Practice variations and rates of late onset sepsis and necrotizing enterocolitis in very preterm born infants, a review
Introduction
Although the rate of late onset sepsis (LOS) in newborns has modestly decreased in some areas as a result of ongoing quality improvement (QI) measures, LOS remains a frequent and devastating problem for newborns in high- and low-income countries (1). Current cause specific mortality rate per 1,000 live births below 29 weeks gestation for LOS is approximately 22 (2). LOS concern infections acquired during hospital stay, i.e., healthcare associated infections/nosocomial infections, which are most often defined as clear clinical evidence of infection and at least one microbiologically relevant positive result derived from a blood culture [including coagulase negative staphylococci (CoNS) and fungal pathogens] taken after 72 hours or 3 days of life (3,4).
Reports about changes of the burden of necrotizing enterocolitis (NEC) are more ambiguous in comparison. Whereas Horbar et al. report that 76% of neonatal intensive care units (NICUs) in the United States (US) reached the same low NEC incidence rate in 2014 as the best quantile of NICUs had reached in 2005 (3), a study from the National Institute of Child Health and Human Development (NICHD) of extremely preterm infants born between 2000–2011 reported that NEC related deaths rose from 23% to 30%, whereas overall mortality declined (2).
The risk of acquiring either LOS or NEC is inversely proportional to gestational age (GA) (5,6). Preterm infants are at higher risk for LOS for several reasons. The immune system of preterm infants exhibits distinct functions geared towards the fetus being well-equipped for life in utero and for appropriate mediation of the transition to ex-utero life at term. But when born preterm, the child’s immune system is not yet adapted to ex-utero life (7). Preterm infants often do not have a vernix caseosa because its production begins during the third trimester, their skin is thinner and takes longer to develop a barrier function than in term born infants, and the majority of maternal immunoglobulin G (IgG) antibody transfer occurs during the last trimester so that preterm born infants are deplete of IgG. This all impairs a robust immune response to pathogens (8,9). Another reason preterm infants are at higher risk for infection is because natural barriers towards infection often need to be compromised in order to be able to provide intensive care: the skin is affected by tapes, catheters, surgery, etc.; and the mucosa by ventilation, nutrition, medication, etc. This may disable the infant’s defense against pathogens (10). Lastly, length of stay in a hospital environment is also inversely proportional to GA and thus submit preterm infants to hospital pathogens for long periods of time (11).
Although an impaired immune system is also believed to play a role in NEC (12), its pathogenesis is less well understood. It appears to be multifactorial, reflecting several different pathways to intestinal necrosis with different inciting factors. Complicating the issue is that NEC may well be more than one disease with separate pathogenesis (12). NEC mostly occurs in infants born prematurely as its etiological factors are largely related to immaturity of the gastrointestinal tract. After birth, the neonatal gut must acquire a healthy complement of commensal bacteria. Disruption or delay of this critical process, leading to deficient or abnormal microbial colonization of the gut, has been implicated as key risk factor in the pathogenesis of NEC (6,13,14). What follows is profound inflammation and intestinal injury (15,16).
LOS and NEC have long-term implications for newborns: a 2013 systematic review and meta-analysis on neurodevelopmental outcome of very low birthweight infants (<1,500 g, VLBW) with neonatal sepsis found 17 studies matching their criteria which indicate that the diagnosis of neonatal sepsis in VLBW infants is associated with an increased risk of one or more long-term neurodevelopmental impairments including higher incidence of cerebral palsy (5). NEC and surgery for NEC have also been associated with increased risk of adverse neurodevelopmental outcome at two years of age (17-19). The financial impact of NEC is estimated at $1 billion per year in the US alone (15). In a study of extremely preterm born infants surviving to 36 weeks postmenstrual age, the presence of LOS or NEC independently increased the risk of late death or survival with neurosensory impairment (20).
Among pediatric acute care hospitals, the prevalence of infections was highest in pediatric intensive care units (15.5%, 95% CI: 11.6–20.3%) and NICUs (10.7%, 9.0–12.7%). This review will focus on literature about LOS or NEC in very preterm infants (<32 weeks gestation) or VLBW infants in order to cover the highest risks of acquiring either illness (21). We will compare LOS and NEC incidence proportions between large geographically defined cohorts and reveal how QI measures rather than novel interventions currently drive the change towards better outcome.
Epidemiology
Because differences exist in the definition of LOS and NEC between health care providers, it is difficult to establish an epidemiological overview for either: Most use 3 days or 72 hours after birth as onset to differentiate nosocomial infections from infections acquired via the mother (early onset sepsis, EOS). However, some use 48 hours or 2 days and again others use 7 days as threshold (Table 1). Usually the definition includes positive blood culture and clinical signs of infection, but sometimes blood culture alone is deemed sufficient (3). Some require additional clinical signs or two independent blood samples to verify coagulase negative staphylococci (CoNS) (26,37). This makes sense as it better allows differentiating true CoNS infestation from a contamination of the blood sample because CoNS is a common colonizer of the human skin (38). However, most include CoNS as they do any other culture proven sepsis. Lastly, some publications specifically state the requirement of at least 5 days of antibiotic treatment in addition to the positive culture result to define an LOS episode (3,22-24,34,36).
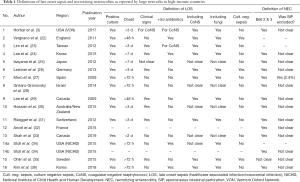
Full table
If we tolerate some constraint in scientific rigor, as is recommended for the purpose of uncovering potential for QI (39), then we may interpret some of the reported LOS incidence proportions as comparable, even when their definitions do not match. Publications using 2 or 3 days as onset are roughly comparable as the peak incidence of LOS is between the 10th and 22nd day of life (4) and EOS prevalence is considerably lower than that of LOS (40), particularly if only cases that present on the 3rd day of life are driving the difference. Additional requirements including the presence of clinical signs or at least 5 days antibiotic treatment should not disable comparison as in most cases blood cultures are cultivated upon clinical signs and positive cultures usually receive 5 or more days of antibiotic treatment (21). Comparisons should nevertheless be treated with caution, particularly if minimum day of onset is after day 7 of life, CoNS are excluded, or if CoNS require 2 blood samples. The latter two are because CoNS account for 53.2–77.9% of LOS in industrialized countries and 35.5–47.4% in some developing regions (4). This means that a different onset or handling of CoNS will have an impact on reported proportions. Another issue to observe is the population used as denominator and whether it encompasses a geographical region. For instance, Grisaru et al. report LOS proportions for two time periods in Israel: 33.4% for 2001–2005 and 28.6% for 2006–2010, respectively (28). This is higher than the 26% reported by Vergnano et al. from England for 2007–2008 (22). Even though both reported proportions for VLBW infants, the comparability is limited because Israel’s data is population based whereas the data for England represents 12 units dedicated to data collection. Also, Israel restricted its population to singleton births which may have a different risk for adverse outcome than multiple births (41).
