Management of the sensitized pediatric heart transplant patient
Introduction
Orthotopic heart transplantation has been an accepted therapy for children with end stage heart disease since the 1980s. Over the last 3 decades, advancements in peri-operative management and transplant immunosuppression have led to significantly improved outcomes, with an overall median survival of 16 years among all pediatric heart transplant recipients, and up to 22 years in those transplanted as infants (1). Furthermore, refined management of congenital heart disease and advanced technologies for managing critically ill patients awaiting transplantation means that more and more children are surviving to the point where transplant becomes an option. However, despite these successes in pediatric heart failure and transplant management, children who are immunologically sensitized to human leukocyte antigen (HLA) remain at increased risk for morbidity and mortality, both while awaiting and after transplant. In this review we will discuss the epidemiology of sensitization, review the immunologic basis and methods of HLA antibody detection, describe outcomes for sensitized transplant candidates, and consider both pre- and post-transplant management options for sensitized patients.
HLA, anti-HLA antibodies, and transplantation
HLAs are cell-surface proteins that aid the immune system in recognition of self vs. non-self. HLA proteins are encoded by genes in the major histocompatibility complex (MHC) located on chromosome 6. Class I MHC proteins (HLA-A, HLA-B, and HLA-C) are expressed on nearly all nucleated cells, while class II MHC (HLA-DR, HLA-DQ, and HLA-DP) expression is limited to B cells, monocytes, dendritic cells, and other antigen presenting cells. In the setting of organ transplantation, both class I and class II HLA can be expressed by vascular endothelial cells of the donor organ, where mismatched donor HLA can be recognized as “non-self” by the recipient’s circulating immune system, thus becoming targets for antibody-mediated injury. The primary mechanism for antibody-mediated graft injury is thought to be via activation of the classical complement cascade, which triggers an inflammatory response leading to endothelial cell injury, microvascular thrombosis, and eventual graft dysfunction (2). Antibody-mediated graft injury can also occur by complement-independent pathways when activated HLA antibodies crosslink at sites other than the Fc receptor, initiating cytokine release and aberrations in intracellular cell signaling (3). While improved understanding of these mechanisms has helped to develop monitoring and treatment strategies which will be discussed in this review, much is still to be learned about what triggers antibody development and which antibodies are clinically significant.
What we do know is that the presence of pre-existing anti-HLA antibodies in a transplant recipient—termed “sensitization”—poses a high risk for early antibody-mediated rejection (AMR) and is associated with worse outcomes. Sensitization typically occurs after an immunologic challenge to non-self material, such as blood transfusions, pregnancy, prior organ transplantation, and/or mechanical circulatory support (MCS) devices (4-6). In children, exposure to human homograft tissue during surgical palliation of congenital heart disease is another important risk factor (7). Sensitized transplant candidates are often subject to longer waitlist times—and consequently higher waitlist mortality—as the availability of HLA compatible donors is limited (6). Pre-transplant sensitization is also associated with increased risk of rejection, cardiac allograft vasculopathy (CAV), and overall mortality in both adult (8) and pediatric (4) heart transplant recipients, especially when donor-specific HLA antibodies (DSA) are identified (9).
DSA can also develop after transplant. New antibody formation can be triggered by re-exposure to previously recognized HLA (a so-called “memory response”, commonly involving class I antibodies), or DSA can develop truly de novo (often later post-transplant, and often class II antibodies) (10). Both the timing and HLA class specificity of DSA development can have clinical implications. Multiple studies have demonstrated that late forming and persistent DSAs are more detrimental than early and/or transient DSAs (9,11,12). And while class I DSA have been associated with acute rejection (13), class II antibodies have been consistently associated with the development of CAV and chronic rejection (11,14).
More recently, antibodies to non-HLA antigens such as vimentin, MHC class I polypeptide-related sequence A (MICA), angiotensin and endothelin receptors have also been implicated in antibody-mediated injury of the graft (15,16). However, the true clinical significance of these antibodies remains largely unknown, and there is no consensus on how best to monitor or manage these antibodies, so this review will focus primarily on HLA specific antibodies.
HLA antibody detection
Several HLA antibody detection assays have been developed to assess a transplant candidate’s HLA antibody load and assess potential donor compatibility. The complement dependent cytotoxic (CDC) assay was first described by Patel and Terasaki in 1969 (17). This cell-based assay involves applying the candidate’s serum to a representative panel of donor T- and B-lymphocytes which express common HLAs, and then adding a source of complement (usually derived from rabbit serum). Complement-fixing HLA antibodies in the candidate serum recognize, bind, and lyse any cells which express those HLA. The number of unique panel cells lysed over the total tested yields a percent panel reactive antibody (PRA). A PRA >10% is considered sensitized. While the CDC assay has the advantage of identifying clinically relevant antibodies (i.e., those that kill donor cells), it is limited by a lack of specificity and sensitivity, variability in technique and interpretation, and the inability to distinguish individual antibody specificities.
More recently, solid phase immunoassays, in which engineered “beads” are coated with one or more HLA antigens, have revolutionized the field of HLA antibody identification. The flow PRA solid phase assay applies candidate serum to a panel of beads which have been coated with class I or class II HLA antigens. Fluorescein tagged anti-human immunoglobulin (anti-IgG) antibody is then added, which binds to any candidate HLA antibody that is bound to HLA antigen on the beads. The tagged anti-IgG antibody is then detected by flow cytometry, yielding a percent PRA for both class I and class II antibodies, although further antibody specificities cannot be identified.
