Ophthalmic manifestations of endocrine disorders—endocrinology and the eye
Disorders of the pancreas
Diabetes mellitus (DM)
The estimated global prevalence of DM was 422 million in 2014 (1). According to projections by the Centers for Disease Control and Prevention, this number will continue to rise, and by 2050, one in three adults may have DM (2). Patients with DM have an increased risk of both macro- and micro-vascular disease, such as coronary artery disease, peripheral arterial disease, and stroke, as well as nephropathy, neuropathy, and retinopathy (1). With regard to the eye, diabetes is the leading cause of blindness among adults under 75 years of age (3). Moreover, the presence of ophthalmic disease, specifically diabetic retinopathy (DR) has significant predictive value for cardiovascular disease and mortality in patients with DM, which is why early recognition and treatment of ophthalmic disease is so critical (4).
Pathophysiology of ophthalmic manifestations
Diabetic eye disease refers to a wide spectrum of ophthalmic complications that affect individuals with DMs. When organized according to different segments of the eye, these conditions include retinal pathology such as DR, macular edema, and ophthalmic vascular disease, as well as anterior segment pathology such as poor corneal healing and cataracts.
Retinal complications of DM are primarily attributed to the microvascular effects of chronic hyperglycemia. Hyperglycemia mediates retinal injury through impaired regulation of retinal blood flow, as well as accumulation of sorbitol and advanced glycosylation end products in the retinal vascular endothelial cells. Impaired endothelial cell function and loss of pericytes leads to accumulation of extracellular fluid in the retinal tissue, as well as increased levels of vascular endothelial growth factor (VEGF) and other vasoproliferative factors that lead to abnormal retinal vascularization (5-7). DR is classically differentiated into two major entities: non-proliferative DR (NPDR) and proliferative DR (PDR). In the earliest stage of NPDR, hyperglycemia results in the formation of nerve-fiber layer infarcts and capillary microaneurysms, which are clinically detected by the presence of cotton-wool spots and small spots of intraretinal blood, respectively. Rupture of the weak-walled capillary microaneurysms leads to larger areas of intraretinal hemorrhage in the superficial or deep layers of the retina, detected as flame-shaped and dot-blot hemorrhages, respectively. Disease progression compromises the integrity of the retinal blood vessels, leading to breakdown of the blood-retina barrier and subsequent leakage of serum protein and lipids into the retina. These changes constitute hard exudates, which present as well-demarcated, yellow-white deposits, often observed at the border of retinal edema (Figure 1). Over time, inadequate oxygenation of the retina causes release of growth factors, such as insulin-like growth factor 1 (IGF-1) and VEGF, in an effort to re-vascularize diseased zones. This characterizes the progression from non-proliferative to PDR (Figures 2,3). The new, abnormal blood vessels, which were formed to combat retinal ischemia, become the source of vision-threating disease. Not only are they fragile and susceptible to leakage, but they develop not only in the vitreous and retina, but also in segments of the eye such as the trabecular meshwork, a structure responsible for drainage of aqueous humor and maintenance of normal intraocular pressure. The combination of anomalous blood vessels in abnormal locations results in blinding complications such as neovascular glaucoma, which can permanently damage the optic nerve, and tractional retinal detachment due to the formation of fibrovascular scar tissue (Figure 4) (11,12). Excessive vascular permeability, as observed in DR, occurs throughout the retina, but when it occurs in the macula (the central region of the retina responsible for keen central vision), it can cause significant visual morbidity if not treated quickly and effectively (11,12). Lastly, it is well known that DM increases the risk of certain ophthalmic vascular disorders, specifically central retinal vein occlusions (CRVOs). Although the exact pathogenesis of retinal vein occlusions is unclear, it is hypothesized that venous stasis and hypercoagulability due to hyperglycemia and abnormal endothelial cell function cause venous thrombosis (13). The extent of vision loss after such a thrombotic event is dependent on the degree of residual retinal perfusion and the presence of associated macular edema (14).
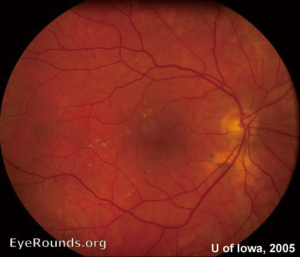
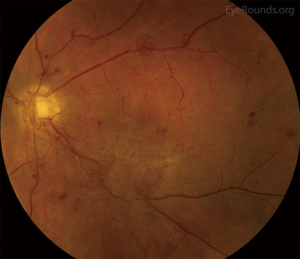
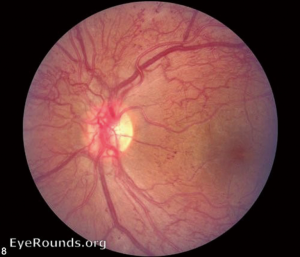
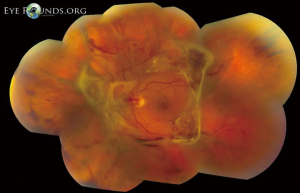
Although the retinal complications of DM are associated with the greatest risk of irreversible vision loss, hyperglycemia can also affect the anterior segment of the eye. Excess glucose in the eye can lead to cataract formation. A variety of studies support the central role of aldose reductase in initiating this process, as it is the enzyme that catalyzes the reduction of glucose to sorbitol. Excess sorbitol accumulates within the lens fiber cells. The subsequent shift in the intracellular osmotic balance leads to lens fiber edema, which ultimately leads to rupture of the lens fibers, and diabetic cataract formation (15). Additionally, just as hyperglycemia can affect peripheral nerve function in the hands and feet, poorly controlled DM can damage the peripheral cranial nerves that innervate the extraocular muscles and cornea. Of the four cranial nerves that innervate the extraocular muscles, the abducens or sixth cranial nerve, is most commonly affected, resulting in debilitating double vision and new onset strabismus. When the trigeminal or fifth cranial nerve is affected, corneal sensation is reduced, since the nasociliary branch of the ophthalmic branch (V1) innervates the surface of the cornea and plays an indirect role in corneal healing.