Comparability between studies is equally an issue in reported NEC proportions, largely because of the multifactorial nature of the disease. Whereas most report their NEC incidence based on the modified version of Bell’s stage 2 or higher (including specific radiologic signs, i.e., pneumatosis intestinalis) (42), some diagnose NEC at a different stage or avoid using Bell’s criteria altogether (6,43). Spontaneous intestinal perforation (SIP) is an illness with sometimes similar symptoms but different pathogenesis from NEC. However, most publications do not specify if cases of SIP were excluded from their NEC incidence prior to reporting (Table 1). Therefore, comparisons between health care providers need to be treated with caution.
Table 2 lists LOS and NEC incidence proportions of several large, geographically defined, prospective neonatal data collections for the last two decades. This list does not claim to be exclusive but it does contain results from the best known large neonatal data collections from Australia/New Zealand, Canada, France, Germany, Israel, Japan, Korea, Spain, Sweden, Switzerland and the US (3,24,25,27-35,45). LOS proportions range from 5% in Japan to 29.4% in Spain for VLBW infants whereas NEC proportion range from 1.6% again in Japan to 7.1% in the US. Whenever available, we listed 2 consecutive periods that inform on a development over time. Most impressive is the one reported by the Vermont Oxford Network (VON) (Horbar et al.) on the development of LOS among VLBW from an internationally comparable 21.9% in 2005 to a low 10.1% 9 years later. In the same study, the proportion of NEC was reduced from 7.1% to 5.2%. Horbar et al. maintain “these results illustrate the magnitude and pace of improvement that has occurred over the decade and describe the variation in outcomes that persists among different NICUs.” (3). Although we are far from being able to infer causality on the part of QI on outcome, we can assume that collaboration in a neonatal network such as the VON with its improvement programs, teaching and reporting do have an effect (46,47). This is illustrated with enhanced statistical rigor also by the Canadian Neonatal Network. Their Evidence Based Practice for Improving Quality (EPIQ) program demonstrated improvement in LOS in a cluster randomized setting in 2005 (29) and in an observational setting using EPIQ in LOS and NEC in 2017 (33) (Table 2).
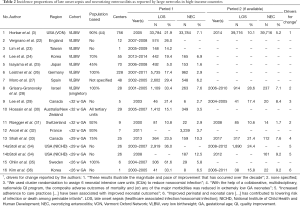
Full table
Also reporting improvement over time based on improved peri- and neonatal care practices are Stoll et al. for the NICHD and Kim et al. for the Korean Neonatal Network (Table 2). Of the commonly reported neonatal outcomes, LOS is unique in so far that the substantial decreases observed in recent years is compared with little change in the previous decades. This decrease has occurred without any specific new therapy or intervention having been introduced (44).
As mentioned, CoNS are the most prominent pathogens of LOS. They are however not as virulent as Gram-negative bacteria and possibly fungi which partly explains the lower rate of short-term infectious complications as well as mortality associated with CoNS sepsis (48,49). Makhoul et al. for instance reported 6.3-fold and 12.3-fold increased risk of early mortality associated with Klebsiella and Pseudomonas sepsis when compared with CoNS (50). However, the risk of neurodevelopment sequelae, such as cognitive and psychomotor impairment, cerebral palsy, and vision impairment was independent of the type of pathogen, indicating that CoNS are capable to exert a long-term detrimental effect on the host (4). In a recent systematic review, Dong et al. concluded that VLBW infants may differ from more mature neonates in terms of disease burden and clinical course of LOS caused by Gram-negative bacteria. Using strategies targeting Gram-negative bacteria, new epidemiologic studies aided by advanced molecular techniques may help to develop an anti-sepsis protocols specialized for VLBW infants (51).
Apart from CoNS as the most common finding from blood cultures in all publications, the other pathogens seem to vary regionally. In their study on neonatal infections in England, the NeonIN group convincingly reveal how the identified strains match other reports from the UK or Germany but are different from infection surveillance reports from the US or Australia (22). Similar geographically dependent differences are reported in Asia or Spain (52,53).
Diagnosis
Another factor exacerbating an epidemiological overview over LOS and NEC is that diagnosing them in clinical practice requires skill and experience. To date, no clear biomarker with high positive and negative predictive value (PPV/NPV) exists so that diagnosis is subjective and variable (54).
Symptoms of LOS are often subtle and non-specific since virtually every clinical finding has been associated with sepsis (21). The low (mostly 1 mL or even lower) volume of bacterial blood samples typically taken for culture have low sensitivity (7). PPV is poor because there is no consensus on how to determine whether the most common isolates, i.e., CoNS, represent true pathogens or contaminants picked up during sampling (55). NPV is difficult to determine because there is no consensus on how to diagnose clinically relevant sepsis where a positive blood culture is missing. Of the 16 reports listed in Tables 1,2, only 3 account separately of culture negative sepsis.
To support diagnosis, ancillary lab tests are commonly used, such as C-reactive protein, interleukins, procalcitonin and others. If they are applied correctly, i.e., using postnatal and GA specific reference values, these tests have good NPV but poor PPV (56,57). This is important because at least in the absence of a positive blood culture, negative lab values allow discontinuation of antibiotic treatment.
A promising development is the ability to identify pathogens in the blood by analyzing their DNA via polymerase chain reaction (PCR). Compared with the conventional culture technology, PCR technologies yield results faster, in a higher sensitivity and with a much smaller sample volume. Recently developed PCR-based diagnostic platforms are highlighted by a low contamination rate (4). This could allow better differentiating between true and contaminated CoNS infestation. However, Molecular assays do not yet have sufficient sensitivity to replace microbial cultures in the diagnosis of LOS (58). Until these tests become available, clinicians will need to continue to make preliminary decisions based on nonspecific signs and tests with poor PPV, particularly in the extremely preterm infants where 1 mL blood samples are difficult to obtain.
In recent years it has however become clear that the absence of certainty cannot be met by just withholding treatment or providing full treatment as either will affect the child’s development and in the case of overtreatment lead to microbial resistance (see below). Instead, constant reassessment is required. For this reason, prediction models with clinical parameters have been developed to facilitate preliminary sepsis diagnosis and the initiation of antimicrobial therapy. A recent systematic review however concluded that these models should be considered as guidance rather than an absolute indicator because of their limited diagnostic accuracy (59).
The initial stages of NEC are also comprised of non-specific signs and symptoms which overlap with other conditions such as sepsis, apnea, or feeding intolerance. The diagnosis of NEC is based on a combination of clinical, radiological, and lab findings. A reliable biomarker is missing. Although disputed by many as inaccurate, incomplete or outdated (6,43), the most common clinical staging system still is the modified Bell’s staging (42) (Table 1).