In current practice, most HLA labs use single antigen bead (SAB) technology, in which a single purified or recombinant HLA epitope has been attached to each bead in the panel. Luminex testing is then used to detect which beads have bound antibody from the candidate serum. These assays not only identify how many HLA antibodies are present (yielding a percent PRA), but they can also determine HLA-antibody specificities and how strongly they bind, as measured by mean fluorescent intensity (MFI). Importantly, while MFI does provide some quantification of antibody binding strength and bead saturation, it may not correlate directly with antibody strength in vivo given that the “expression” of each HLA allele on its bead may not reflect the true expression of that particular allele on actual donor cells. In fact, there is still no consensus as to what MFI cutoff corresponds to a “clinically relevant” antibody. Typically, MFI levels greater than 1,000 are considered “positive”, while levels more than 5,000 are considered “clinically important”, but in practice, cutoffs are institution-dependent, based on collaboration between clinician and HLA lab expertise (18).
One of the primary challenges of SAB assays is that they can detect antibodies that do not fix complement, which may not be clinically relevant (5). To address this concern, a modified SAB assay has been developed to distinguish complement-fixing from non-complement fixing antibodies by identifying which antibodies bind C1q, the first step in the complement cascade. C1q assays have been shown to more accurately predict a positive crossmatch and antibody mediated rejection in heart transplant recipients (19-21). Additionally, assays detecting antibodies which can bind and activate C3d and C4d—further down the complement cascade—have been found to correlate with an increased risk of AMR (22) and poor long-term cardiac graft outcome (23).
If specific HLA-antibodies are detected, the transplant program can choose which, if any, HLA must be avoided in a donor, termed “unacceptable antigens” (UAs). The choice of UAs is program dependent, but typically depends on the HLA class, strength of antibody binding (MFI), C1q positivity, and milieu of candidate clinical factors. Once UAs are chosen, a calculated PRA (cPRA) can be generated which provides an estimate of the percentage of organ donors that will be incompatible for a candidate based on national data (24).
When a donor becomes available, a direct crossmatch may be performed to determine the potential reactivity of the candidate HLA-antibody profile to donor HLA. Prospective and retrospective crossmatch assays can be CDC or flow-based and require the HLA laboratory to have both candidate serum and donor cells at hand. Similar to PRA testing, flow crossmatch is more sensitive than CDC (25), but non-complement-fixing antibodies can be detected which may not be clinically relevant. In a study of renal transplant recipients with a negative CDC crossmatch but positive flow crossmatch, flow results had no significant predictive value of acute rejection, graft loss, or death (26).
Retrospective crossmatching is easily and routinely done post-transplant. Although the results are not available until after the transplant is complete, they can be helpful in guiding post-transplant immunosuppression, especially in the event of a positive crossmatch.
Prospective crossmatching, on the other hand, is typically reserved for highly sensitized transplant candidates to help determine the HLA compatibility of a potential donor, before donor acceptance. Unfortunately, the requirement of donor samples can be prohibitive which often leads to increased time on the waitlist. In a cohort of >6,000 pediatric transplant candidates, a requirement for a prospective crossmatch at or during listing was associated with increased waitlist mortality (27).
More recently, the use of SAB to specify recipient HLA-antibody profile allows for comparison to a potential donor HLA type to predict crossmatch results. This process is termed virtual crossmatch and has been shown to have good correlation with both prospective flow-based assays (28,29) and with retrospective crossmatch results (30).
Epidemiology of sensitization
Large pediatric transplant registries have described sensitization rates of between 9–11%, with increasing prevalence in the current era (1,31,32). This trend is likely due to a combination of factors, including increasing numbers of high-risk patients surviving to transplant (i.e., those with palliated congenital heart disease and those requiring mechanical support) and more sensitive anti-HLA antibody detection assays (33). One recent study reported a sensitization rate of 50–60% when PRA detection was limited to the SAB assay with conservative MFI cutoffs (≥1,000 MFI) (34).
The use of cryopreserved human allograft material in congenital heart surgery is a major risk factor for HLA-antibody sensitization in pediatric heart transplant candidates. In a study by Hooper and colleagues, 12 of 13 children receiving allograft material had PRA >50% at 3 months after implantation. At an average of 8 years after surgery, all 12 remained sensitized, although 10 had a lower PRA (35). Hawkins et al. compared sensitization in patients undergoing congenital heart surgery who received allograft versus those who did not, and found that 92% of the allograft group were sensitized (PRA >10%) at 3 months, with 85% remaining sensitized at 1 year. This was compared to 0% when an allograft was not implanted (36). And in a retrospective review of 40 neonates with hypoplastic left heart syndrome, Ideen and colleagues compared sensitization rates between those palliated by Norwood operation using allograft material for arch reconstruction vs. those palliated by a hybrid approach using ductal stent and pulmonary artery banding (no allograft). Patients exposed to allograft during the Norwood operation had significantly higher rates of sensitization compared to those who underwent a hybrid palliation (38% vs. 0%, P=0.005), with 85% of sensitized allograft recipients being highly sensitized (PRA >50%) (37).