Clinical presentation and relevance
DR is a potentially blinding complication that is observed in over 90% and 60% of patients with type 1 DM and type 2 DM, respectively, 20 years after diagnosis (16). In patients with high-risk PDR, the 5-year risk of severe vision loss may be as high as 60% (17). Individuals with DR may complain of decreased visual acuity, loss of parts of their visual field, new-onset flashes, and floaters. While signs and symptoms may be worse in one eye, individuals typically have bilateral involvement due to the systemic nature of this condition (18). In patients with clinically significant macular edema (CSME), the risk of moderate vision loss is approximately 25–30% over a 3-year time frame (17). Macular edema may occur at any point during the natural course of DR and is the primary driver of vision loss in NPDR (18). Among individuals with diabetes, cataracts are observed at a higher frequency and demonstrate a younger age of onset (12). Patients with cataracts tend to notice deficits more commonly during nighttime and may complain of increased glare. The field of vision may become blurry or faded, halos may appear around lights, and vertical lines may become distorted, bent, or wavy (18). Paralysis of the sixth cranial nerve comprises up to one half of all cranial nerve palsies encountered among patients with poorly controlled diabetes (19). As this nerve is responsible for innervating the lateral rectus muscle, patients may present with an inability to abduct the eye, resulting in esotropia (medial deviation of the eye) and binocular diplopia. Young children with sixth nerve palsy may not complain of diplopia but compensate for loss of abduction by rotating their head to the affected side (20).
Since many of the ophthalmic findings of diabetic disease may predate the onset of ocular symptoms, early and regular ophthalmologic examinations is paramount. It is recommended that patients with DM have annual complete eye exams, including evaluation of visual acuity, pupillary response, extraocular muscle movement, anterior segment exam, and complete dilated fundus examination. Any patient identified to have ocular pathology is then monitored closely with more frequent exams as determined by their ophthalmologist.
Treatment
Two landmark studies, the Diabetes Control and Complications Trial (DCCT) and the United Kingdom Prospective Diabetes Study (UKPDS), demonstrated that glycemic control is essential in the prevention of ocular manifestations associated with DM. In the DCCT trial, subjects with T1DM were randomized to receive either intensive or conventional insulin therapy. Although the incidence of DR was similar between the two groups three years from study onset, intensive therapy decreased DR incidence by 50%, DR progression by 50%, and risk of peripheral neuropathy by 60% after 5 years (21). Similarly, the UKPDS, an investigation of intensive versus conventional glucose control among patients with T2DM, showed a 25% decrease in microvascular events, with additional embedded studies underscoring the importance of not only tight glycemic control, but also tight blood pressure regulation in minimizing the risk of micro- and macro-vascular complications (22). In addition to metabolic control, multiple clinical trials have demonstrated the efficacy of agents targeting the renin-angiotensin system and peroxisome proliferator-activated receptor α (PPAR-α) in the medical management of DR (11).
Although prevention is the best strategy for managing ophthalmic complications of DM, treatment of retinal disease has improved drastically with the advent of pan-retinal photocoagulation (PRP), intravitreal anti-VEGF agents, and improved retinal surgical techniques. These modalities, when used appropriately, can maximize visual potential in patients with high risk PDR, CSME, and venous occlusions (23-27). For those patients with visually significant cataracts affecting visual function and activities of daily living, cataract extraction with intraocular lens placement is a well-established option with generally good outcomes, although patients with diabetes are at higher risk for intraoperative and post-operative complications (18,28).
Finally, patients affected by cranial nerve palsies due to diabetic neuropathy typically improve within 3–6 months. In those cases with an atypical course, residual symptoms, or persistently poor extraocular motility, additional work-up and/or strabismus surgery are often indicated.
The pediatric perspective
Although most pediatric patients diagnosed with diabetes have type 1 disease, the growing obesity epidemic is rapidly changing this statistic. In the US alone, estimates are as high as 5,000 new cases per year of youth-onset type 2 diabetes, with a strong correlation with lower socioeconomic status and race (29). Moreover, it has been shown that type 2 DM in adolescents is a different disease than that seen in adults, with a more rapid decline in functioning B-cells and accelerated development of diabetic complications (30). Nonetheless, the ophthalmic complications of both type 1 and type 2 DM are well understood, and are drivers of early screening and intervention in diabetic patients of all ages. Current guidelines established by the American Academy of Pediatrics, American Academy of Ophthalmology, and American Diabetes Association recommend annual screening for T1DM patients beginning at age 10, or 3–5 years after diagnosis, whichever comes later, with annual follow up thereafter (31). Although there are no established guidelines or data regarding ophthalmic screening for pediatric patients with ype 2 disease, it can be extrapolated that a similar approach be taken to minimize the risk of vision loss in this population.
Disorders of the thyroid gland
Graves’ disease
Graves’ disease is the most common cause of hyperthyroidism worldwide, with an annual incidence of 20–50 cases per 100,000 persons (32). This disorder has a strong propensity for females and is typically diagnosed in the third and fourth decades of life. As with most autoimmune conditions, there is often a strong familial component, and Graves’ has been linked to a variety of human leukocyte antigens (HLA), including HLA-B8 and -DR3 in Caucasians and HLA Bw35 in Asians. Systemic symptoms of hyperthyroidism include heat intolerance, fatigue, and anxiety. Physical signs in a patient with Graves’ disease often include sweating, tremor, a diffuse goiter, weight loss, digital clubbing, pretibial myxedema, and numerous cardiovascular changes, including tachycardia, arrhythmias, and bounding peripheral pulses (33). Similarly, with regard to the eye and orbit, patients can present with a variety of signs and symptoms encompassed within the term Graves’ ophthalmopathy, which will be discussed below. It is important to note, however, that although 90% of patients with Graves’ disease are hyperthyroid, a smaller subset of patients may be euthyroid or even hypothyroid, indicating that thyroid function tests alone are insufficient to make the diagnosis of Graves’ disease (34).
Pathophysiology of ophthalmic manifestations
Graves’ disease is characterized by the presence of activating IgG autoantibodies that target the thyrotropin receptor (34). Following the evasion of immune system regulation, these antibodies stimulate thyroid hormone production independent of molecular feedback mechanisms (32). The clinical features observed in Graves’ ophthalmopathy are attributed to increased hyaluronan production by orbital fibroblasts following activation by inflammatory cytokines and thyroid-stimulating immunoglobulins. Subsequent hyaluronan deposition leads to expansion and pathologic changes in various ocular tissues including the orbital fat and extraocular muscles (34). Although immunoglobulins are fundamental to the pathophysiology of Graves’ disease, there is no direct association between levels of thyroid-stimulating immunoglobulins and serum thyroid hormone concentrations in patients with Graves’ disease (35). Thus, thyroid status itself cannot in isolation make a diagnosis of Grave’s disease, or serve as a marker of the severity of ophthalmic disease.