Treatment
Guidelines for the management of EOS exist and have shown effect on the one hand by reducing the risk of EOS but also changing pathogens causing EOS (60,61). Similar evidence to support guidance for the management of LOS in NICUs is still missing (62). This may be due to the more similar spectrum of reported organisms causing EOS than in LOS, where we find larger regional variability (4,22,40,52,53). Treatment guidelines for EOS focus largely on the two most prevalent strains group B streptococcus and E. coli. In LOS there are different pathogens and different sites of infection (blood, brain, lung, urinary tract, or skin and soft tissue) which require different types of antibiotic compositions and durations of treatment (21). For the last several decades, therefore, treatment for LOS has remained largely empirical and focused mainly on antibiotics. Antibiotics are hence the most commonly prescribed medications in newborn infants requiring intensive care (63). Clinical trials to improve treatment are rare because of a lack of consensus in definition of sepsis and selection of appropriate endpoints (64). As a result, there is inadequate evidence from randomized trials in favor of any particular antimicrobial regimen (65).
As even fulminant infections may have minimal and unspecific early warning signals, antimicrobial treatment must proceed in all suspected cases without delay. Understanding the local microbiological epidemiology of the patient, the neonatal unit and the hospital as a whole is essential in order to choose the appropriate empiric therapy (21). As the exact pathogen is not known at onset of treatment, initial treatment usually targets many different pathogens simultaneously. The resulting intensive use of antimicrobials in neonatal units has led to changes in the antimicrobial susceptibility patterns of organism (62,66). Once the pathogen is identified in culture, usually within 48 to 72 hours, treatment should therefore be converted by switching to the narrowest effective agent or by terminating the unspecific course of antibiotics. But the knowledge on how to adjust or terminate antibiotic use is limited. The most frequent blood culture result, CoNS, may mean a contamination. If the culture is negative, then this may not automatically mean that the child is free of a debilitating pathogen but instead it may be due to previous antibiotic exposure, low colony count sepsis, and difficulty obtaining an adequate volume of blood for culturing (67). Particularly in extremely preterm born infants, previous antibiotic exposure is frequent if not ubiquitous due to EOS prophylaxis (62). Also, these infants are the ones where adequate volume of blood is most difficult to obtain.
Variations in the treatment of LOS, particularly in the use of antibiotics, is therefore to be expected. In a study enrolling infants receiving >72 hours antibiotic treatment, 35% received them inappropriately. The overuse was largely due to failure to narrow antibiotic coverage after microbiologic results were known (66). This result was confirmed by a recent study in 2019 on VLBW infants in which the most important factor determining duration of initial antibiotic treatment was clinical status and not laboratory results (68). Along these lines, the California Perinatal Quality Care Collaborative recently reported a 40-fold variation in antibiotic use rates between units, ranging from 2.4% to 97.1% of patient-days. The study highlights significant variations in practice and the almost certain overuse of antibiotic therapy as the 40-fold variation could not be attributable to clinical differences in the NICUs, including the rate of positive culture results (69). In a survey of guidelines for the use of 41 antimicrobials in French NICUs, 444 dosage regimens were identified. The number of different dosing regimens varied from 1 to 32 per drug (70). Another European study including 89 NICUs found wide variation in dosing of antibiotics, with significant under- and overdosing (71).
Obviously, treating infants at even a low risk of a possibly lethal infection with antibiotics seems indicated and not treating without certainty of no risk is difficult to legitimize. But the potentially unnecessary exposure to antibiotics may affect the immediate course of the child’s hospitalization and its long-term outcome. Studies have linked the number of days exposed to antibiotics with subsequent mortality, a higher risk for NEC and non-CoNS infections (62). Alterations in the gut microbiome, an effect of exposure to antimicrobials, may be linked to long-term outcomes such as childhood obesity or asthma (62,67).
Finding ways to limit antibiotic use therefore are necessary and have a large potential both for the improvement of care and the limitations of cost. Cost can be reduced by direct reduction of use and, more importantly, by the possible reduction of short- and long-term adverse outcome. The Choosing Wisely campaign in the US charges medical societies with identifying unnecessary tests and treatments that contribute to healthcare waste (72). A broad selection of stakeholders selected the five foremost tests or treatments that cannot be adequately justified on the basis of efficacy, safety or cost in newborn medicine. As number two, the stakeholders selected ‘avoid routine continuation of antibiotic therapy beyond 48 hours for initially asymptomatic infants without evidence of bacterial infection’ (73).
Whereas randomized trials for the treatment of LOS are missing, the evidence base for antibiotic stewardship programs (ASP) aimed at limiting unnecessary antibiotic treatment is currently growing. A recent Cochrane review summarized 58 randomized controlled trials (RCTs) and 163 non-randomized studies from adult medicine. They concluded that there was high-certainty evidence that interventions were effective in increasing compliance with antibiotic policy and reducing duration of antibiotic treatment. Based on lower quality of evidence, they also maintained that lower use of antibiotics probably did not increase mortality and likely reduced length of stay (74).
In 2016, the VON partnered with the American Center for Disease Control (CDC) to establish an ASP focused on decreasing antibiotic overuse in newborns. It was based on the CDC recommended 7 core elements: leadership, commitment, accountability, drug expertise, action, tracking, reporting, and education. In a first assessment, Ho et al. analyzed the baseline prevalence and makeup of this ASP and assessed the variability of NICU antibiotic use rates (75). Among the participating 143 centers completing structured self-assessments, no center addressed all 7 core elements. There were significant gaps between CDC recommendations to improve antibiotic use and antibiotic practices during the newborn period and wide variation in point-prevalence antibiotic use rates. Seventy-four percent of infants who received antibiotics >48 hours did not have infections proven by culture documenting ample potential for improvement based on the Choosing Wisely initiative alone (75).
Medical treatment for NEC includes bowel decompression and rest, fluid resuscitation, antibiotic therapy, and supportive care. Antibiotic treatment is indicated as sepsis may result in 20–30% infants due to translocation of organisms through a compromised intestinal barrier (21). Indication for surgical intervention is given in cases of pneumoperitoneum or clinical deterioration despite maximal medical therapy (76-78).
Prevention
Improving treatment for LOS and NEC is adamant. But an even better approach is to avoid either of them altogether. As antibiotic overuse carries the risk for adverse short and long-term outcome, reducing their use in an ASP can be seen as treatment guide and as prevention. Other risk factors for LOS and NEC have been amply described and may serve as target for prevention.
LOS has been associated with lack of hygiene as far back as 1847 when Ignaz Semmelweis realized that hands play an important role in the chain of transmission of bacteria. At the obstetrical clinic in Vienna, the German-Hungarian physician ordered the use of chlorinated lime solution for hand washing and thereby reduced mortality rate due to childbed fever from 18% to 2%. According to Dr. Stoll et al.’s landmark paper on LOS in 2002, risk factors other than above mentioned GA/birth weight or antibiotic overuse, are catheters, hyperalimentation, age at first feeding, age at full feeding and mechanical ventilation (37). These risk factors were later confirmed in other studies (79-82), and in 2015 Dr. Stoll herself concluded that “the reduction in late-onset sepsis likely reflects increased attention to improved hand hygiene, skin care, human milk feeding, uniform practices for catheter insertion and care (central line bundles), and attention to discontinuing invasive devices when not needed.” (34). Risk factors for NEC further include male sex, fetal growth restriction and a hemodynamically significant patent ductus arteriosus, the latter if treated with indomethacin (83-86). However, the key risk factor remains a deficient or abnormal microbial colonization of the gut (see above) (6,13,14). This deficiency can be met by reducing unnecessary exposure to antibiotics, better hygiene, and specific prevention measures discussed below.