Children requiring MCS are also at increased risk for HLA sensitization (34). Potential triggers for HLA antibody production in patients requiring MCS include increased exposure to blood products or a possible immunologic response to the textured surfaces of some devices (38). Multiple studies have demonstrated high rates of HLA sensitization in children with left ventricular assist device (LVAD) support (43–66%), with a less prominent HLA antibody response to extracorporeal membrane oxygenation (ECMO) (7%) (39,40). However, while sensitization is common in children requiring MCS, several studies suggest that sensitization in response to MCS may not have as negative an impact as sensitization in patients not supported by MCS. For example, O’Connor and colleagues examined sensitization in children supported by ventricular assist device (VAD) and found that while more than 1/3 of VAD recipients developed PRA >10%, only half of these saw sustained sensitization in long term follow-up (41). A United Network for Organ Sharing (UNOS) registry study involving more than 3,000 pediatric heart transplant recipients, 19% of whom were bridged to transplant with a durable VAD, found no difference in post-transplant outcomes despite a greater rate of sensitization among those supported by a VAD (42% vs. 30%, P<0.001) (42).
Outcomes in sensitized patients
Waitlist mortality
Because sensitized patients have a limited donor pool, they often face longer wait times for transplant and therefore are at higher risk of waitlist mortality. Feingold et al. showed longer waitlist duration (2.6 vs. 1.3 months, P=0.02) and higher waitlist mortality (22% vs. 8.4%, P=0.055) for sensitized patients when a negative prospective crossmatch is required (6). Another study of the Pediatric Heart Transplant Society (PHTS) registry found higher risk of waitlist mortality and lower rates of transplant in sensitized patients (4).
More recently, reports of transplanting across a potential positive crossmatch suggest better waitlist outcomes compared to waiting for a negative prospective crossmatch (6). In a large multicenter observational study—also known as the Clinical Trials in Organ Transplantation in Children (CTOTC) study—Webber and colleagues reported on 290 transplant candidates, more than half (53%) of whom were sensitized (MFI ≥1,000), and 240 of whom underwent transplantation. Despite a shorter waitlist duration for non-sensitized recipients, waitlist mortality was no different for sensitized vs. non-sensitized candidates (6.6% vs. 5.8%, P=0.6) (5).
Rejection
The risk of rejection is highest in sensitized patients transplanted across a positive crossmatch (43,44). In the CTOTC study by Webber et al., only 11 of 143 sensitized patients had a positive CDC crossmatch, and these patients experienced inferior freedom from rejection in positive compared to negative crossmatch recipients (40 vs. 82%, P<0.001) (5). In a smaller series of crossmatch positive transplants, Holt et al. described a 92% incidence of rejection, with a significant proportion of patients experiencing recurrent episodes and rejection with hemodynamic compromise (44). For this reason, most programs will not intentionally transplant across a positive crossmatch, although some centers are using novel immunosuppression protocols to transplant across a weakly positive crossmatch (18). Of note, rejection can also occur in sensitized patients after a negative crossmatch, often due to re-activation of quiescent antibodies upon exposure to the graft. In these cases, rejection can often be successfully treated if identified early and treated aggressively, but the long-term risks of antibody-mediated graft injury may persist.
CAV
The presence of anti-HLA antibodies prior to transplant is associated with increased incidence of CAV (6,32,45). In one single center study of 105 patients, 5-year CAV-free survival was 0% in patients with DSA compared to 25% in patients without (P<0.01) (46). Persistent DSA, particularly to class II HLA-DQ, has been associated with an increased incidence of CAV and graft loss compared to patients with transient or no-DSA (12).
Post-transplant graft loss
Finally, multiple large registry studies have described a correlation between pre-transplant sensitization and post-transplant graft loss (47) as well as all-cause mortality (31,32). The mortality risk appears most pronounced early after transplantation, with several studies showing amelioration of risk beyond the first year (4,32).
As with rejection, the risk of mortality is higher in the setting of a positive crossmatch (4,48). Conversely, when sensitized patients are fortunate to receive a negative crossmatch, survival is comparable to non-sensitized patients (6,49). However, even in the setting of a positive crossmatch, the utilization of peri-operative desensitization therapies has been associated with very acceptable short and medium-term survival (5,50-52).
Management of the sensitized patient
Pre-transplant
Once a transplant candidate is identified as being sensitized, the transplant center must make several decisions, including which UAs to avoid, how often to monitor PRAs, and whether desensitization therapies are indicated.
Most centers use an MFI cutoff of 5,000 to determine which HLA antigens should be avoided in a donor (18), but for highly sensitized patients the list of UAs can be refined with the help of C1q testing and HLA lab expertise. Notably, UAs often need to be adjusted over time, as PRA results can change in response to new sensitizing events or desensitization therapies. It is also important to be aware that certain desensitization therapies [i.e., intravenous immunoglobulin (IVIG)] can interfere with SAB Luminex testing, so the HLA lab must be made aware of these issues when interpreting results. There are no published guidelines as to how frequently to monitor PRAs, and there is significant variability between centers, ranging anywhere between monthly to annually (18,53). Many programs, including ours, adjust the frequency of PRA monitoring based on the initial PRA and the clinical risk for sensitization (Figure 1A).