Clinical presentation and relevance
Thyroid eye disease is the most common systemic manifestation of Graves’ disease, seen in up to 50% of Graves’ patients (36). Often, patients initially seek medical attention because of cosmetically unacceptable proptosis, eyelid swelling, the “wide-eyed stare” and symptomatic diplopia (32). The diagnosis of Graves’ ophthalmopathy is confirmed when eyelid retraction, exophthalmos, restricted extraocular motility, strabismus, or optic nerve dysfunction is noted in the presence of thyrotropin-activating IgG auto-antibodies (37). Among ophthalmic abnormalities linked to Graves’ disease, eyelid retraction is present at some point in the course of disease in 90% of patients, with other ophthalmic signs and symptoms associated with variable frequency (Table 1) (38).
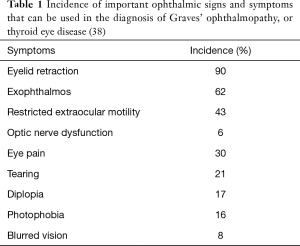
Full table
As a result of increased adrenergic activity of the levator palpebrae superioris muscle, eyelid movement disorders may present as eyelid retraction in primary gaze (Dalrymple sign) or as delayed descent of the upper eyelids in downgaze (von Graefe sign) (3). Exophthalmos, used exclusively to describe the proptosis of Graves’ ophthalmopathy, can predispose patients to exposure keratopathy and corneal ulceration (Figure 5) (34). Extraocular muscle infiltration clinically manifests as restricted ocular motility and diplopia (33). The inferior and medial recti muscles are the most commonly and earliest affected, but all six extraocular muscles can become involved (Figure 6) (33). Compressive optic neuropathy presents as decreased visual acuity, afferent pupillary defect, loss of color vision, or blurred disc margins detected on funduscopic examination (33). Other ocular features of Graves’ ophthalmopathy include conjunctival chemosis, eyelid swelling, keratoconjunctivitis sicca, and superior limbic keratoconjunctivitis (32). The severity of active ophthalmopathy waxes and wanes, but typically lasts up to 3 years before burning out (32). However, the severe inflammation associated with the active phase of the disease mediates substantial fibrotic remodeling within the orbit and the extraocular muscles, which results in permanent changes in the function and appearance of ocular structures. Thus, many ophthalmic features associated with Graves’ ophthalmology often never truly regress (33,41).
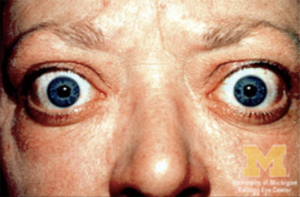
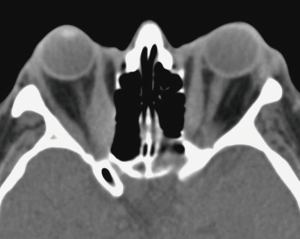
Treatment
Management of Graves’ disease is multi-faceted and complex. Anti-thyroid drugs (ATDs), radioactive iodine ablation therapy, and/or thyroid surgery can be implemented individually or in combination with one another to facilitate restoration of a euthyroid state. In some cases, oral steroids and/or external beam orbital radiation are often necessary to minimize associated orbital inflammation. Lastly, it is well known that cigarette smoking is associated with a higher degree of disease severity and a lower effectiveness of medical treatment (36). Thus, smoking cessation is the most important lifestyle modification a Graves’ patient can make to manage their disease and risk of ophthalmic complications. Although ATDs are associated with a durable remission in up to 50% of patients, they are linked with a high risk of recurrence after withdrawal of treatment. Radioactive iodine therapy has been a staple in the management of hyperthyroidism management for several decades and often results in a rapid reduction of hyperthyroid symptoms. However, studies show that it can cause progression of ophthalmopathy in nearly 15% of patients, especially those that are high risk, although this can be mitigated by the use of oral steroids (32,36). Lastly, surgical management may be most beneficial for patients with large goiters, those who prefer surgical to medical therapy, and women who may become pregnant in close proximity to treatment (32).
Treatment for Graves’ ophthalmopathy depends on the phase and severity of the disease, with the vast majority of patients requiring conservative measures only (32). Typically, ophthalmologists recommend aggressive ocular lubrication as a first-line measure to protect against corneal decompensation from exposure keratopathy due to impaired eyelid function and exophthalmos. Additionally, sunglasses may help reduce photophobia, and elevating the head of the bed at night may reduce the degree of periorbital edema observed in the morning (3). However, those with more significant ophthalmic complications of Graves’ disease may require surgical intervention. The most invasive of these is orbital decompression surgery, which is performed for those with vision-threatening compressive optic neuropathy. Strabismus surgery can treat debilitating diplopia and is typically recommended only once the active phase of the disease has ended. A variety of surgical procedures can be performed on the eyelids to address appearance and function, and minimize the risk of vision loss due to exposure keratopathy (32).
Pediatric perspective
Graves’ disease is the most common cause of hyperthyroidism in the pediatric population, with an annual incidence of 0.1 cases per 100,000 children and 3.0 cases per 100,000 adolescents (42). However, ocular manifestations of Graves’ disease among pediatric patients are relatively infrequent and not typically associated with significant morbidity (43). Nonetheless, signs such as eyelid retraction or exophthalmos should be evaluated for in pediatric patients with otherwise non-specific signs and symptoms to potentially support a diagnosis of Graves’ disease. Conversely, pediatric patients with the disease should still be monitored for ophthalmic complications, however mild.
Disorders of the hypothalamic-pituitary axis
Pituitary tumors
Two main types of tumors can occupy the pituitary fossa. Craniopharyngiomas, which originate from remnants of Rathke’s pouch, account for up to 90% of pediatric tumors arising in the pituitary region, and are the most common pediatric neoplasm associated with hypopituitarism in children (44). Pituitary adenomas, on the other hand, comprise only 2.7% of supratentorial tumors in children, and are usually benign, and classified according to size, functional status, primary cell origin, and hormone secreted (growth hormone, adrenocorticotropic hormone, thyroid stimulating hormone, and the gonadotropin hormones) (44). Prolactinomas are the most common pituitary adenomas in adults and children, with 53% of pediatric pituitary adenomas identified as prolactinomas (44,45). These masses are typically diagnosed through neurologic manifestations of mass effect and intracranial expansion, or as distinct syndromes characterized by clinical features of hormone over- or under-secretion.