Prevention strategies largely focus on minimizing the listed risk factors. Even though it is meanwhile common knowledge that appropriate hand hygiene (HH) reduces the risk of LOS, contemporary reports still document improvement potential in the compliance rates of hand disinfection routines. The most often cited figure for HH compliance is 40% and originates back to a review of 34 studies in 2002 (87). Whereas a current systematic review summarizing HH compliance is missing (88), several individual reports exist on humble HH compliance. In their 2018 study, Cunningham et al. describe their response to a spike in nosocomial infection in a children’s hospital. After first consulting their records on self-reported compliance rates for HH in their hospital, which were high, they were astounded that unannounced checks revealed that HH compliance was at a low 50%. As previous reports on improvement and sustainability of HH compliance did not convince them, they applied an approach developed in military known as stand-down. As a result, compliance increased from less than 65% to more than 95% and was sustained for 3.5 years at the time of reporting (89). In a neonatal setting, Pessoa-Silva et al. report a sustainable increase in HH compliance from 42% to 55% (90), whereas Raskind et al. report falling back to their baseline 89% HH compliance after 3 months (91). Judging from the heterogeneity of the reports on compliance and sustainability, unannounced observation of local HH seems a worth-while approach to test for local improvement potential.
Another approach to LOS prevention currently addresses central line-associated bloodstream infections (CLABSI), one of the major contributors to LOS. CLABSI reduction can be achieved by combining evidence-based prevention strategies into “bundles”. Bundles mainly focus on avoiding central-line insertion whenever possible, minimizing dwell times, and careful attention to sterile line maintenance (4,8). A recent systematic review and meta-analysis additionally lists HH, empowerment of staff, feedback to staff, specialized teams, as well as reviewing compliance and check lists as reported elements of CLABSI reduction bundles. The cumulative analysis of 27 studies from 2002 to 2016 showed a statistically and clinically significant benefit of preventive bundles to avoid CLABSI in neonatal intensive care. However, the studies also showed heterogeneity in endpoint definitions and implemented measures as well as a publication bias towards reporting success rather than failure (92).
An evidence-based overview over which bundle elements are most effective is missing and cannot be drawn out of the afore mentioned systematic review or individual studies (92,93). However, comprehensive guidelines exist and recommend specific measures along the lines listed above (94,95). Based on these guidelines, a NICU in Vienna, Austria, developed an interesting approach focusing on simulation training of central line insertion (96). Their multidisciplinary team applied a bundle consisting of three elements: (I) a simulation-based standardization and education of a peripherally inserted central catheter insertion technique, (II) improvement of breast milk hygiene management by standardized HH trainings for mothers and (III) compulsory standardized HH trainings for all staff members. As a result, their CLABSI infections per 1,000 central line days decreased from 13.9 in 2010 to 9.5 in 2011 and 4.7 in 2012 (96).
The further preventive measures target both LOS and NEC and focus on human milk feeding and onset of feeding, or on providing the benefiting components of human milk when it is not directly available: donor milk, probiotics, prebiotics and lactoferrin. Human milk contains antimicrobial proteins and peptides as well as other bioactive molecules. They protect the infant against infection, contribute to a healthy microbial colonization of the gastrointestinal tract and support appropriate maturation of the developing immune system (7,8). Microbes present in human milk may act as commensal gastrointestinal microorganisms and potentially have beneficial effects for the developing gastrointestinal tract. The taxa from individual mother’s samples over time remains similar, but each mother’s samples differ from other mothers’ milk microbiota, suggesting a specific microbial ecology for each mother-infant dyad. Individual studies have shown that providing the infant with own mother’s milk (OMM) decreased rates of LOS, NEC, retinopathy of prematurity, lower rates of re-hospitalizations in the first year of life, and improved neurodevelopmental outcome (97,98). Evidence from a systematic review supporting these findings in premature infants is missing (99). However, in their own systematic review on neurodevelopmental outcomes of preterm infants fed human milk, Lechner and Vohr conclude that any intervention that has the potential to decrease morbidities with long-term effects (such as LOS and NEC) and the potential to increase cognitive ability (by increasing white matter development and cortical thickness) must be supported even if the reported effect size is small (100).
To avoid deficient or abnormal microbial colonization of the gut, implementing evidence-based standardized feeding guidelines may help reduce the incidence of NEC (16). Although such guidelines are not uniform, they usually incorporate an early minimal enteral nutrition phase during which 10–20 mL/kg/d of enteral nutrition is provided without increase, followed by daily advancement based on continued tolerance. Older studies suspected an association of NEC with the start of enteral feeding and therefore withheld feedings for days to weeks after birth (101).
If human milk is not available, donor milk may be an alternative. But even if using donor milk in lieu of formula feedings has led to reduced NEC rates in recent studies, it is unclear whether donor milk itself protected against NEC or whether the avoidance of formula acted as a protective factor (16). Other issues with donor milk concern pasteurization, which significantly decreases the concentration of many of the protective elements in human milk. Also, the effect of the milk may be hampered because the GA of the donors’ infants and the infants receiving their milk are mismatched or the donor milk may be pooled (7).
Multiple meta-analyses of randomized studies have shown that probiotics are associated with reduced rates of NEC and LOS (102,103). Yet dissent prevails. Some maintain, sufficient evidence is given for probiotics-prophylaxis (104). Others maintain that evidence is missing on which subgroups really require or benefit from probiotics (e.g., when used as a supplement to OMM) or regarding the unknown long-term effects on immune function and metabolism following replacement of a maternally derived intestinal microbiome with a dominant exogenous bacterial species (105). These reasons and a lack of consensus regarding the strain(s), dosage, duration and timing of use has prevented many units from adopting it as a prevention strategy.
Further preventive approaches concern the commonly used technique in NICUs called kangaroo mother care or skin-to-skin care, where there is close contact between mother and infant. This could potentially have a salutary effect on the mucosal immune system of the preterm infant (12).
Several RCTs have been undertaken to find alternative approaches to treating or preventing LOS and NEC. Polyclonal intravenous immunoglobulin (IVIG) targets the lack of IgG in preterm infants. In its meta-analysis, a 2013 Cochrane review found that overall there was a 3% reduction in sepsis and a 4% reduction in one or more episodes of any serious infection but found not association with reductions in other clinically important outcomes, including mortality. The authors concluded that it depended on costs and the values assigned to the clinical outcomes whether IVIG should be implemented and that no further studies were warranted (9,106).
