The role of inducible costimulatory molecular ligand (ICOSL) in children with neutrophilic asthma
Introduction
Bronchial asthma has been widely researched due to its recurrent nature, its effects on children’s growth and development, the financial burden it imposes, and the resulting reduction in quality of life. There are approximately 30 million asthmatic patients in China, and children with asthma account for almost one-third of the total number of cases (1). The pathogenesis of asthma is complex. Th1–Th2 imbalance, which is considered the dominant factor in Th2 imbalance, is the most important immunological mechanism involved in the pathogenesis of eosinophilic asthma. However, it alone is not sufficient to explain the development of all asthma types (2).
Bronchial asthma is the most common noninfectious disease in children, with approximately 300 million asthmatic patients being diagnosed worldwide. The prevalence of this disease has increased significantly in middle and low-income countries in recent years (3,4). It is believed that the increase of eosinophils is one of the characteristics of airway inflammation in asthma. However, in recent years, it has been shown that certain patients do not exhibit increased sputum eosinophil numbers, and more than 50% of asthmatic subjects present with neutrophil infiltration of airway inflammation (5,6). Studies have shown that infection contributes to the exacerbation of neutrophilic asthma (7,8). Neutrophil Extracellular Traps (NETs) are formed in the airways of some patients with asthma. NETs can damage the airway epithelium and trigger an inflammatory response, thereby exacerbating the severity of neutrophil asthma (9,10). The number of neutrophils is also elevated during exacerbation and increased with the duration of asthma (5,6).
Most patients with eosinophilic asthma are sensitive to corticosteroid, but the curative effect of neutrophil asthma is not obvious. Some patients with acute severe asthma were treated with recombinant human DNA enzyme (rhDNase) and inhaled corticosteroid, which could reduce the viscosity of sputum and improve lung ventilation (11). Some asthma patients only respond to specific biological inhibitors and need to use relevant biomarkers. For patients with high levels of eosinophil count and IgE in sputum, mepolizumab and omalizumab achieved better therapeutic effect (12,13). However, no significant progress has been made in the treatment of neutrophil asthma. The role of neutrophil infiltration in the treatment of severe asthma, fatal asthma, chronic persistent asthma, and refractory asthma has been increasingly acknowledged (14-16). However, the main pathogenesis of neutrophil infiltration in asthma is not fully understood. It is thus important to study and understand the role of neutrophil infiltration in the pathogenesis of asthma.
Inducible co-stimulator ligand (ICOSL) also known as B7-H2, B7h, GL50, or B7RP-1, is an important member of the ICOS/ICOSL signaling pathway together with its receptor, ICOS. It has been shown that the ICOS/ICOSL signaling pathway is associated with inflammatory response, tumor and transplant rejection, and the development of autoimmune diseases (17). However, research on asthma is rare. In the present study, we investigated the expression levels of ICOSL in peripheral blood and bronchoalveolar lavage fluid (BALF) samples from children with neutrophilic asthma, and its correlation with the cytokine secretion of Th1, Th2, and Th17. The clinical significance of the expression of ICOSL in peripheral blood and BALF samples of children with neutrophilic asthma and the association between the concentration levels of cytokines is also discussed.
We present the following article in accordance with the MDAR reporting checklist (available at http://dx.doi.org/10.21037/tp-20-172).
Methods
Study design
Collection of peripheral blood and BALF
Samples were collected from children with asthma within 24 h following admission, and from children who required removal of foreign bodies prior to surgery. A total of 2 mL of sample was collected from peripheral blood and BALF of children who required electronic bronchoscopy. The samples were stored at −80 °C following centrifugation and used for the detection of cytokines.
Clinical data collection
The allergen test in children with asthma was performed to assess allergies. At the same time, the clinical data of the children with asthma and of the control group were collected.
Cytokine detection
Samples of BALF and plasma were obtained for cytokine and ICOSL detection from the children at the time of admission and at discharge. The samples were immediately centrifuged and preserved at −80 °C for subsequent assays. Cytokines were detected using enzyme-linked immunosorbent assay (ELISA). The levels of ICOSL, IFN-γ, IL-4, IL-17, neutrophil elastase (NE), and matrix metalloproteinase-9 (MMP-9) were assessed with ELISA kits (R&D systems, USA).