Pathophysiology of ophthalmic manifestations
The pituitary gland is located in the sella turcica, also known as the pituitary fossa, which is a saddle-shaped depression on the upper surface of the sphenoid bone. The optic chiasm, containing the optic nerves and tracts, typically lies superior to the pituitary gland and anterior to the pituitary stalk (33). However, the chiasm may lie anterior (also termed a prefixed chiasm) or posterior (postfixed chiasm) to the gland in 15% or 5% of cases, respectively (17). The nasal fibers of the retina, which carry visual information from the temporal visual fields, run in the medial aspect of the optic nerves and then decussate across the chiasm to the contralateral side of the optic tracts. The temporal fibers of the retina, which carry visual information from the nasal visual fields, run laterally and remain on the ipsilateral side of the optic tracts (Figure 7). On either side of the pituitary fossa laterally lie the cavernous sinuses, which each contain the ipsilateral oculomotor (III), trochlear (IV), and abducens (VI) cranial nerves, ophthalmic (V1) and maxillary (V2) branches of the trigeminal cranial nerve, and internal carotid artery (33). It is this anatomic configuration that dictates the ocular manifestations associated with pituitary disorders.
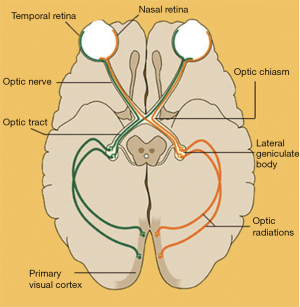
Clinical presentation and relevance
Visual symptoms of a pituitary mass are usually the result of mechanical compression of the optic nerves, chiasm, or tracts (41). Although the prevalence of ophthalmologic features associated with pituitary pathology is decreasing as a result of earlier recognition of accompanying endocrine complaints and an increase in intracranial imaging performed for other indications, visual symptoms remain a key presenting feature of non-functioning and gonadotrophic adenomas (17,45). Abnormalities of the visual field are the most well known visual symptom associated with pituitary tumors. The classic bitemporal hemianopsia occurs due to pituitary tumor enlargement and subsequent compression of the optic chiasm from below. Initially, the inferior fibers of the optic nerves that run medially in the chiasm and carry the visual information from the superior and temporal fields are damaged earliest, resulting in early loss of the superotemporal quadrants (17). Continued compression eventually damages the superior fibers of the optic nerve on both sides of the chiasm, leading to a complete bitemporal hemianopsia. In patients with a prefixed chiasm, optic tract compression may lead to incongruous homonymous hemianopia. In contrast, those with a postfixed chiasm may present with decreased visual acuity, reduced color vision, or a contralateral junctional scotoma (33). In contrast, craniopharyngiomas, which are typically suprasellar in origin, classically present with inferotemporal quadrantanopsia due to compression of the optic chiasm centrally from above (47). If visual field defects progress undetected by patient or physician, patients may complain of an insidious decrease in vision, despite good visual acuity as tested in the office, often leaving patient and physician flummoxed. Such findings underscore the importance of routine visual field testing to gross confrontation, and the use of perimetry, when available. Ultimately, chronic nerve compression by a pituitary mass results in diffuse, irreversible atrophy of the optic nerve which is typically associated with severe vision and field loss (17). Lateral extension of a pituitary fossa mass into the cavernous sinuses can affect the cranial nerves contained therein, accounting for partial or complete ophthalmoplegia, diplopia, ptosis, pupillary anomalies, and facial pain (17). Such expansion of a neoplasm into the sinuses is typically a feature of aggressive and rapidly growing tumors, and is usually associated with a poor prognosis (33).
Treatment
A reduction in the size, and thus mass effect, of pituitary tumors is generally accomplished via surgical resection, radiation therapy, or medical management. For patients with pituitary adenomas, postoperative improvements in ophthalmologic symptoms are noted in nearly all patients with a pre-surgical visual acuity of at least 20/100 and in approximately 60% of remaining patients (17). Although bromocriptine, a dopamine agonist, has demonstrated efficacy in reducing the size of prolactinomas, medical agents are less efficacious in the treatment of growth hormone-secreting adenomas and ACTH-secreting adenomas (45). The probability of recurrence following treatment is directly proportional to the size of the tumor (33). With regard to craniopharyngiomas, treatment generally comprises of a combination of surgical resection, radiation therapy, and/or cyst drainage (48).
Pediatric perspective
Although craniopharyngiomas are the most common pediatric tumor of the pituitary region, they are uncommon overall, accounting for only 6% of pediatric intracranial tumors (44,48). Older studies reported a 20% mortality for individuals with craniopharyngiomas at 5 years from diagnosis, but more recent reports indicate that the 5- and 10-year survival rates exceed 90% regardless of treatment type (48,49).
Septo-optic dysplasia (SOD)
SOD is a rare congenital disorder, with an estimated incidence of 1 in 10,000 live births. It is characterized by the presence of two or more of the following abnormalities: hypothalamic-pituitary endocrine deficiencies, optic nerve hypoplasia (ONH), and midline brain anomalies (Figure 8) (51). The most commonly reported features include hypopituitarism, typically manifesting as growth hormone deficiency, visual impairment, and developmental delay, although any of the pituitary hormones can be deficient (52). Approximately 30% of SOD cases present with the classic triad of features (53).
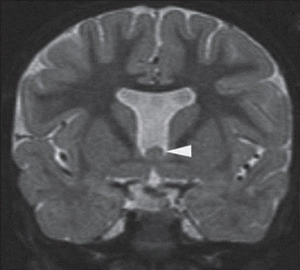
Pathophysiology of ophthalmic manifestations
At present, the etiology of SOD remains unclear. However, given the neurologic abnormalities associated with this condition, genetic and environmental factors that impact early forebrain development, which occurs at 4–6 weeks of gestation, likely play a role. Several studies have identified antenatal substance abuse and young maternal age as risk factors (51). Moreover, mutations in genes crucial for development, including HESX1, SOX2, and SOX3, have been linked to this disorder (54).