Immune replacement therapies were widely explored in the hope of correcting the immune deficiencies and thus preventing neonatal infections. Colony-stimulating factors (CSF), such as granulocyte CSF and granulocyte macrophage CSF, are cytokines that promote the proliferation and antimicrobial function of neutrophils, monocytes and macrophages. Several clinical trials investigated the effect of supplying granulocyte CSF (G-CSF) and granulocyte/macrophage (GM-SCF) in the prevention and treatment of neonatal sepsis. However, a 2003 Cochrane review of ten studies demonstrated no significant survival advantage at 14 days from the start of therapy (7). This may be because the immune system of preterm infants is not simply immature, but rather specifically regulated for the early stage of postnatal life (4).
Pentoxifylline is a non-specific phosphodiesterase inhibitor with immunomodulatory properties. It may be beneficial in preterm neonates with LOS or NEC as its effects are more pronounced in neonatal immune cells than in adults. A meta-analysis of six RCTs encompassing 416 infants concluded that Pentoxifylline, when used as an adjunct therapy to antibiotics in neonatal sepsis, might decrease mortality (relative risk 0.38, 95% CI: 0.20–0.71). But the overall quality of evidence was determined as low by the authors. They concluded that further large trials were needed (107). This may soon be provided by an RCT in Australia/New Zealand, which is currently recruiting 1,800 infants to determine if pentoxifylline can improve long-term outcome in preterm infants with LOS or NEC (108).
The role of epidermal growth factors, prebiotics, glutamine, and oral lactoferrin on reducing the risk of NEC has not yet been confirmed. Prebiotics are human milk oligosaccharides that promote the growth of beneficial commensal probiotics like Bifidobacterium and Bacteroides in the healthy breast-fed term infants’ intestine. Lactoferrin limits the amount of iron available to pathogenic bacteria and promotes growth of commensal bacteria (9). Investigations into these products, particularly prebiotics, are presently underway (7).
Conclusions
LOS and NEC are multifaceted illnesses that are difficult to diagnose, rendering a comparison between health care providers difficult. Comparison is further complicated because an overall accepted and applicable definition for either is missing to date. After decades of research, both continue to have a high burden on the health of newborns, particularly very preterm born infants.
Empirical therapy for LOS is often inappropriately used with unnecessary broad-spectrum antibiotics and a prolonged duration of treatment. This leads to an increasing number of multidrug-resistant Gram-negative micro-organisms which poses a serious concern worldwide (51). Beyond antibiotics and supportive care, there is presently no approved drug for the treatment or prevention of sepsis in preterm or term neonates (7).
New findings suggest that many of the long-standing practices used to treat or prevent infection or NEC would profit from (I) improved local performance where effective care is not provided and (II) local revision of current practices in relation to newest findings in basic research (e.g., on the function and deficiencies of the preterm born immune system and the benefits of OMM). Local data collection or better yet, participating in a national surveillance system, form the prerequisite for both and have led to substantial reduction of LOS (109).
A better implementation of known effective care models may then form the basis for novel therapies. Novel therapies require proof of effectiveness, usually by performing RCTs. As numbers needed to treat are high, neonatal trials are commonly performed on a multicenter level. However, if the different centers do not observe the same hygiene rules, antibiotic stewardship, milk feeding practices, pro-/pre- or symbiotic usage, and/or central line removal policies, the effect of a new intervention for LOS or NEC may well be biased during trials even if randomization is performed correctly, because the new treatments most often target the same pathways as the prevention practices listed.
This review also reveals several concrete knowledge gaps of which the community would greatly profit if they were met: evidence to support guidance for the management of LOS in NICUs, a systematic review on the effect of OMM on LOS or NEC and evidence on the effectiveness of probiotics to prevent NEC in infants receiving OMM.
Acknowledgments
None.
Footnote
Conflicts of Interest: M Adams receives a salary as network coordinator for the Swiss Neonatal Network. D Bassler has no conflicts of interest to declare.
Ethical Statement: The authors are accountable for all aspects of the work in ensuring that questions related to the accuracy or integrity of any part of the work are appropriately investigated and resolved.
References
- Wynn JL. Defining Neonatal Sepsis. Curr Opin Pediatr 2016;28:135-40. [Crossref] [PubMed]
- Patel RM, Kandefer S, Walsh MC, et al. Causes and Timing of Death in Extremely Premature Infants from 2000 through 2011. N Engl J Med 2015;372:331-40. [Crossref] [PubMed]
- Horbar JD, Edwards EM, Greenberg LT, et al. Variation in Performance of Neonatal Intensive Care Units in the United States. JAMA Pediatr 2017;171:e164396. [Crossref] [PubMed]
- Dong Y, Speer CP. Late-onset neonatal sepsis: recent developments. Arch Dis Child Fetal Neonatal Ed 2015;100:F257-63. [Crossref] [PubMed]
- Alshaikh B, Yusuf K, Sauve R. Neurodevelopmental outcomes of very low birth weight infants with neonatal sepsis: systematic review and meta-analysis. J Perinatol 2013;33:558-64. [Crossref] [PubMed]
- Battersby C, Longford N, Costeloe K, et al. Development of a Gestational Age-Specific Case Definition for Neonatal Necrotizing Enterocolitis. JAMA Pediatr 2017;171:256-63. [Crossref] [PubMed]
- Schüller SS, Kramer BW, Villamor E, et al. Immunomodulation to Prevent or Treat Neonatal Sepsis: Past, Present, and Future. Front Pediatr 2018;6:199. [Crossref] [PubMed]
- Collins A, Weitkamp JH, Wynn JL. Why are preterm newborns at increased risk of infection? Arch Dis Child Fetal Neonatal Ed 2018;103:F391-4. [Crossref] [PubMed]
- Ramasethu J. Prevention and treatment of neonatal nosocomial infections. Matern Health Neonatol Perinatol 2017;3:5. [Crossref] [PubMed]
- Kollmann TR, Kampmann B, Mazmanian SK, et al. Protecting the Newborn and Young Infant from Infectious Diseases: Lessons from Immune Ontogeny. Immunity 2017;46:350-63. [Crossref] [PubMed]
- Seaton SE, Barker L, Draper ES, et al. Estimating neonatal length of stay for babies born very preterm. Arch Dis Child Fetal Neonatal Ed 2019;104:F182-6. [Crossref] [PubMed]
- Neu J. Necrotizing Enterocolitis: The Mystery Goes On. Neonatology 2014;106:289-95. [Crossref] [PubMed]
- Patel RM, Denning PW. Intestinal Microbiota and Its Relationship with Necrotizing Enterocolitis. Pediatr Res 2015;78:232-8. [Crossref] [PubMed]
- Guthrie SO, Gordon PV, Thomas V, et al. Necrotizing Enterocolitis Among Neonates in the United States. J Perinatol 2003;23:278-85. [Crossref] [PubMed]
- McElroy SJ. Unraveling the enigma that is neonatal necrotizing enterocolitis. J Perinatol 2014;34:729-30. [Crossref] [PubMed]
- Patel AL, Panagos PG, Silvestri JM. Reducing Incidence of Necrotizing Enterocolitis. Clin Perinatol 2017;44:683-700. [Crossref] [PubMed]
- Martin CR, Dammann O, Allred EN, et al. Neurodevelopment of extremely preterm infants who had necrotizing enterocolitis with or without late bacteremia. J Pediatr 2010;157:751-6.e1. [Crossref] [PubMed]
- Schlapbach LJ, Adams M, Proietti E, et al. Outcome at two years of age in a Swiss national cohort of extremely preterm infants born between 2000 and 2008. BMC Pediatr 2012;12:198. [Crossref] [PubMed]
- Hintz SR, Kendrick DE, Stoll BJ, et al. Neurodevelopmental and Growth Outcomes of Extremely Low Birth Weight Infants After Necrotizing Enterocolitis. Pediatrics 2005;115:696-703. [Crossref] [PubMed]
- Bassler D, Stoll BJ, Schmidt B, et al. Using a Count of Neonatal Morbidities to Predict Poor Outcome in Extremely Low Birth Weight Infants: Added Role of Neonatal Infection. Pediatrics 2009;123:313-8. [Crossref] [PubMed]
- Cantey JB. Neonatal Infections: Pathophysiology, Diagnosis, and Management. Cham: Springer International Publishing, 2018.