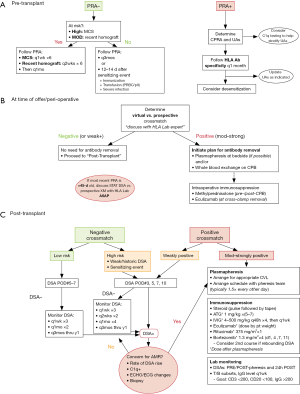
Depending on the degree of sensitization and the clinical status of the patient, medical therapies to reduce the number of circulating antibodies—termed “desensitization”—may be considered.
The threshold for initiating desensitization therapy is center and patient-specific. In a survey of 75 centers participating in an international consensus conference in 2016, 21% of respondents reported using PRA or cPRA threshold of >80% and 21% reported a threshold of >50%, with the remainder using values ranging from >10% to >90% in conjunction with other clinical factors (18). In a recent scientific statement from the American Heart Association, a PRA >50% in patients who have had multiple positive crossmatches was suggested as an indication for desensitization (54).
Once the decision is made to proceed with desensitization, several therapeutic options exist which target various points along the pathway from B-cell activation to antibody production (Figure 2). However, no single therapy has been found to be reliably effective, and in most cases, a combination therapy approach is used (Table 1). Furthermore, very little data exists on the effectiveness of specific desensitization strategies in pediatric heart transplant candidates, such that most of our experience has been extrapolated from adult data and/or from studies in other solid organ transplant candidates or recipients.
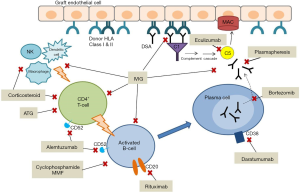
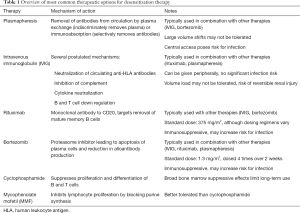
Full table
Plasmapheresis, including therapeutic plasma exchange (TPE) and immunoabsorption, involves extracorporeal mechanical removal of antibodies from the circulation. TPE indiscriminately removes proteins, including not only HLA-antibodies but also clotting factors, which can predispose to coagulopathy. Due to large volume shifts, TPE may not be well tolerated in hemodynamically unstable patients. Immunoabsorption involves passing serum over columns containing IgG to remove antibodies. These modalities require central access, which poses risk of infection. Antibody rebound following plasmapheresis is a well described phenomenon (55), so these methods are rarely if ever used in isolation (56).
IVIG is often used in conjunction with plasmapheresis. The mechanism of action is thought to involve neutralization of circulating anti-HLA antibodies by binding with soluble HLA class I molecules. Other proposed effects include inhibition of complement, neutralization of cytokines, and down regulation of B and T cell activity (57). When used in combination with plasmapheresis, IVIG is effective in reducing PRA and improving mortality (52).
Rituximab is a monoclonal antibody to CD20, a B-cell marker involved in regulation of B-cell development and differentiation. Rituximab causes depletion of mature memory B cells—the precursors of antibody-producing plasma cells—in the peripheral circulation as well as bone marrow and lymph nodes. Balfour and colleagues used rituximab in a sensitized pediatric patient who failed IVIG, mycophenolic acid, and plasmapheresis. Multiple doses of rituximab reduced PRA from 55% to 18%, allowing for a negative crossmatch (58). Schumacher et al. described their experience with a desensitization regimen involving IVIG and rituximab in 14 sensitized pediatric heart transplant candidates. There was a significant reduction in PRA among all patients, with most patients requiring multiple doses of rituximab (47).
Bortezomib is a proteasome inhibitor used primarily for the treatment of relapsed or refractory multiple myeloma (59). Proteasome inhibition leads to apoptosis of rapidly dividing cells. In the setting of solid organ transplant with AMR, bortezomib induces apoptosis in activated plasma cells and reduces DSA production (60). Bortezomib has been used successfully to treat AMR in pediatric heart transplant (61,62), but reports of its use in desensitization prior to heart transplant are limited. May and colleagues utilized bortezomib in a 9-year-old with dilated cardiomyopathy supported by VAD with 100% PRA despite IVIG. With repeated cycles of plasmapheresis followed by rituximab and bortezomib, PRA fell to 0% allowing for a negative crossmatch (63). Patel et al. reported their experience using bortezomib in conjunction with TPE in 7 adults with PRA >50%. Six of the 7 patients experienced significant reduction in PRA, (mean 62% to 35%, P=0.01). However, infection was a common adverse event (64).
Cyclophosphamide is a cytotoxic agent that suppresses proliferation and differentiation of B and T cells. In desensitization, it is typically given via intravenous pulses to limit toxicities encountered during long term oral use. Given the broad bone marrow suppressive effects, cyclophosphamide is not commonly described in contemporary pediatric desensitization protocols.
Mycophenolic acid mofetil (MMF) inhibits lymphocyte proliferation by blocking amino acid purine synthesis. In the sensitized patient, it blocks B-cell proliferation and thus production of HLA-antibodies. Generally, it is better tolerated for long-term immunosuppression than cyclophosphamide.
At time of transplant
When a donor offer is received, a decision must be made whether to proceed with transplant based on virtual and/or prospective crossmatch results. If a positive crossmatch is anticipated, antibody removal at the time of transplant should be arranged. In addition, our center initiates treatment with eculizumab in the operating room for patients with an expected positive crossmatch (Figure 1B).