Clinical presentation and relevance
ONH is present in about 80% of cases of SOD and may be the initial presenting feature of this disorder, with endocrine abnormalities often manifesting later. Bilateral optic nerve involvement is observed in 88% of patients, although patients with unilateral ONH should also be evaluated for SOD (Figure 9) (54). Affected children typically present to a pediatrician’s or ophthalmologist’s office with family members concerned about possible blindness, misaligned eyes, or nystagmus (53). Importantly, the severity of visual impairment often varies widely, with some patients demonstrating no light perception vision, and others maintaining close to 20/40 vision. Early diagnosis of this syndrome is crucial as untreated visual and endocrine abnormalities can worsen neurodevelopmental outcomes (51).
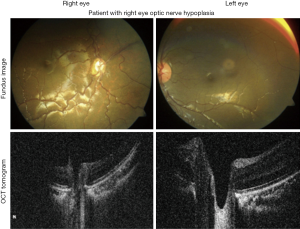
Treatment
Treatment of SOD requires a multi-disciplinary approach, with input from neurology, endocrinology, and ophthalmology. Management includes identification and replacement of all hormone deficiencies as well as management of co-existing ophthalmic disease to maximize a child’s visual potential. Affected patients require ongoing follow-up and close monitoring of neurodevelopmental status and associated developmental delays (51).
Pediatric perspective
Almost all cases of SOD are diagnosed within the first year of life, with a nearly equivalent number of male and female cases (51). Endocrinologists should monitor patients with SOD on a long-term basis as endocrine abnormalities may present later in life. The prevalence of associated hypothalamic-pituitary dysregulation has been shown to be approximately 60% (56).
Hereditary endocrine syndromes
Multiple endocrine neoplasia (MEN)
MEN is a group of distinct, autosomal dominant disorders involving the endocrine glands. A diagnosis of MEN type 1 (MEN1) includes the presence of tumors in at least two of the following locations: parathyroid glands, pituitary glands, and gastro-entero-pancreatic tract (57). Among these, parathyroid tumors are the most common tumor overall and prolactinomas are the most common type of pituitary tumor (58). MEN types 2A (MEN2A) and 2B (MEN2B), on the other hand, have an increased risk of medullary thyroid carcinoma and pheochromocytoma. Additional characteristic features in MEN2A and MEN2B include hyperparathyroidism and Marfanoid habitus with mucosal neuromas, respectively (59).
Pathophysiology of ophthalmic manifestations
The gene MEN1 encodes a protein called Menin, a putative tumor suppressor. However, the exact mechanisms of this protein in tumorigenesis of MEN type 1 are unknown (58). The vast majority of MEN2 cases are associated with gain of function mutations in the RET protooncogene (59).
Clinical presentation and relevance
Although patients with both types of MEN syndromes are typically diagnosed based on non-ophthalmic findings and a strong known family history of disease, in new cases, a detailed ocular exam may direct the work-up and differential diagnosis. Visual field defects secondary to pituitary adenomas (see “Pituitary Tumors”) represent the majority of ocular findings in MEN1 (58). Patients with a diagnosis of MEN2A and MEN2B may demonstrate prominent corneal nerves. However, mucosal neuromas on the eyelid or conjunctiva are unique to MEN2B, and can cause thickening and eversion of the eyelids as well as displacement of the eyelashes (59-61). Additional features associated with MEN2 include prominent eyebrows and impaired pupillary dilation (61). Patients with these findings should be thoroughly evaluated by their primary care physicians and referred to endocrinology, given the mortality associated with these rare genetic syndromes.
Treatment
Given the heterogeneous clinical presentation of MEN syndromes, treatment is managed on a case-by-case basis and is beyond the scope of this review (57,61).
Pediatric perspective
Most patients with either type of MEN are diagnosed in adulthood, although there are reports of medullary thyroid carcinoma associated with MEN2 presenting in infancy (62). Given the autosomal dominant inheritance pattern of MEN, an extended family history and additional testing may be indicated when the index of suspicion for MEN is high.
Wolfram syndrome
Wolfram syndrome is a rare autosomal recessive condition characterized by the presence of DMs, diabetes insipidus, optic atrophy, and deafness (DIDMOAD). Additional endocrine associations include ACTH deficiency and growth hormone deficiency (52). DMs is usually the presenting feature, diagnosed around 6 years of age (63). The prevalence of cases with all four components of the acronym DIDMOAD is only between 14% and 58%, although the onset of each component varies over time. Accordingly, any pediatric patient diagnosed with urinary incontinence and diabetes should be evaluated for DIDMOAD, as the urinary incontinence may be an occult sign of diabetes insipidus. This slowly progressive disorder carries a poor prognosis, with a median age at death of 30 years, generally due to central apnea secondary to brainstem atrophy, as well as neuropsychiatric disease (64).
Pathophysiology of ophthalmic manifestations
Wolfram syndrome has been linked to mutations in the gene WFS1. This gene encodes wolframin, a protein that plays a regulatory role in calcium homeostasis in the endoplasmic reticulum (65). Immunohistochemical studies of the human retina have demonstrated wolframin expression in retinal ganglion cells, optic axons, and the optic nerve. Dysfunction of wolframin in these locations may explain the ophthalmologic findings associated with this syndrome (64,66).
Clinical presentation and relevance
Children with Wolfram syndrome present with bilateral optic nerve atrophy around 11 years of age. Initially, ocular manifestations are mild and include loss of color and peripheral vision. However, visual symptoms progress to blindness within approximately eight years of diagnosis of atrophy (64). Progression of the disorder can be reliably correlated with retinal thinning (63). Less common ophthalmologic features include cataracts, retinal pigmentation, DR, glaucoma, abnormal pupillary reflexes, and nystagmus (64).
Treatment
To date, no known treatments exist for the ophthalmic complications of Wolfram syndrome. However, idebenone and docosahexaenoic acid have shown promise as potential management strategies to delay disease progression (63). However, given the spectrum of ophthalmic complications, as well as neurologic, neuropsychiatric, and endocrine manifestations, early diagnosis and supportive care is paramount.
Pediatric perspective
Wolfram syndrome is an exceedingly rare congenital disorder where DM and diabetes insipidus can coexist with optic atrophy and deafness. The annual incidence of this disease is at most 1 in 160,000 persons (63).