- Vergnano S, Menson E, Kennea N, et al. Neonatal infections in England: the NeonIN surveillance network. Arch Dis Child Fetal Neonatal Ed 2011;96:F9-14. [Crossref] [PubMed]
- Lim WH, Lien R, Huang YC, et al. Prevalence and Pathogen Distribution of Neonatal Sepsis Among Very-Low-Birth-Weight Infants. Pediatr Neonatol 2012;53:228-34. [Crossref] [PubMed]
- Lee SM, Chang M, Kim KS. Blood Culture Proven Early Onset Sepsis and Late Onset Sepsis in Very-Low-Birth-Weight Infants in Korea. J Korean Med Sci 2015;30:S67-74. [Crossref] [PubMed]
- Isayama T, Lee SK, Mori R, et al. Comparison of Mortality and Morbidity of Very Low Birth Weight Infants Between Canada and Japan. Pediatrics 2012;130:e957-65. [Crossref] [PubMed]
- Leistner R, Thürnagel S, Schwab F, et al. The impact of staffing on central venous catheter-associated bloodstream infections in preterm neonates – results of nation-wide cohort study in Germany. Antimicrob Resist Infect Control 2013;2:11. [Crossref] [PubMed]
- Moro M, Pérez-Rodriguez J, Figueras-Aloy J, et al. Predischarge Morbidities in Extremely and Very Low-Birth-Weight Infants in Spanish Neonatal Units. Am J Perinatol 2009;26:335-43. [Crossref] [PubMed]
- Grisaru-Granovsky S, Reichman B, Lerner-Geva L, et al. Population-based trends in mortality and neonatal morbidities among singleton, very preterm, very low birth weight infants over 16 years. Early Hum Dev 2014;90:821-7. [Crossref] [PubMed]
- Lee SK, Aziz K, Singhal N, et al. Improving the quality of care for infants: a cluster randomized controlled trial. CMAJ 2009;181:469-76. [Crossref] [PubMed]
- Hossain S, Shah PS, Ye XY, et al. Outcome comparison of very preterm infants cared for in the neonatal intensive care units in Australia and New Zealand and in Canada. J Paediatr Child Health 2015;51:881-8. [Crossref] [PubMed]
- Rüegger C, Hegglin M, Adams M, et al. Population based trends in mortality, morbidity and treatment for very preterm- and very low birth weight infants over 12 years. BMC Pediatr 2012;12:17. [Crossref] [PubMed]
- Ancel PY, Goffinet F, Kuhn P, et al. Survival and Morbidity of Preterm Children Born at 22 Through 34 Weeks’ Gestation in France in 2011: Results of the EPIPAGE-2 Cohort Study. JAMA Pediatr 2015;169:230-8. Erratum in: Error in group information and figure. [JAMA Pediatr 2015]. [Crossref] [PubMed]
- Shah PS, Dunn M, Aziz K, et al. Sustained quality improvement in outcomes of preterm neonates with a gestational age less than 29 weeks: results from the Evidence-based Practice for Improving Quality Phase 31. Can J Physiol Pharmacol 2019;97:213-21. [Crossref] [PubMed]
- Stoll BJ, Hansen NI, Bell EF, et al. Trends in Care Practices, Morbidity, and Mortality of Extremely Preterm Neonates, 1993-2012. JAMA 2015;314:1039-51. [Crossref] [PubMed]
- Ohlin A, Björkman L, Serenius F, et al. Sepsis as a risk factor for neonatal morbidity in extremely preterm infants. Acta Paediatr 2015;104:1070-6. [Crossref] [PubMed]
- Kim JK, Chang YS, Sung S, et al. Trends in the incidence and associated factors of late-onset sepsis associated with improved survival in extremely preterm infants born at 23–26 weeks’ gestation: a retrospective study. BMC Pediatr 2018;18:172. [Crossref] [PubMed]
- Stoll BJ, Hansen N, Fanaroff AA, et al. Late-Onset Sepsis in Very Low Birth Weight Neonates: The Experience of the NICHD Neonatal Research Network. Pediatrics 2002;110:285-91. [Crossref] [PubMed]
- Al Wohoush I, Rivera J, Cairo J, et al. Comparing clinical and microbiological methods for the diagnosis of true bacteraemia among patients with multiple blood cultures positive for coagulase-negative staphylococci. Clin Microbiol Infect 2011;17:569-71. [Crossref] [PubMed]
- Plsek PE. Quality Improvement Methods in Clinical Medicine. Pediatrics 1999;103:203-14. [PubMed]
- Puopolo KM. Epidemiology of Neonatal Early-onset Sepsis. NeoReviews 2008;9:e571-9. [Crossref]
- Heino A, Gissler M, Hindori-Mohangoo AD, et al. Variations in Multiple Birth Rates and Impact on Perinatal Outcomes in Europe. PLoS One 2016;11:e0149252. [Crossref] [PubMed]
- Walsh MC, Kliegman RM. Necrotizing Enterocolitis: Treatment Based on Staging Criteria. Pediatr Clin North Am 1986;33:179-201. [Crossref] [PubMed]
- Gordon PV, Swanson JR, Attridge JT, et al. Emerging trends in acquired neonatal intestinal disease: is it time to abandon Bell’s criteria? J Perinatol 2007;27:661-71. [Crossref] [PubMed]
- Soll RF. Progress in the care of extremely preterm infants. JAMA 2015;314:1007-8. [Crossref] [PubMed]
- Leistner R, Piening B, Gastmeier P, et al. Nosocomial Infections in Very Low Birthweight Infants in Germany: Current Data from the National Surveillance System NEO-KISS. Klin Pädiatr 2013;225:75-80. [Crossref] [PubMed]
- Horbar JD, Soll RF, Edwards WH. The Vermont Oxford Network: A Community of Practice. Clin Perinatol 2010;37:29-47. [Crossref] [PubMed]
- Spitzer AR. Has Quality Improvement Really Improved Outcomes for Babies in the Neonatal Intensive Care Unit? Clin Perinatol 2017;44:469-83. [Crossref] [PubMed]
- Tsai MH, Hsu JF, Chu SM, et al. Incidence, Clinical Characteristics and Risk Factors for Adverse Outcome in Neonates With Late-onset Sepsis. Pediatr Infect Dis J 2014;33:e7. [Crossref] [PubMed]
- Piening BC, Geffers C, Gastmeier P, et al. Pathogen-specific mortality in very low birth weight infants with primary bloodstream infection. PLoS One 2017;12:e0180134. [Crossref] [PubMed]