Eculizumab, a humanized anti-C5 monoclonal antibody, acts at the final step in antibody mediated cellular injury by blocking terminal complement activation. Eculizumab can act quickly to block antibody mediated graft injury even while antibody levels are high, potentially protecting the graft from injury while giving other therapies directed at lowering antibody levels time to work. A phase 4 randomized controlled trial is currently underway to investigate whether eculizumab prevents AMR in sensitized adult cardiac transplant candidates (The De-novo Use of Eculizumab in Presensitized Patients Receiving Cardiac Transplantation; NCT02013037).
Post-transplant
Management of the sensitized patient after transplant is focused on monitoring for the presence of DSA and the prevention/treatment of AMR. International Society for Heart and Lung Transplantation guidelines recommend monitoring for DSA at 1, 3, 6, and 12 months post-transplant (65). More recent consensus documents recommend continued monitoring annually after the first year (18). Sensitized patients, even in the setting of a negative crossmatch, remain at high risk for DSA production and therefore require more frequent monitoring, especially early post-transplant. If at any time DSA are detected and/or there is clinical concern for rejection, AMR-specific therapies should be initiated (Figure 1C).
AMR treatment options are similar to desensitization therapies, again with no single treatment being adequate, such that a combination approach is most often used (62). In addition to plasmapheresis, IVIG, rituximab, and bortezomib, other immunosuppressive agents including corticosteroids, anti-thymoglobulin, and eculizumab are often used to treat AMR—especially in the setting of graft dysfunction—to reduce non-specific inflammation and cell damage, inhibit T-cell mediated activation of B-cells, and block complement mediated endothelial injury.
Alemtuzumab, a recombinant monoclonal antibody to CD52 (found on mature lymphocytes but not plasma cells), is another agent that has been used to treat refractory AMR and early graft failure in small case reports of pediatric heart transplant recipients (66,67).
Future directions
Several novel biological therapies are now being considered in adult and other solid organ transplant recipients which may hold promise for future desensitization and AMR therapies in pediatric heart recipients.
Daratumumab is a monoclonal antibody with specificity against CD38 which is expressed on plasma cells. Like bortezomib, daratumumab was developed and is now used to treat multiple myeloma. A recent case report details the use of daratumumab as salvage therapy in renal transplant AMR refractory to plasma exchange, IVIG, rituximab and eculizumab. Although repeat biopsy after completion of therapy showed no evidence of AMR, the patient developed severe cell mediated rejection and renal function was only marginally improved (68).
Other novel agents in development target the proteasome (carfilzomib), complement system (C1 inhibitor), immunoglobulin degradation (imlifidase), inflammatory cytokines (tocilizumab), and T and B cell stimulation (belimumab and belatacept). Of course, future study will be needed to understand both the benefits and the risks of these therapies in pediatric patients.
Finally, ongoing discovery in the world of transplant immunology will allow us to more precisely identify what triggers antibody production and which antibodies are truly pathologic. For example, we now know that the antigenicity of each HLA molecule is determined by surface amino acid residues, termed eplets (or epitopes), which can vary even among the same allele designation. In kidney transplant recipients, epitope mismatching has been shown to be more predictive (than allele mismatching) of immune-mediated complications such as rejection and graft loss (69), and epitope-mismatch analysis is now being used to identify “acceptable mismatches” in highly sensitized renal transplant candidates (70). Similarly, HLA epitope mismatching has been correlated with adverse events after pediatric heart transplant (71). Although epitope analysis is not yet mainstream in heart transplantation, this technique may someday help to improve the accuracy of virtual crossmatching and tailor immunosuppression protocols for the individual patient.
Conclusions
The number of sensitized pediatric heart transplant candidates is increasing, and the presence of anti-HLA antibodies has implications for successful transplantation and outcomes thereafter. Despite advances in our understanding of transplant immunology and HLA antibody detection, many questions remain, including which antibodies should be treated and what is the best treatment strategy. Future study should focus on elucidating the mechanisms of immune-mediated allograft injury so that new therapeutic targets can be discovered and management can be tailored to the individual patient.
Acknowledgments
None.
Footnote
Conflicts of Interest: The authors have no conflicts of interest to declare.
Ethical Statement: The authors are accountable for all aspects of the work in ensuring that questions related to the accuracy or integrity of any part of the work are appropriately investigated and resolved.
