, hypertrophic, and hypocontractile, resulting in systolic and diastolic dysfunction. When exercise capacity crosses a threshold of about 45% of predicted, as typically occurs in the third decade of life, hospitalization rates and symptoms increase significantly.
Given the known studies on limited exercise tolerance of Fontan patients and clinical experience in the evaluation and treatment of Fontan patients, we sought to evaluate the benefit of at-home compression treatments on the cardiorespiratory function and capacity of Fontan patients. By introducing a pressure boost to the pulmonary circulation similar to the native right ventricle, it is conceivable that the deleterious complications of the Fontan palliation might be reversed. We hypothesize that regularly applied external compression therapy will enhance systemic venous return to the pulmonary arteries and improve left atrial return volume. This study demonstrated an improvement in exercise capacity after compression therapy in two subjects. There are, however, several limitations of this pilot study. For a compelling case of improvement to be made, additional study subjects are required, and an appropriate statistical evaluation of the pre-treatment and post-treatment measurements are needed with sufficient power.
There is an inherent bias in the exercise protocol that seems to favor subject experience with the equipment. It is known that the majority of test subjects tend to perform better the second time on the treadmill. The first study subject did not reach the RER peak of greater than 1.0 during the treadmill study, which means that it is unreliable to use the change in VO2 peak as an indication of increased exercise capacity. This result has been observed in other external compression studies and exercise studies, including Fontan patients. Feldman et al. (17) showed in a 6 month follow up that subjects who received a treatment regimen of 35 1-hour sessions over a period of 7–8 weeks using enhanced external counterpulsation (EECP) technology were able to improve their exercise time, although peak VO2 did not improve with therapy. Both Bossers et al. (13) and Akkerman et al. (18) report that finding VO2 Max is limited to healthy adult subjects who can actually perform maximally during a graded exercise stress test. Subjects must continually push themselves long enough for VO2 to reach the plateau in the face of increasing workload. Both studies indicate that in the pediatric population and Fontan patients a true plateau in oxygen uptake is seldom attained. Bossers et al. (13) conducted a large multi-center study with 110 Fontan patients and showed that as much as 20% of the subjects were not able to reach a maximal exercise state (RER ≥1.00), and thus sub-maximal exercise parameters were used in the evaluation (e.g., VE/VCO2 slope and OUES slope).
Although the second subject was able to perform at a sufficient level of effort, as indicated by RER peak, there was still no clear plateau of VO2, thus no conclusion can be drawn for certain that a true VO2 max level was reached. Hence, it was essential to analyze sub-maximal exercise parameters, which included VE/VCO2 slope, VT, and OUES. These parameters do show, particularly OUES, an improvement in exercise capacity after compression treatment. OUES improved by 32% and 7.2% for the first and second subject, respectively. Larger studies have shown an average VE/VCO2 slope for Fontan patients to be around approximately 127% greater than their healthy counterparts (13). This observation is in agreement with the results seen in this OUES analysis, and these two subjects seem to be relatively healthy Fontan patients. As measured, an elevated slope is expected from CHD patients since an elevated VE/VCO2 slope is inversely related to cardiac output. In the Fontan population, a diminished pulmonary perfusion also contributes to this elevated slope state. As cardiorespiratory measurements improve with long-term pneumatic compression therapy, a reduction in VE/VCO2 slope is expected.
VT was also measured noninvasively by analyzing the continuous measurements of ventilatory and gas exchange variables during the stress test. VT for CHD patients occurs closer to VO2 max/peak than does for healthy subjects; the VT/VO2 ratios for CHD subjects are higher than healthy subjects (67.6% vs. 44.6%) (19). At rest, these patients have near normal ventilation (9–10 L/min); however, during peak exercise, the minute ventilation rate is significantly less than that of normal subjects (20).
Due to a scheduling conflict, the second subject was not able to perform the post-treatment stress test immediately following the last day of treatment. The post-treatment stress test was performed three days after the last day of treatment. Although not the primary reason, this could have caused some variation between the two subjects results post treatment. Nevertheless, both subjects showed an improvement in exercise duration time, peak oxygen volume, and ventilation threshold, as compared to the baseline evaluation. These findings are promising and provide the foundation for future studies that will focus on increasing study participation (sample size) to better assess the clinical benefit of compression therapy for Fontan patients.
Conclusions
A consistent attenuation of the chronic venous insufficiency found in the Fontan patients is required to avoid long-term complications, such as liver congestion, cardiac arrhythmias, and other co-morbidities. Limited therapeutic options exist to achieve this attenuation. This study describes the initial evaluation of an alternative, non-invasive, at-home therapeutic option for Fontan patients. Routinely administered, externally applied compression treatments to the lower extremities is expected to augment systemic venous return, improve ventricular preload, and thus enhance cardiac output in Fontan patients. Two subjects received at-home compression therapy for 6 days for which the cardiovascular benefit was evaluated using an exercise stress test. Both subjects demonstrated an improvement in exercise duration time, peak oxygen volume, and ventilation threshold, as compared to the baseline evaluation. The findings of this study provide the foundation for future studies of at-home external compression therapy for Fontan patients.