- Makhoul IR, Sujov P, Smolkin T, et al. Pathogen-Specific Early Mortality in Very Low Birth Weight Infants with Late-Onset Sepsis: A National Survey. Clin Infect Dis 2005;40:218-24. [Crossref] [PubMed]
- Dong Y, Glaser K, Speer CP. Late-onset sepsis caused by Gram-negative bacteria in very low birth weight infants: a systematic review. Expert Rev Anti Infect Ther 2019;17:177-88. [Crossref] [PubMed]
- Al-Taiar A, Hammoud MS, Cuiqing L, et al. Neonatal infections in China, Malaysia, Hong Kong and Thailand. Arch Dis Child Fetal Neonatal Ed 2013;98:F249-55. [Crossref] [PubMed]
- Rello J, Sa-Borges M, Correa H, et al. Variations in Etiology of Ventilator-associated Pneumonia across Four Treatment Sites. Am J Respir Crit Care Med 1999;160:608-13. [Crossref] [PubMed]
- Rhee C, Kadri SS, Danner RL, et al. Diagnosing sepsis is subjective and highly variable: a survey of intensivists using case vignettes. Crit Care 2016;20:89. [Crossref] [PubMed]
- Nash C, Chu A, Bhatti M, et al. Coagulase Negative Staphylococci in the Neonatal Intensive Care Unit: Are We Any Smarter? NeoReviews 2013;14:e284-93. [Crossref]
- Hedegaard SS, Wisborg K, Hvas AM. Diagnostic utility of biomarkers for neonatal sepsis--a systematic review. Infect Dis (Lond) 2015;47:117-24. [Crossref] [PubMed]
- Chiesa C, Natale F, Pascone R, et al. C reactive protein and procalcitonin: reference intervals for preterm and term newborns during the early neonatal period. Clin Chim Acta 2011;412:1053-9. [Crossref] [PubMed]
- Pammi M, Flores A, Leeflang M, et al. Molecular assays in the diagnosis of neonatal sepsis: a systematic review and meta-analysis. Pediatrics 2011;128:e973-85. [Crossref] [PubMed]
- Verstraete EH, Blot K, Mahieu L, et al. Prediction Models for Neonatal Health Care–Associated Sepsis: A Meta-analysis. Pediatrics 2015;135:e1002-14. [Crossref] [PubMed]
- Stoll BJ, Hansen N, Fanaroff AA, et al. Changes in pathogens causing early-onset sepsis in very-low-birth-weight infants. N Engl J Med 2002;347:240-7. [Crossref] [PubMed]
- Shane AL, Stoll BJ. Neonatal sepsis: Progress towards improved outcomes. J Infect 2014;68:S24-32. [Crossref] [PubMed]
- Cotten CM. Adverse Consequences of Neonatal Antibiotic Exposure. Curr Opin Pediatr 2016;28:141-9. [Crossref] [PubMed]
- Clark RH, Bloom BT, Spitzer AR, et al. Reported medication use in the neonatal intensive care unit: data from a large national data set. Pediatrics 2006;117:1979-87. [Crossref] [PubMed]
- Oeser C, Lutsar I, Metsvaht T, et al. Clinical trials in neonatal sepsis. J Antimicrob Chemother 2013;68:2733-45. [Crossref] [PubMed]
- Sivanandan S, Soraisham AS, Swarnam K. Choice and duration of antimicrobial therapy for neonatal sepsis and meningitis. Int J Pediatr 2011;2011:712150. [Crossref] [PubMed]
- Patel SJ, Oshodi A, Prasad P, et al. Antibiotic Use in Neonatal Intensive Care Units and Adherence with Centers for Disease Control and Prevention 12 Step Campaign to Prevent Antimicrobial Resistance. Pediatr Infect Dis J 2009;28:1047-51. [Crossref] [PubMed]
- Soll RF, Edwards WH. Antibiotic Use in Neonatal Intensive Care. Pediatrics 2015;135:928-9. [Crossref] [PubMed]
- Charron AC, Carl MA, Warner BB, et al. Determinants of Initial Antibiotic Duration in Very Low Birth Weight Neonates. Infect Dis Ther 2019;8:209-17. [Crossref] [PubMed]
- Schulman J, Dimand RJ, Lee HC, et al. Neonatal Intensive Care Unit Antibiotic Use. Pediatrics 2015;135:826-33. [Crossref] [PubMed]
- Leroux S, Zhao W, Bétrémieux P, et al. Therapeutic guidelines for prescribing antibiotics in neonates should be evidence-based: a French national survey. Arch Dis Child 2015;100:394-8. [Crossref] [PubMed]
- Metsvaht T, Nellis G, Varendi H, et al. High variability in the dosing of commonly used antibiotics revealed by a Europe-wide point prevalence study: implications for research and dissemination. BMC Pediatr 2015;15:41. [Crossref] [PubMed]
- Choosing Wisely | Promoting conversations between providers and patients [Internet]. [cited 2019 Apr 28]. Available online: http://www.choosingwisely.org/
- Ho T, Dukhovny D, Zupancic JA, et al. Choosing Wisely in Newborn Medicine: Five Opportunities to Increase Value. Pediatrics 2015;136:e482-9. [Crossref] [PubMed]
- Davey P, Marwick CA, Scott CL, et al. Interventions to improve antibiotic prescribing practices for hospital inpatients. Cochrane Database Syst Rev 2017;2:CD003543. [PubMed]
- Ho T, Buus-Frank ME, Edwards EM, et al. Adherence of Newborn-Specific Antibiotic Stewardship Programs to CDC Recommendations. Pediatrics 2018;142:e20174322. [Crossref] [PubMed]
- Hull MA, Fisher JG, Gutierrez IM, et al. Mortality and Management of Surgical Necrotizing Enterocolitis in Very Low Birth Weight Neonates: A Prospective Cohort Study. J Am Coll Surg 2014;218:1148-55. [Crossref] [PubMed]
- Rich BS, Dolgin SE. Necrotizing Enterocolitis. Pediatr Rev 2017;38:552-9. [Crossref] [PubMed]
- Robinson JR, Rellinger EJ, Hatch LD, et al. Surgical necrotizing enterocolitis. Semin Perinatol 2017;41:70-9. [Crossref] [PubMed]
- Graham PL, Begg MD, Larson E, et al. Risk Factors for Late Onset Gram-Negative Sepsis in Low Birth Weight Infants Hospitalized in the Neonatal Intensive Care Unit Pediatr Infect Dis J 2006;25:113-7. [Crossref] [PubMed]
- Downey LC, Smith PB, Benjamin DK. Risk factors and prevention of late-onset sepsis in premature infants. Early Hum Dev 2010;86:7-12. [Crossref] [PubMed]