References
- Rossano JW, Cherikh WS, Chambers DC, et al. The International Thoracic Organ Transplant Registry of the International Society for Heart and Lung Transplantation: Twenty-first pediatric heart transplantation report-2018; Focus theme: Multiorgan Transplantation. J Heart Lung Transplant 2018;37:1184-95. [Crossref] [PubMed]
- Colvin MM, Cook JL, Chang P, et al. Antibody-mediated rejection in cardiac transplantation: emerging knowledge in diagnosis and management: a scientific statement from the American Heart Association. Circulation 2015;131:1608-39. [Crossref] [PubMed]
- Akiyoshi T, Hirohashi T, Alessandrini A, et al. Role of complement and NK cells in antibody mediated rejection. Hum Immunol 2012;73:1226-32. [Crossref] [PubMed]
- Mahle WT, Tresler MA, Edens RE, et al. Allosensitization and outcomes in pediatric heart transplantation. J Heart Lung Transplant 2011;30:1221-7. [Crossref] [PubMed]
- Webber S, Zeevi A, Mason K, et al. Pediatric heart transplantation across a positive crossmatch: First year results from the CTOTC-04 multi-institutional study. Am J Transplant 2018;18:2148-62. [Crossref] [PubMed]
- Feingold B, Bowman P, Zeevi A, et al. Survival in allosensitized children after listing for cardiac transplantation. J Heart Lung Transplant 2007;26:565-71. [Crossref] [PubMed]
- Shaddy RE, Hunter DD, Osborn KA, et al. Prospective analysis of HLA immunogenicity of cryopreserved valved allografts used in pediatric heart surgery. Circulation 1996;94:1063-7. [Crossref] [PubMed]
- Nwakanma LU, Williams JA, Weiss ES, et al. Influence of pretransplant panel-reactive antibody on outcomes in 8,160 heart transplant recipients in recent era. Ann Thorac Surg 2007;84:1556-62; discussion 1562-3. [Crossref] [PubMed]
- Ho EK, Vlad G, Vasilescu ER, et al. Pre- and posttransplantation allosensitization in heart allograft recipients: major impact of de novo alloantibody production on allograft survival. Hum Immunol 2011;72:5-10. [Crossref] [PubMed]
- Byku M, Chang PP. Desensitization for sensitized patients awaiting heart transplant. Curr Opin Organ Transplant 2019;24:233-8. [Crossref] [PubMed]
- Smith JD, Banner NR, Hamour IM, et al. De novo donor HLA-specific antibodies after heart transplantation are an independent predictor of poor patient survival. Am J Transplant 2011;11:312-9. [Crossref] [PubMed]
- Irving CA, Carter V, Gennery AR, et al. Effect of persistent versus transient donor-specific HLA antibodies on graft outcomes in pediatric cardiac transplantation. J Heart Lung Transplant 2015;34:1310-7. [Crossref] [PubMed]
- Tambur AR, Pamboukian SV, Costanzo MR, et al. The presence of HLA-directed antibodies after heart transplantation is associated with poor allograft outcome. Transplantation 2005;80:1019-25. [Crossref] [PubMed]
- Topilsky Y, Gandhi MJ, Hasin T, et al. Donor-specific antibodies to class II antigens are associated with accelerated cardiac allograft vasculopathy: a three-dimensional volumetric intravascular ultrasound study. Transplantation 2013;95:389-96. [Crossref] [PubMed]
- Zhang Q, Reed EF. The importance of non-HLA antibodies in transplantation. Nat Rev Nephrol 2016;12:484-95. [Crossref] [PubMed]
- Gates KV, Pereira NL, Griffiths LG. Cardiac Non-Human Leukocyte Antigen Identification: Techniques and Troubles. Front Immunol 2017;8:1332. [Crossref] [PubMed]
- Patel R, Terasaki PI. Significance of the positive crossmatch test in kidney transplantation. N Engl J Med 1969;280:735-9. [Crossref] [PubMed]
- Kobashigawa J, Colvin M, Potena L, et al. The management of antibodies in heart transplantation: An ISHLT consensus document. J Heart Lung Transplant 2018;37:537-47. [Crossref] [PubMed]
- Chin C, Chen G, Sequeria F, et al. Clinical usefulness of a novel C1q assay to detect immunoglobulin G antibodies capable of fixing complement in sensitized pediatric heart transplant patients. J Heart Lung Transplant 2011;30:158-63. [Crossref] [PubMed]
- Chen G, Sequeira F, Tyan DB. Novel C1q assay reveals a clinically relevant subset of human leukocyte antigen antibodies independent of immunoglobulin G strength on single antigen beads. Hum Immunol 2011;72:849-58. [Crossref] [PubMed]
- Reinsmoen NL, Patel J, Mirocha J, et al. Optimizing transplantation of sensitized heart candidates using 4 antibody detection assays to prioritize the assignment of unacceptable antigens. J Heart Lung Transplant 2016;35:165-72. [Crossref] [PubMed]
- Zhang Q, Hickey M, Drogalis-Kim D, et al. Understanding the Correlation Between DSA, Complement Activation, and Antibody-Mediated Rejection in Heart Transplant Recipients. Transplantation 2018;102:e431-8. [Crossref] [PubMed]
- Smith JD, Hamour IM, Banner NR, et al. C4d fixing, luminex binding antibodies - a new tool for prediction of graft failure after heart transplantation. Am J Transplant 2007;7:2809-15. [Crossref] [PubMed]
- Organ Procurement and Transplantation Network. CPRA Calculator. Available online: https://optn.transplant.hrsagov/resources/allocation-calculators/cpra-calculator/
- Vaidya S, Partlow D, Susskind B, et al. Prediction of crossmatch outcome of highly sensitized patients by single and/or multiple antigen bead luminex assay. Transplantation 2006;82:1524-8. [Crossref] [PubMed]
- Wen R, Wu V, Dmitrienko S, et al. Biomarkers in transplantation: Prospective, blinded measurement of predictive value for the flow cytometry crossmatch after negative antiglobulin crossmatch in kidney transplantation. Kidney Int 2006;70:1474-81. [Crossref] [PubMed]
- Feingold B, Park SY, Comer DM, et al. Outcomes after listing with a requirement for a prospective crossmatch in pediatric heart transplantation. J Heart Lung Transplant 2013;32:56-62. [Crossref] [PubMed]
- Bielmann D, Honger G, Lutz D, et al. Pretransplant risk assessment in renal allograft recipients using virtual crossmatching. Am J Transplant 2007;7:626-32. [Crossref] [PubMed]
- Zangwill SD, Ellis TM, Zlotocha J, et al. The virtual crossmatch--a screening tool for sensitized pediatric heart transplant recipients. Pediatr Transplant 2006;10:38-41. [Crossref] [PubMed]
- Zangwill S, Ellis T, Stendahl G, et al. Practical application of the virtual crossmatch. Pediatr Transplant 2007;11:650-4. [Crossref] [PubMed]
- Rossano JW, Morales DL, Zafar F, et al. Impact of antibodies against human leukocyte antigens on long-term outcome in pediatric heart transplant patients: an analysis of the United Network for Organ Sharing database. J Thorac Cardiovasc Surg 2010;140:694-9, 699.e1-2.