Acknowledgements
The authors wish to acknowledge the generous financial support for this work as provided by the Frederick Banting Foundation in Richmond, Virginia. The clinical study was performed at the Virginia Commonwealth University, and authorship contributions to data processing, analysis, and article composition occurred at affiliated locations as indicated.
Footnote
Conflicts of Interest: Joseph Hernandez is now the Director of Research for the Frederick Banting Foundation. The other authors have no conflicts of interest to declare.
Ethical Statement: The Institutional Review Board (HM# 13521) at Virginia Commonwealth University (VCU) reviewed and approved of this pilot clinical study and written informed consent was obtained from all patients.
References
- Khairy P, Ionescu-Ittu R, Mackie AS, et al. Changing mortality in congenital heart disease. J Am Coll Cardiol 2010;56:1149-57. [Crossref] [PubMed]
- Aahus University Hospital: Aahus University; 2015 [cited 2015 03/07]. Available online: http://www.auh.dk/om-auh/afdelinger/center-for-medfodte-hjertesygdomme/born-med-medfodt-hjertesygdom/medfodt-hjertesygdom/univentrikulare-hjerter-og-fontan--eller-tcpc-operation/
- de Leval MR, Deanfield JE. Four decades of Fontan palliation. Nat Rev Cardiol 2010;7:520-7. [Crossref] [PubMed]
- Wilmot I, Lorts A, Morales D. Pediatric Mechanical Circulatory Support. Korean J Thorac Cardiovasc Surg 2013;46:391-401. [Crossref] [PubMed]
- VanderPluym C, Urschel S, Buchholz H. Advanced therapies for congenital heart disease: ventricular assist devices and heart transplantation. Can J Cardiol 2013;29:796-802. [Crossref] [PubMed]
- Jayakumar KA, Addonizio LJ, Kichuk-Chrisant MR, et al. Cardiac transplantation after the Fontan or Glenn procedure. J Am Coll Cardiol 2004;44:2065-72. [Crossref] [PubMed]
- Rodefeld MD, Frankel SH, Giridharan GA. Cavopulmonary assist: (em)powering the univentricular fontan circulation. Semin Thorac Cardiovasc Surg Pediatr Card Surg Annu 2011;14:45-54. [Crossref] [PubMed]
- Prêtre R, Häussler A, Bettex D, et al. Right-sided univentricular cardiac assistance in a failing Fontan circulation. Ann Thorac Surg 2008;86:1018-20. [Crossref] [PubMed]
- Lee JR, Choi JS, Kang CH, et al. Surgical results of patients with a functional single ventricle. Eur J Cardiothorac Surg 2003;24:716-22. [Crossref] [PubMed]
- Throckmorton AL, Ballman KK, Myers CD, et al. Mechanical cavopulmonary assist for the univentricular Fontan circulation using a novel folding propeller blood pump. ASAIO J 2007;53:734-41. [Crossref] [PubMed]
- Ravishankar C, Gaynor J. Mechanical support of the functionally single ventricle. Cardiol Young 2006;16:55-60. [Crossref] [PubMed]
- Kanani M, Hsia TY. Options for the failing ventricle in pediatric heart disease. Curr Cardiol Rep 2013;15:404. [Crossref] [PubMed]
- Bossers SS, Helbing WA, Duppen N, et al. Exercise capacity in children after total cavopulmonary connection: lateral tunnel versus extracardiac conduit technique. J Thorac Cardiovasc Surg 2014;148:1490-7. [Crossref] [PubMed]
- Gewillig MH, Lundström UR, Bull C, et al. Exercise responses in patients with congenital heart disease after Fontan repair: patterns and determinants of performance. J Am Coll Cardiol 1990;15:1424-32. [Crossref] [PubMed]
- Walker F. Pregnancy and the various forms of the Fontan circulation. Heart 2007;93:152-4. [Crossref] [PubMed]
- Shachar GB, Fuhrman BP, Wang Y, et al. Rest and exercise hemodynamics after the Fontan procedure. Circulation 1982;65:1043-8. [Crossref] [PubMed]
- Feldman AM, Silver MA, Francis GS, et al. Enhanced external counterpulsation improves exercise tolerance in patients with chronic heart failure. J Am Coll Cardiol 2006;48:1198-205. [Crossref] [PubMed]
- Akkerman M, van Brussel M, Bongers BC, et al. Oxygen uptake efficiency slope in healthy children. Pediatr Exerc Sci 2010;22:431-41. [Crossref] [PubMed]
- Ohuchi H, Kagisaki K, Miyazaki A, et al. Impact of the evolution of the Fontan operation on early and late mortality: a single-center experience of 405 patients over 3 decades. Ann Thorac Surg 2011;92:1457-66. [Crossref] [PubMed]
- Driscoll DJ, Danielson GK, Puga FJ, et al. Exercise tolerance and cardiorespiratory response to exercise after the Fontan operation for tricuspid atresia or functional single ventricle. J Am Coll Cardiol 1986;7:1087-94. [Crossref] [PubMed]