Bardet-Biedl syndrome
A wide spectrum of clinical features is associated with the autosomal recessive ciliopathy named Bardet-Biedl syndrome (52). Prevalence of the disease is especially increased among inbred and consanguineous populations. Primary findings include obesity, developmental disability, kidney dysfunction, rod-cone dystrophy, dystrophic extremities, and hypogonadism. Generally, children develop obesity by age three and ocular disturbances by age nine, at which point suspicion for Bardet-Biedl arises (67).
Pathophysiology of ophthalmic manifestations
Twenty different genes (BBS1-BBS20) that all code for ciliary proteins have been identified to play a role in the pathogenesis of Bardet-Biedl syndrome. Mutations in these genes impair ciliary genesis and function, and thereby result in disease of multiple systems that depend on intact ciliary function, including the reproductive and visual systems. Rods and cones are photoreceptor cells in the retina responsible for day and night vision, respectively. The outer segments of these cells are modified sensory cilia, which aid in the processes of photon capture and visual transduction (67).
Clinical presentation and relevance
Over 90% of patients with Bardet-Biedl syndrome develop retinitis pigmentosa due to dysfunction of both rods and cones. The first associated ocular finding is night blindness or nyctalopia, which typically manifests in early childhood. In fact, a decrease in rod and cone amplitudes may be detected as early as 2 years of age by electroretinogram testing (67). By the second decade of life, patients suffer a loss in peripheral vision, which eventually affects the central visual field (68). With disease progression in the third decade, 98% of patients report complete loss of vision (67). Additional ophthalmologic features that often accompany a diagnosis of Bardet-Biedl include myopia, strabismus, and cataract formation (52).
Treatment
At present, no efficacious therapy exists to combat the diffuse ciliopathy caused by Bardet-Biedl. As a result, infertility, progressive retinal dystrophy, and vision decline are inevitable outcomes of the disease (67). However, supplementation with high doses of vitamin A may aid in preservation of retinal function (61). The future for treatment of this disease currently rests on the potential of gene therapy (67,68).
Pediatric perspective
Symptoms of Bardet-Biedl syndrome develop slowly prior to age ten. For this reason, diagnosis typically occurs in adolescence or young adults. However, in patients with the correct constellation of symptoms, this disease should be considered given the considerable impact on health and quality of life (69).
Neurofibromatosis type 1 (NF-1)
NF-1 is a complex, autosomal dominant, multi-system disorder that affects 1 in 3,000 individuals. A diagnosis of NF-1 requires at least two of the following cardinal features: Lisch nodules, optic gliomas, neurofibromas, café au lait spots, freckling in axillary or inguinal regions, osseous lesions, and history of NF-1 in a first-degree relative (70). Patients with NF-1 may initially present to an endocrinologist with concerns for short stature or precocious puberty. Later, if affected patients undergo radiation therapy for optic gliomas, additional endocrinological care is often required due to subsequent deficiencies in TSH, ACTH, and gonadotropins (52).
Pathophysiology of ophthalmic manifestations
Neurofibromatosis is caused by a mutation on chromosome 17 of the gene that encodes neurofibromin. As a negative Ras regulator, neurofibromin is a tumor suppressor gene. Therefore, defective or absent neurofibromin causes irregular signaling and oncogenesis in nerve tissue in the skin, brain, and throughout the body (71).
Clinical presentation and relevance
Over half of NF-1 cases have ophthalmic involvement, with findings in the anterior segment and ocular adnexa (17). Lisch nodules, the most common ophthalmologic manifestation, jump in incidence from 22% in patients under 4 years of age to 96% in patients older than 20 years (72). They are benign melanocytic hamartomas appreciated on the surface of the iris as small, well-defined yellow-brown masses (Figure 10) (74). Patients with NF-1 may also present with glaucoma as a result of hamartomatous infiltration of the angle, angle closure secondary to nodular thickening of the ciliary body and/or choroid, or failure of the anterior chamber to develop normally (75). Furthermore, cutaneous or plexiform neurofibromas as well as café au lait spots may be noted on the eyelids and throughout the orbit, with the incidence of glaucoma being well-correlated with the presence of eyelid neuromas (17). Gliomas of the optic nerve and optic chiasm are the intraorbital tumors most commonly linked to this syndrome and are a significant cause of visual morbidity in neurofibromatosis. The highest prevalence of these tumors occurs in the first decade of life, but they can grow at any point in time. Children typically present with unilateral proptosis, pain, or eye redness, but may later develop decreased visual acuity and limited extraocular mobility. Depending on the size, location, and rate of growth, optic gliomas can cause blindness, visual field defects, or even result in loss of the eye (52).
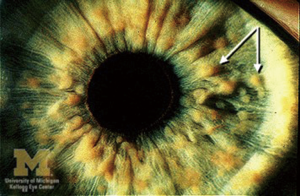
Treatment
Management of NF-1 is multifaceted. Surgical excision is often the treatment of choice for neurofibromas. Optic gliomas, because of their location, are more difficult to excise without significant morbidity. However, even radiotherapy which may be utilized in these cases, is not without its own risks, including optic atrophy, blindness, and complications of radiation to the orbit such as cataract, retinopathy, and secondary tumors (17). NF-1 related glaucoma is managed with topical ocular anti-hypertensives, or ophthalmic surgery when indicated. Lisch nodules, the most common ocular finding, are benign and require no intervention.
Pediatric perspective
Patients with NF-1 often live into adulthood, but 95% of patients receive their diagnosis by the age of 8 years (76). As with other autosomal dominant inherited disorders, it is imperative for clinicians to evaluate family history if there is suspicion for the presence of this syndrome. However, relying on the major and minor diagnostic criteria can be helpful in the absence of a known family history.
Acknowledgements
None.
Footnote
Conflicts of Interest: The authors have no conflicts of interest to declare.
References
- World Health Organization. Global report on diabetes. Isbn 2016;978:88.