- Kirk R, Edwards LB, Kucheryavaya AY, et al. The Registry of the International Society for Heart and Lung Transplantation: thirteenth official pediatric heart transplantation report--2010. J Heart Lung Transplant 2010;29:1119-28. [Crossref] [PubMed]
- Dipchand AI, Kirk R, Naftel DC, et al. Ventricular Assist Device Support as a Bridge to Transplantation in Pediatric Patients. J Am Coll Cardiol 2018;72:402-15. [Crossref] [PubMed]
- Zuckerman WA, Zeevi A, Mason KL, et al. Study rationale, design, and pretransplantation alloantibody status: A first report of Clinical Trials in Organ Transplantation in Children-04 (CTOTC-04) in pediatric heart transplantation. Am J Transplant 2018;18:2135-47. [Crossref] [PubMed]
- Hooper DK, Hawkins JA, Fuller TC, et al. Panel-reactive antibodies late after allograft implantation in children. Ann Thorac Surg 2005;79:641-4; discussion 645. [Crossref] [PubMed]
- Hawkins JA, Breinholt JP, Lambert LM, et al. Class I and class II anti-HLA antibodies after implantation of cryopreserved allograft material in pediatric patients. J Thorac Cardiovasc Surg 2000;119:324-30. [Crossref] [PubMed]
- Ideen C, Albers E, Warner P, et al. Effect of initial surgical palliation on allosensitization and post-transplant outcomes in infants with hypoplastic left heart syndrome. J Heart Lung Transplant 2014;33:1178-80. [Crossref] [PubMed]
- Itescu S, John R. Interactions between the recipient immune system and the left ventricular assist device surface: immunological and clinical implications. Ann Thorac Surg 2003;75:S58-65. [Crossref] [PubMed]
- Yang J, Schall C, Smith D, et al. HLA sensitization in pediatric pre-transplant cardiac patients supported by mechanical assist devices: the utility of Luminex. J Heart Lung Transplant 2009;28:123-9. [Crossref] [PubMed]
- Hong BJ, Delaney M, Guynes A, et al. Human leukocyte antigen sensitization in pediatric patients exposed to mechanical circulatory support. ASAIO J 2014;60:317-21. [Crossref] [PubMed]
- O'Connor MJ, Menteer J, Chrisant MR, et al. Ventricular assist device-associated anti-human leukocyte antigen antibody sensitization in pediatric patients bridged to heart transplantation. J Heart Lung Transplant 2010;29:109-16. [Crossref] [PubMed]
- Castleberry C, Zafar F, Thomas T, et al. Allosensitization does not alter post-transplant outcomes in pediatric patients bridged to transplant with a ventricular assist device. Pediatr Transplant 2016;20:559-64. [Crossref] [PubMed]
- Richmond ME, Hsu DT, Mosca RS, et al. Outcomes in pediatric cardiac transplantation with a positive HLA cross-match. Pediatr Transplant 2012;16:29-35. [Crossref] [PubMed]
- Holt DB, Lublin DM, Phelan DL, et al. Mortality and morbidity in pre-sensitized pediatric heart transplant recipients with a positive donor crossmatch utilizing peri-operative plasmapheresis and cytolytic therapy. J Heart Lung Transplant 2007;26:876-82. [Crossref] [PubMed]
- Kobayashi D, Du W, L'ecuyer TJ. Predictors of cardiac allograft vasculopathy in pediatric heart transplant recipients. Pediatr Transplant 2013;17:436-40. [Crossref] [PubMed]
- Tran A, Fixler D, Huang R, et al. Donor-specific HLA alloantibodies: Impact on cardiac allograft vasculopathy, rejection, and survival after pediatric heart transplantation. J Heart Lung Transplant 2016;35:87-91. [Crossref] [PubMed]
- Schumacher KR, Almond C, Singh TP, et al. Predicting graft loss by 1 year in pediatric heart transplantation candidates: an analysis of the Pediatric Heart Transplant Study database. Circulation 2015;131:890-8. [Crossref] [PubMed]
- Wright EJ, Fiser WP, Edens RE, et al. Cardiac transplant outcomes in pediatric patients with pre-formed anti-human leukocyte antigen antibodies and/or positive retrospective crossmatch. J Heart Lung Transplant 2007;26:1163-9. [Crossref] [PubMed]
- Scott V, Williams RJ, Levi DS. Outcomes of cardiac transplantation in highly sensitized pediatric patients. Pediatr Cardiol 2011;32:615-20. [Crossref] [PubMed]
- Asante-Korang A, Amankwah EK, Lopez-Cepero M, et al. Outcomes in highly sensitized pediatric heart transplant patients using current management strategies. J Heart Lung Transplant 2015;34:175-81. [Crossref] [PubMed]