- Perlman SE, Saiman L, Larson EL. Risk factors for late-onset health care–associated bloodstream infections in patients in neonatal intensive care units. Am J Infect Control 2007;35:177-82. [Crossref] [PubMed]
- Aziz K, McMillan DD, Andrews W, et al. Variations in rates of nosocomial infection among Canadian neonatal intensive care units may be practice-related. BMC Pediatr 2005;5:22. [Crossref] [PubMed]
- Ahle M, Drott P, Andersson RE. Epidemiology and Trends of Necrotizing Enterocolitis in Sweden: 1987–2009. Pediatrics 2013;132:e443-51. [Crossref] [PubMed]
- Patel BK, Shah JS. Necrotizing enterocolitis in very low birth weight infants: a systemic review. ISRN Gastroenterol 2012;2012:562594. [Crossref] [PubMed]
- Gephart SM, McGrath JM, Effken JA, et al. Necrotizing enterocolitis risk: state of the science. Adv Neonatal Care 2012;12:77-87. [Crossref] [PubMed]
- Ohlsson A, Walia R, Shah SS. Ibuprofen for the treatment of patent ductus arteriosus in preterm or low birth weight (or both) infants. Cochrane Database Syst Rev 2015;2:CD003481. [PubMed]
- Boyce JM, Pittet D; Healthcare Infection Control Practices Advisory Committee, et al. Guideline for Hand Hygiene in Health-Care Settings. Recommendations of the Healthcare Infection Control Practices Advisory Committee and the HICPAC/SHEA/APIC/IDSA Hand Hygiene Task Force. Society for Healthcare Epidemiology of America/Association for Professionals in Infection Control/Infectious Diseases Society of America. MMWR Recomm Rep 2002;51:1-45, quiz CE1-4.
- Bolon MK. Hand Hygiene: An Update. Infect Dis Clin North Am 2016;30:591-607. [Crossref] [PubMed]
- Cunningham D, Brilli RJ, McClead RE, et al. The Safety Stand-down: A Technique for Improving and Sustaining Hand Hygiene Compliance Among Health Care Personnel. J Patient Saf 2018;14:107-11. [Crossref] [PubMed]
- Pessoa-Silva CL, Hugonnet S, Pfister R, et al. Reduction of Health Care–Associated Infection Risk in Neonates by Successful Hand Hygiene Promotion. Pediatrics 2007;120:e382-90. [Crossref] [PubMed]
- Raskind CH, Worley S, Vinski J, et al. Hand Hygiene Compliance Rates After an Educational Intervention in a Neonatal Intensive Care Unit. Infect Control Hosp Epidemiol 2007;28:1096-8. [Crossref] [PubMed]
- Schmid S, Geffers C, Wagenpfeil G, et al. Preventive bundles to reduce catheter-associated bloodstream infections in neonatal intensive care. GMS Hyg Infect Control 2018;13:Doc10. [PubMed]
- Pogorzelska-Maziarz M. The Use and Effectiveness of Bundles for Prevention of Central Line–Associated Bloodstream Infections in Neonates: A Review of the Literature. J Perinat Neonatal Nurs 2016;30:148-59. [Crossref] [PubMed]
- O’Grady NP, Alexander M, Burns LA, et al. Guidelines for the prevention of intravascular catheter-related infections. Am J Infect Control 2011;39:S1-34. [Crossref] [PubMed]
- Miller MR, Griswold M, Harris JM, et al. Decreasing PICU catheter-associated bloodstream infections: NACHRI’s quality transformation efforts. Pediatrics 2010;125:206-13. [Crossref] [PubMed]
- Steiner M, Langgartner M, Cardona F, et al. Significant Reduction of Catheter-associated Blood Stream Infections in Preterm Neonates After Implementation of a Care Bundle Focusing on Simulation Training of Central Line Insertion. Pediatr Infect Dis J 2015;34:1193-6. [Crossref] [PubMed]
- Underwood MA. Human milk for the premature infant. Pediatr Clin North Am 2013;60:189-207. [Crossref] [PubMed]
- Meier PP. Human Milk and Clinical Outcomes in Preterm Infants. Nestle Nutr Inst Workshop Ser 2019;90:163-74. [Crossref] [PubMed]
- de Silva A, Jones PW, Spencer SA. Does human milk reduce infection rates in preterm infants? A systematic review. Arch Dis Child Fetal Neonatal Ed 2004;89:F509-13. [Crossref] [PubMed]
- Lechner BE, Vohr BR. Neurodevelopmental Outcomes of Preterm Infants Fed Human Milk: A Systematic Review. Clin Perinatol 2017;44:69-83. [Crossref] [PubMed]
- Neu J, Walker WA. Necrotizing Enterocolitis. N Engl J Med 2011;364:255-64. [Crossref] [PubMed]
- Ofek Shlomai N, Deshpande G, Rao S, et al. Probiotics for preterm neonates: what will it take to change clinical practice? Neonatology 2014;105:64-70. [Crossref] [PubMed]
- Taylor RS. Probiotics to prevent necrotizing enterocolitis: Too cheap and easy? Paediatr Child Health 2014;19:351-2. [Crossref] [PubMed]
- Tarnow-Mordi W, Soll RF. Probiotic Supplementation in Preterm Infants: It Is Time to Change Practice. J Pediatr 2014;164:959-60. [Crossref] [PubMed]
- Modi N. Probiotics and Necrotising Enterocolitis: The Devil (as Always) Is in the Detail. Neonatology 2014;105:71-3. [Crossref] [PubMed]
- Ohlsson A, Lacy JB. Intravenous immunoglobulin for preventing infection in preterm and/or low birth weight infants. Cochrane Database Syst Rev 2013.CD000361. [PubMed]
- Pammi M, Haque KN. Pentoxifylline for treatment of sepsis and necrotizing enterocolitis in neonates. Cochrane Database Syst Rev 2015.CD004205. [PubMed]
- ANZCTR - Trial Registration 12616000405415 [Internet]. [cited 2019 Apr 29]. Available online: https://www.anzctr.org.au/Trial/Registration/TrialReview.aspx?id=370404&isReview=true
- Schröder C, Schwab F, Behnke M, et al. Epidemiology of healthcare associated infections in Germany: Nearly 20 years of surveillance. Int J Med Microbiol 2015;305:799-806. [Crossref] [PubMed]