ELISA measure method
- Detection of ICOSL concentration in peripheral blood and BALF by ELASA. Dilute the ICOSL standard product to 10, 5, 2.5, 1.25, 0.625, 0.312, 0.156 ng/mL, a total of seven concentrations. Set blank wells (without sample), standard wells, and sample wells to be tested. The blood sample to be tested is diluted 5 times, and BALF is not diluted. Add 100 µL/well to the enzyme-labeled coating plate, cover with sealing film, 37 °C Incubate for 1 h. Discard the liquid and spin dry without washing. Add 100 µL of A working solution to each well, cover the ELISA plate with membrane, 37 °C Incubate for 1 h. Add about 350 µL of the washing solution to the microtiter plate, let it stand for 1–2 min, tap the microtiter plate on absorbent paper to remove all the liquid in the well, repeat the plate washing 3 times; after the last washing, pipette or pour take out the remaining washing buffer, buckle the enzyme-labeled plate upside down on absorbent paper, and blot all the remaining liquid in the well. Add 100 µL of B working solution to each well, cover the ELISA plate with membrane, 37 °C Incubate for 30 min. Discard the solution in the well, spin dry, and wash the plate 5 times. Add 90 µL of TMB substrate solution to each well, cover the ELISA plate, and develop color at 37 °C in the dark (control the reaction time at 10–20 min, not more than 30 min. When the first 3–4 wells of the standard wells have obvious gradient blue, stop when the gradient of the last 3–4 holes is not obvious). Add 50 mL of stop solution to each well to stop the reaction. At this time, the blue color is yellow. The order of adding the stop solution should be as consistent as possible with the order of adding the substrate solution. If the color is uneven, please gently shake the plate to make the solution evenly mixed. After ensuring that there are no water droplets at the bottom of the microplate and no bubbles in the wells, immediately use a microplate reader to measure the optical density (O.D. value) of each well at a wavelength of 450 nm. According to the OD value of the standard, draw its standard curve, calculate the concentration, and multiply it by the dilution factor.
- Detection of NE concentration in peripheral blood and BALF by ELASA. Standard wells: dilute NE standards to 1,200, 600, 300, 150, 75 pg/mL, five concentrations in total. Sample addition: set blank holes (no sample added), standard holes, and sample holes to be tested. Add 50 µL of different concentration standards to each standard hole. Dilute the sample to be tested 2.5 times, BALF is not diluted, add 50 µL/well to the enzyme-labeled coated plate, and then add 50 µL of the enzyme-labeled reagent. Shake gently, cover with sealing film, and incubate at 37 °C for 60 minutes. Washing: add about 300 µL/well of the washing solution to the ELISA plate, let it stand for 30 s, discard the liquid, spin dry, wash the plate 5 times, buckle the ELISA plate upside down on absorbent paper, and leave the residue in the well Soak up all the liquid. Color development: add 50 µL of color developer A and 50 µL of color developer B to each well of the ELISA plate, shake gently to mix, and develop color at 37 °C for 10 min in the dark. Stop: add 50 µL stop solution to each well to stop the reaction (in this case, the blue vertical transmission is yellow). Measurement: adjust the blank hole to zero, and measure the absorbance (OD value) of each hole in sequence with a wavelength of 450 nm. According to the concentration and OD value, the regression equation of standard curve was drawn by elisacalc, and the concentration was calculated.
- Detection of IFN-γ concentration in peripheral blood and BALF by ELASA. Dilute the IFN-γ standard to: 480, 240, 120, 60, 30 pg/mL; the rest of the steps are the same as the NE steps.
- Detection of IL-4 concentration in peripheral blood and BALF by ELASA. Dilute the IL-4 standard to: 320, 160, 80, 40, 20 pg/mL, a total of five concentrations; the rest of the steps are the same as the NE steps.
- Detection of IL-17 concentration in peripheral blood and BALF by ELASA. Dilute the IL-17 standard to: 160, 80, 40, 20, 10 pg/mL, the rest of the steps are the same as the NE steps.
- Detection of MMP9 concentration in peripheral blood and BALF by ELASA. Dilute the MMP9 standard to: 480, 240, 120, 60, 30 ng/mL, a total of five concentrations; the rest of the steps are the same as the NE steps.
Allergen detection: the allergic condition of the children was evaluated using blood allergen or skin allergen origin prick tests.
Participants
Children who were admitted to the Respiratory Medicine, Children’s Hospital of Soochow University from August 2014 to July 2016 with the diagnostic criteria for asthma (18) were selected. Participants were excluded if detected positive for the following: BALF pathogen, which included respiratory syncytial virus, adenovirus, influenza virus A, B, parainfluenza virus [1, 2, 3], Boka virus, human metapneumovirus, rhinovirus, mycoplasma, chlamydia pneumonia, or tuberculosis and bacteriological pathogen.
A total of 32 asthmatic children were recruited and classified according to the BALF cell criterion of more than 50% neutrophils (the percentage of neutrophils in BALF was in the 95th percentile in the control group) (19). They were grouped into either the neutrophilic asthma group (NA group) or the asthma group (A group).