- Boyle JP, Thompson TJ, Gregg EW, et al. Projection of the year 2050 burden of diabetes in the US adult population: Dynamic modeling of incidence, mortality, and prediabetes prevalence. Popul Health Metr 2010;8:29. [Crossref] [PubMed]
- Urrets-Zavalía JA, Espósito E, Garay I, et al. The eye and the skin in endocrine metabolic diseases. Clin Dermatol 2016;34:151-65. [Crossref] [PubMed]
- Kramer CK, Rodrigues TC, Canani LH, et al. Diabetic retinopathy predicts all-cause mortality and cardiovascular events in both type 1 and 2 diabetes: Meta-analysis of observational studies. Diabetes Care 2011;34:1238-44. [Crossref] [PubMed]
- Kohner EM, Patel V, Rassam SM. Role of blood flow and impaired autoregulation in the pathogenesis of diabetic retinopathy. Diabetes 1995;44:603-7. [Crossref] [PubMed]
- Lorenzi M. The polyol pathway as a mechanism for diabetic retinopathy: Attractive, elusive, and resilient. Exp Diabetes Res 2007;2007:61038. [PubMed]
- Singh VP, Bali A, Singh N, et al. Advanced glycation end products and diabetic complications. Korean J Physiol Pharmacol 2014;18:1-14. [Crossref] [PubMed]
- Dinn RB, Weingeist TA. Non-proliferative diabetic retinopathy. EyeRounds Online Atlas of Ophthalmology, 2005.
- Kirkpatrick C. Proliferative diabetic retinopathy (PDR). EyeRounds Online Atlas of Ophthalmology, 2015.
- Gauger E. Traction retinal detachment secondary to proliferative diabetic retinopathy. EyeRounds Online Atlas of Ophthalmology, 2013.
- Antonetti DA, Klein R, Gardner TW. Diabetic retinopathy. N Engl J Med 2012;366:1227-39. [Crossref] [PubMed]
- Ferris FL, Davis MD, Aiello LM. Treatment of diabetic retinopathy. N Engl J Med 1999;341:667-78. [Crossref] [PubMed]
- Karia N. Retinal vein occlusion: Pathophysiology and treatment options. Clin Ophthalmol 2010;4:809-16. [Crossref] [PubMed]
- Jeganathan VS, Wang JJ, Wong TY. Ocular associations of diabetes other than diabetic retinopathy. Diabetes Care 2008;31:1905-12. [Crossref] [PubMed]
- Pollreisz A, Schmidt-Erfurth U. Diabetic cataract-pathogenesis, epidemiology and treatment. J Ophthalmol 2010;2010:608751. [PubMed]
- Williams R, Airey M, Baxter H, et al. Epidemiology of diabetic retinopathy and macular oedema: A systematic review. Eye 2004;18:963-83. [Crossref] [PubMed]
- Thomann KH, Marks ES, Adamczyk DT. Primary eyecare in systemic disease. 2nd edition. Maidenhead, United States: McGraw-Hill Education Europe, 2001.
- Yanoff M, Duker J. Ophthalmology. 4th edition. Saunders, 2013.
- Trigler L, Siatkowski RM, Oster AS, et al. Retinopathy in patients with diabetic ophthalmoplegia. Ophthalmology 2003;110:1545-50. [Crossref] [PubMed]
- Somlai J, Kovacs T. Neuro-Ophthalmology. Springer International, 2016:187.
- Diabetes Control and Complications Trial Research Group, Nathan DM, Genuth S, et al. The effect of intensive treatment of diabetes on the development and progression of long-term complications in insulin-dependent diabetes mellitus. N Engl J Med 1993;329:977-86. [Crossref] [PubMed]
- Intensive blood-glucose control with sulphonylureas or insulin compared with conventional treatment and risk of complications in patients with type 2 diabetes (UKPDS 33). UK Prospective Diabetes Study (UKPDS) Group. Lancet 1998;352:837-53. [Crossref] [PubMed]
- The Diabetic Retinopathy Study Research Group. Photocoagulation treatment of proliferative diabetic retinopathy: The second report of diabetic retinopathy study findings. Ophthalmology 1978;85:82-106. [Crossref] [PubMed]
- Early Treatment Diabetic Retinopathy Study Research Group. Photocoagulation for diabetic macular edema. Early treatment diabetic retinopathy study report number 1. Arch Ophthalmol 1985;103:1796-806. [Crossref] [PubMed]
- Two-year course of visual acuity in severe proliferative diabetic retinopathy with conventional management. Diabetic Retinopathy Vitrectomy Study (DRVS) report #1. Ophthalmology 1985;92:492-502. [Crossref] [PubMed]
- Nguyen QD, Brown DM, Marcus DM, et al. Ranibizumab for diabetic macular edema: Results from 2 phase III randomized trials: RISE and RIDE. Ophthalmology 2012;119:789-801. [Crossref] [PubMed]
- Mitchell P, Bandello F, Schmidt-Erfurth U, et al. The RESTORE study: Ranibizumab monotherapy or combined with laser versus laser monotherapy for diabetic macular edema. Ophthalmology 2011;118:615-25. [Crossref] [PubMed]
- Haddad NM, Sun JK, Abujaber S, et al. Cataract surgery and its complications in diabetic patients. Semin Ophthalmol 2014;29:329-37. [Crossref] [PubMed]
- Nadeau KJ, Anderson BJ, Berg EG, et al. Youth-onset type 2 diabetes consensus report: Current status, challenges, and priorities. Diabetes Care 2016;39:1635-42. [Crossref] [PubMed]
- Elder DA, Herbers PM, Weis T, et al. β-cell dysfunction in adolescents and adults with newly diagnosed type 2 diabetes mellitus. J Pediatr 2012;160:904-10. [Crossref] [PubMed]
- Thomas RL, Harvey JN, Owens DR. When should screening for diabetic retinopathy begin for children with type 1 diabetes? Expert Rev Endocrinol Metab 2016;11:97-102. [Crossref]
- Smith TJ, Hegedüs L. Graves’ disease. N Engl J Med 2016;375:1552-65. [Crossref] [PubMed]
- Chen HC, Kohner EV. Endocrine disease and the eye. In: Duane’s Clinical Ophthalmology. Lippincott Williams & Wilkins, 2006:1-22.
- Bahn RS. Graves’ ophthalmopathy. N Engl J Med 2010;362:726-38. [Crossref] [PubMed]
- Weetman AP. Graves’ disease. N Engl J Med 2000;343:1236-48. [Crossref] [PubMed]
- Wiersinga WM, Bartalena L. Epidemiology and prevention of graves’ ophthalmopathy. Thyroid 2002;12:855-60. [Crossref] [PubMed]
- Bartley GB, Gorman CA. Diagnostic criteria for graves’ ophthalmopathy. Am J Ophthalmol 1995;119:792-5. [Crossref] [PubMed]
- Bartley GB, Fatourechi V, Kadrmas EF, et al. Clinical features of Graves’ ophthalmopathy in an incidence cohort. Am J Ophthalmol 1996;121:284-90. [Crossref] [PubMed]
- Trobe JD. Graves disease. The Eyes Have It, 2014.