- Pollock-BarZiv SM, den Hollander N, Ngan BY, et al. Pediatric heart transplantation in human leukocyte antigen sensitized patients: evolving management and assessment of intermediate-term outcomes in a high-risk population. Circulation 2007;116:I172-8. [Crossref] [PubMed]
- Daly KP, Chandler SF, Almond CS, et al. Antibody depletion for the treatment of crossmatch-positive pediatric heart transplant recipients. Pediatr Transplant 2013;17:661-9. [PubMed]
- Betkowski AS, Graff R, Chen JJ, et al. Panel-reactive antibody screening practices prior to heart transplantation. J Heart Lung Transplant 2002;21:644-50. [Crossref] [PubMed]
- Colvin MM, Cook JL, Chang PP, et al. Sensitization in Heart Transplantation: Emerging Knowledge: A Scientific Statement From the American Heart Association. Circulation 2019;139:e553-78. [Crossref] [PubMed]
- Yamada C, Ramon DS, Cascalho M, et al. Efficacy of plasmapheresis on donor-specific antibody reduction by HLA specificity in post-kidney transplant recipients. Transfusion 2015;55:727-35. [Crossref] [PubMed]
- Kobashigawa J, Mehra M, West L, et al. Report from a consensus conference on the sensitized patient awaiting heart transplantation. J Heart Lung Transplant 2009;28:213-25. [Crossref] [PubMed]
- Kazatchkine MD, Kaveri SV. Immunomodulation of autoimmune and inflammatory diseases with intravenous immune globulin. N Engl J Med 2001;345:747-55. [Crossref] [PubMed]
- Balfour IC, Fiore A, Graff RJ, et al. Use of rituximab to decrease panel-reactive antibodies. J Heart Lung Transplant 2005;24:628-30. [Crossref] [PubMed]
- Moreau P, San Miguel J, Ludwig H, et al. Multiple myeloma: ESMO Clinical Practice Guidelines for diagnosis, treatment and follow-up. Ann Oncol 2013;24 Suppl 6:vi133-7. [Crossref] [PubMed]
- Perry DK, Burns JM, Pollinger HS, et al. Proteasome inhibition causes apoptosis of normal human plasma cells preventing alloantibody production. Am J Transplant 2009;9:201-9. [Crossref] [PubMed]
- Morrow WR, Frazier EA, Mahle WT, et al. Rapid reduction in donor-specific anti-human leukocyte antigen antibodies and reversal of antibody-mediated rejection with bortezomib in pediatric heart transplant patients. Transplantation 2012;93:319-24. [Crossref] [PubMed]
- Thrush PT, Pahl E, Naftel DC, et al. A multi-institutional evaluation of antibody-mediated rejection utilizing the Pediatric Heart Transplant Study database: Incidence, therapies and outcomes. J Heart Lung Transplant 2016;35:1497-504. [Crossref] [PubMed]
- May LJ, Yeh J, Maeda K, et al. HLA desensitization with bortezomib in a highly sensitized pediatric patient. Pediatr Transplant 2014;18:E280-2. [Crossref] [PubMed]
- Patel J, Everly M, Chang D, et al. Reduction of alloantibodies via proteasome inhibition in cardiac transplantation. J Heart Lung Transplant 2011;30:1320-6. [Crossref] [PubMed]
- Costanzo MR, Dipchand A, Starling R, et al. The International Society of Heart and Lung Transplantation Guidelines for the care of heart transplant recipients. J Heart Lung Transplant 2010;29:914-56. [Crossref] [PubMed]
- Raj S, Ruiz P, Rusconi P. Early primary graft failure after a pediatric heart transplant and successful rescue with plasmapheresis, immunoglobulins, and alemtuzumab. Ann Pediatr Cardiol 2017;10:69-71. [Crossref] [PubMed]
- Das B, Dimas V, Guleserian K, et al. Alemtuzumab (Campath-1H) therapy for refractory rejections in pediatric heart transplant recipients. Pediatr Transplant 2017. [Crossref] [PubMed]
- Jordan SC, Vescio R, Toyoda M, et al. Daratumumab for Treatment of Antibody-Mediated Rejection in a Kidney Transplant Recipient [abstract]. Am J Transplant 2019;19 (suppl 3).
- Do Nguyen HT, Wong G, Chapman JR, et al. The Association Between Broad Antigen HLA Mismatches, Eplet HLA Mismatches and Acute Rejection After Kidney Transplantation. Transplant Direct 2016;2:e120. [Crossref] [PubMed]
- Albers E, Jorgensen N, Friedland-Little J, et al. HLA Eplet Mismatching is Associated with Increased Risk of Graft Loss in Pediatric Heart Transplant Recipients. J Heart Lung Transplant 2017;36:S78. [Crossref]
- Sullivan PM, Warner P, Kemna MS, et al. HLA molecular epitope mismatching and long-term graft loss in pediatric heart transplant recipients. J Heart Lung Transplant 2015;34:950-7. [Crossref] [PubMed]