Concomitantly, children that were examined with the electronic bronchoscope for the presence of foreign bodies in Children’s Hospital of Soochow University composed the control group (C group). A total of 16 cases were selected (11 males and 5 females). The C group did not present with allergic diseases and exhibited no previous history of this type of disease. The C group included subjects that had gone nearly 4 weeks without the use of any drugs and who did not exhibit a previous history of infection. In the C group, the percentage of neutrophils in BALF was in the 95th percentile.
The present study was approved by the ethics committee of the Children’s Hospital of Soochow University. The guardians of the children provided signed informed consent, and all the children with asthma were treated with conventional treatment (20). Depending on the condition of the children, they were administered with inhaled corticosteroids or oral corticosteroids, oxygen, suitable fluid support, and other comprehensive support.
The present study was performed according to the protocol approved by the ethics committee of the Children’s Hospital of Soochow University. All procedures performed in this study involving human participants were in accordance with the Declaration of Helsinki (as revised in 2013).
Statistical analysis
The data were analyzed using the PASW 18.0 statistical package, and the measurement data were expressed as mean ± SD (standard deviation). The count data are expressed as percentages or rates. The rank-sum test was used for comparison between the groups, and the kappa consistency test was used for consistency analysis. The differences were considered significant with a P value <0.05.
Results
General information
A total of 32 children with asthma were enrolled in the present study. All children with asthma were admitted to the hospital with cough and wheezing symptoms, including 2 cases with oxygen inhalation prior to or following admission in the NA group. A total of 3 cases obtained from outpatient or admission were treated by methylprednisolone and hydrocortisone sodium succinate intravenous administration. A total of 12 children inhaled Pulmicort Respules more than 3 times, and 1 case was treated with oxygen inhalation prior to or following admission to the A group. A total of 6 cases from outpatient visits or admission were treated by methylprednisolone and hydrocortisone sodium succinate intravenously. The length of hospital stay in the NA group was the longest with hospitalization lasting between 6 and 21 days, while the length of hospitalization of the A group lasted between 2 and 15 days. The C group exhibited the shortest hospitalization time ranging between 4 and 10 days. The differences were significant (P<0.05, Table 1).
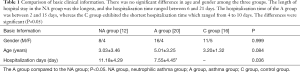
Full table
Assessment of allergic conditions in the three groups of children
In the NA group, 9 cases presented with eczema or positive allergen, 7 cases with eczema, 3 cases with dust mite allergy, 2 cases with freshwater fish allergy, and 2 cases with seafood allergy. In group A, 15 cases presented with eczema history or allergen-positive history, 11 cases with eczema, 5 cases with dust mite allergy, 2 cases with fungal allergy, 2 cases with egg allergy, 1 case with fish allergy, and 1 case with shrimp allergy. No history of eczema and positive allergens was noted in the subjects of the C group.
Cytokine levels in peripheral blood and BALF samples
Comparison of the levels of cytokines in the peripheral blood of patients
The mean ICOSL levels of the NA group were 10.06±1.01, which were significantly higher than those in the A group (8.16±1.25) and the C group (7.19±2.34) (P<0.05). The concentration levels of NE, IL-17, IL-4, and MMP-9 of the NA group were 445.48±40.79, 254.15±50.50, 217.88±90.74, and 561.42±427.76, respectively, which were significantly higher than those noted in the A group (319.39±51.44, 127.02±43.10, 180.50±81.24, and 258.73±114.46, respectively) and in the C group (267.54±47.18, 146.27±46.67, 97.63±68.65, and 309.74±159.44 respectively) (P<0.05). The concentration levels of NE and IL-4 in group A were significantly higher than those in group C. The concentration levels of IFN-γ in groups NA and A were 307.81±73.52 and 285.88±91.23 respectively, which were significantly lower than those of the C group (397.11±72.91; Figure 1).