- Gaillard F. Thyroid associated orbitopathy. Radiopaedia, 2010.
- Buzdugă C, Turliuc D, Găleşanu C, et al. Ocular manifestations in endocrine diseases. Rom J Funct Clin Macro-Microsc Anat Anthropol 2016;15:229-32.
- Lee HS, Hwang JS. The treatment of Graves’ disease in children and adolescents. Ann Pediatr Endocrinol Metab 2014;19:122-6. [Crossref] [PubMed]
- Goldstein SM, Katowitz WR, Moshang T, et al. Pediatric thyroid-associated orbitopathy: the Children’s Hospital of Philadelphia experience and literature review. Thyroid 2008;18:997-9. [Crossref] [PubMed]
- Lafferty AR, Chrousos GP. Pituitary tumors in children and adolescents. J Clin Endocrinol Metab 1999;84:4317-23. [Crossref] [PubMed]
- Lake MG, Krook LS, Cruz SV. Pituitary adenomas: An overview. Am Fam Physician 2013;88:319-27. [PubMed]
- Trobe JD. Visual pathway. The Eyes Have It, 2014.
- Erfurth EM. Endocrine aspects and sequel in patients with craniopharyngioma. J Pediatr Endocrinol Metab 2015;28:19-26. [Crossref] [PubMed]
- PDQ Pediatric Treatment Editorial Board. Childhood craniopharyngioma treatment (PDQ)-Health professional version. Natl Cancer Inst, 2017.
- Bunin GR, Surawicz TS, Witman PA, et al. The descriptive epidemiology of craniopharyngioma. J Neurosurg 1998;89:547-51. [Crossref] [PubMed]
- Al-Senawi R, Al-Jabri B, Al-Zuhaibi S, et al. Septo-optic dysplasia complex: Clinical and radiological manifestations in Omani children. Oman J Ophthalmol 2013;6:193-8. [Crossref] [PubMed]
- Webb EA, Dattani MT. Septo-optic dysplasia. Eur J Hum Genet 2010;18:393-7. [Crossref] [PubMed]
- Chopra R, Chander A, Jacob JJ. The eye as a window to rare endocrine disorders. Indian J Endocrinol Metab 2012;16:331-8. [Crossref] [PubMed]
- Ferran K, de , Paiva IA, Gilban DL, et al. Septo-optic dysplasia. Arq Neuropsiquiatr 2010;68:400-5. [Crossref] [PubMed]
- Kelberman D, Dattani MT. Septo-optic dysplasia - Novel insights into the aetiology. Horm Res 2008;69:257-65. [Crossref] [PubMed]
- Pilat A, Sibley D, McLean R, et al. High-resolution imaging of the optic nerve and retina in optic nerve hypoplasia. Ophthalmology 2015;122:1330-9. [Crossref] [PubMed]
- Tison K. Clinical findings and management of septo-optic dysplasia. Optom Vis Perf 2016;4:72-6.
- Giusti F, Marini F, Brandi ML. Multiple endocrine neoplasia type 1. GeneReviews®, 1993.
- Marini F, Falchetti A, Luzi E, et al. Multiple Endocrine Neoplasia Type 1 (MEN1) Syndrome. In: Riegert-Johnson DL, Boardman LA, Hefferon T, et al. editors. Cancer Syndromes. Bethesda (MD): National Center for Biotechnology Information (US), 2008.
- Marquard J, Eng C. Multiple endocrine neoplasia type 2. GeneReviews®, 1993.
- Kanski JJ. Systemic diseases and the eye: Signs and differential diagnosis. London, United Kingdom: Elsevier Health Sciences, 2001.
- Roy FH. Ocular syndromes and systemic diseases. 5th ed. New Delhi, India: Jaypee Brothers Medical Publishers, 2014.
- Machens A, Dralle H. Multiple endocrine neoplasia type 2: Achievements and current challenges. Clinics (Sao Paulo) 2012;67 Suppl 1:113-8. [Crossref] [PubMed]
- Urano F. Wolfram syndrome: Diagnosis, management, and treatment. Curr Diab Rep 2016;16:6. [Crossref] [PubMed]
- Kumar S. Wolfram syndrome: Important implications for pediatricians and pediatric endocrinologists. Pediatr Diabetes 2010;11:28-37. [Crossref] [PubMed]
- Schmidt-Kastner R, Kreczmanski P, Preising M, et al. Expression of the diabetes risk gene wolframin (WFS1) in the human retina. Exp Eye Res 2009;89:568-74. [Crossref] [PubMed]
- Rigoli L, Lombardo F, Di Bella C. Wolfram syndrome and WFS1 gene. Clin Genet 2011;79:103-17. [Crossref] [PubMed]
- Priya S, Nampoothiri S, Sen P, et al. Bardet-Biedl syndrome: Genetics, molecular pathophysiology, and disease management. Indian J Ophthalmol 2016;64:620-7. [Crossref] [PubMed]
- Castro-Sánchez S, Álvarez-Satta M, Valverde D. Bardet-Biedl syndrome: A rare genetic disease. J Pediatr Genet 2013;2:77-83. [Crossref] [PubMed]
- Forsythe E, Beales PL. Bardet-Biedl syndrome. Eur J Hum Genet 2013;21:8-13. [Crossref] [PubMed]
- Friedman J. Neurofibromatosis 1. GeneReviews®, 1993.
- Lakkis MM, Tennekoon GI. Neurofibromatosis type 1. J Neurosci Res 2000;62:755-63. [Crossref] [PubMed]
- North K. Neurofibromatosis type 1. Am J Med Genet 2000;97:119-27. [Crossref] [PubMed]
- Trobe JD. Neurofibromatosis type 1. The Eyes Have It, 2014.
- Lubs M-LE, Bauer MS, Formas ME, et al. Lisch nodules in neurofibromatosis type 1. N Engl J Med 1991;324:1264-6. [Crossref] [PubMed]
- Harley RD, Nelson LB, Olitsky SE. Harley’s Pediatric Ophthalmology. 5th edition. Lippincott Williams & Wilkins, 2005:292.
- Irons M, Ullrich N. Neurofibromatosis in Children. Boston Children’s Hospital, 2011.