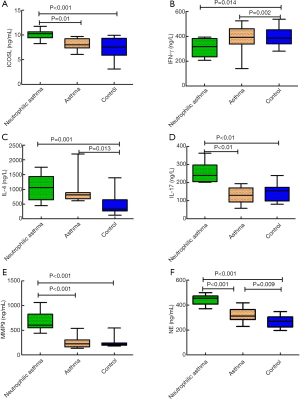
Comparison of the levels of cytokines in BALF
The concentration levels of ICOSL in the NA group (0.98±0.09) were significantly higher than those of the A group (0.33±0.09) and the C group (0.43±0.21; P<0.05). The mean concentration levels of NE, IL-17, and MMP-9 in the NA group were 37.70±6.93, 18.47±2.33, and 58.62±11.30 respectively, which were significantly higher than those of the A group (15.87±9.17, 9.59±3.52, and 40.16±18.19 respectively; P<0.05) and of the C group (27.33±6.01, 6.69±4.09, 27.66±15.74, respectively; P<0.05). The concentration levels of MMP-9 in the A group were significantly higher than those in the C group. The expression levels of IFN-γ in the A group were 12.42±3.32, which were lower than those in the NA group (16.32±3.76) and the C group (22.21±3.09). The differences were significant (P<0.05). IL-17 levels in the NA group were significantly higher than those in the C group, whereas the concentration levels of IL-4 in the NA and A groups were significantly higher than those in the C group (Figure 2).
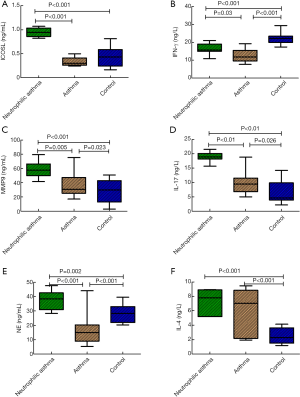
Correlation analysis of ICOSL, IFN-γ, NE, IL-17, and MMP-9 in peripheral blood and BALF samples
- Correlation analysis of ICOSL, IFN-γ, NE, IL-17, and MMP-9 levels in peripheral blood: the concentration levels of ICOSL and IL-17 in peripheral blood samples exhibited a positive correlation, (r=0.753, P=0.012), whereas those of ICOSL, IFN-γ, NE, MMP-9, and IL-4 were less consistent (r=0.633, 0.117, 0.753, 0.217, 0.233; P>0.05; Table 2).
- Correlation analysis of ICOSL, IFN-γ, NE, IL-17, and MMP-9 levels in BALF samples: the concentration levels of ICOSL and IL-17 in BALF samples exhibited a positive correlation (r=0.774; P=0.009), whereas ICOSL, IFN-γ, NE, MMP-9, and IL-4 levels were less consistent (r=0.111, 0.420, 0.774, 0.253, 0.096; P>0.05; Table 3).
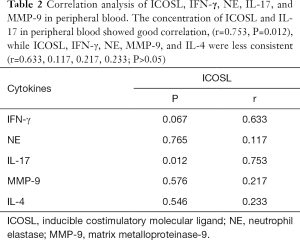
Full table
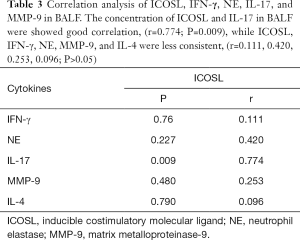
Full table
Discussion
Previous studies have demonstrated that more than 50% of asthmatic cases involve neutrophil infiltration. This trend can also be observed in patients with severe asthma, fatal asthma, infantile asthma, chronic persistent asthma, and refractory asthma. The present study detected the concentration levels of ICOSL in children with neutrophilic asthma in peripheral blood and BALF samples and explored their correlation with the concentration levels of Th1, Th2, and Th17 type cytokines.
The results indicated that the cytokines secreted by Th2 cells, notably IL-4, in BALF and peripheral blood samples of the NA and A groups were higher than those of the C group. In BALF, the concentration levels of IFN-γ, which represented Th1, were significantly lower in the NA group than in the A and C groups. These results suggest a Th1–Th2 imbalance in children with neutrophilic asthma and asthma. The cytokines secreted by type Th2 appeared hyperactive. The data further demonstrated apparent Th1 cell dysfunction in children with neutrophilic asthma.
The pathogenesis of asthma is complicated, with epithelial cells, fibroblasts, dendritic cells, neutrophils, eosinophils, mast cells, T lymphocytes, and other cells involved in chronic airway inflammation (21). It has been proposed that Th2-related cytokines and a series of pathological processes caused by mucous secretion, cell proliferation, and eosinophil infiltration, are involved in the pathogenesis and maintenance of allergic asthma, which in turn correlate positively with the severity of disease symptoms. In contrast to these observations, IFN-γ secretion by Th1 cells can inhibit the proliferation of Th2 and play an inhibitory role in inflammation (2). The Th1–Th2 imbalance is characterized by the relative inhibition of Th1 function and relative hyperfunction of Th2 function, which causes the increase in IgE synthesis and the production of IL-4, IL-5, IL-13, and other Th2 cytokines. These processes are considered the main pathogenetic mechanisms of asthma.
IL-4 is a pleiotropic cytokine secreted by CD4+T cell subsets, B cells, and mast cells. As a characteristic cytokine secreted by Th2 cells, it exhibits potent chemical chemotactic activity to eosinophils, neutrophils, and other inflammatory cells. It can significantly increase the accumulation and activation of inflammatory cells in the airways, thereby inducing exacerbations of acute asthma (22,23). IFN-γ is a cytokine that has been studied recently and can inhibit the proliferation of B lymphocytes and the secretion of IgG1 and IgE in B lymphocytes. In addition, it can also inhibit the expression of low affinity receptors for IgE on B cells. IFN-γ can block allergic reactions and relieve asthma symptoms, enhancing the immune function and exerting non-specific anti-infection activity. Moreover, it can enhance phagocytic function and inhibit the survival of plasma cells, which play a protective role in the pathogenesis of asthma (24). In recent years, it has been revealed that neutrophil infiltration in bronchial biopsy and induced sputum is responsible for the severe or acute exacerbation of the condition of asthmatic patients. The products of neutrophils mainly include NE, MMPs (mainly MMP-9), and IL-17 (10,25-27). NE mainly degrades basement membrane elastin, which is closely associated with lung tissue damage. Increased levels of NE in neutrophils can result in severe acute lung injury and acute respiratory distress syndrome (ARDS). NE further promotes neutrophil recruitment and persists in the inflammatory response at accumulation sites. Lock et al. (28) indicated that in BALF samples, NE levels were significantly higher in patients with acute exacerbation of severe asthma and in patients with severe asthmatic respiratory tract submucosal inflammation, regardless of the presence of eosinophils. The concentration levels of MMP-9 and NE correlated positively. In recent years, increasing attention has been paid to the association between the number of Th17 cells in asthma, notably in severe asthma, and the resistance to steroid treatment of asthma. Previous studies demonstrated that Th17/IL-17 activation could aggravate airway hyperresponsiveness and airway inflammation in asthma, whereas the degree of IL-17 elevation was closely associated with the severity of asthma (29-31).
In the present study, IFN-γ, NE, IL-17, MMP-9, and IL-4 levels were detected in the peripheral blood and BALF samples of children with neutrophilic asthma, children with asthma, and control subjects. It was found that the NE, IL-17, and MMP-9 concentration level in the peripheral blood of the NA group patients was significantly higher than those in the A and C groups. The IFN-γ level in the NA and A group was significantly lower than those in the C groups. The concentration level of IL-4 in the NA and A groups was significantly higher than those in the C group. The concentration level of NE and MMP-9 in the BALF samples was significantly higher in the NA group than in the A and C groups. The concentration level of MMP-9 in the A group was higher than that in the C group. The concentration level of IFN-γ in the A group was lower than those in the NA and C groups. The IL-17 levels in the NA group were significantly higher than those in the C group. The concentration level of IL-4 in the NA and A groups was significantly higher than that in the C group. The results suggested an imbalance of Th1–Th2–Th17 immune function in the NA and A groups. This effect was more severe in the NA group. The clinical data of the NA, A, and C groups were analyzed and compared. The analysis indicated that the NA group exhibited the longest hospitalization and more severe Th1–Th2–Th17 immune imbalance. The more difficult the clinical treatment, the longer the required treatment time.
The ICOSL molecule is the main member of the CD28/B7 superfamily (32), by virtue of its activation in T cell polarization. ICOSL causes optimization of Th1/Th2 subsets and the same type of immunoglobulin conversion plays an important role in this process. ICOSL binds to its receptor ICOS to activate the ICOS-ICOSL signaling pathway. Previous studies on ICOSL have mainly focused on inflammatory diseases, autoimmune diseases, and tumors. ICOSL is highly expressed in inflammatory diseases (33) and is closely associated with the severity of inflammation. However, a limited number of studies have been conducted on asthma, and the conclusions are not clear. Matesic et al. (34) transplanted ovalbumin (OVA)-specific T cell receptor transgenic ICOS plus T cells into OVA-sensitized BALB/c mice yielding a significantly increased number of lymphocytes, macrophages, neutrophils, and eosinophils in BALF. However, Akbari et al. used allergen-induced mouse asthma models to investigate the role of ICOS/ICOSL signaling pathways and found that ICOS stimulated the production of regulatory T cells. The production of Treg cells depends on the expression of high levels of ICOSL by lung dendritic cells. Treg cells inhibit the function of antigen-specific T cells and the formation of AHR (airway hyperresponsiveness) (35,36). The present study examined the levels of ICOSL in peripheral blood and BALF samples from children with neutrophilic asthma. The levels of ICOSL, IFN-γ, NE, IL-17, and MMP-9 were evaluated in peripheral blood and BALF samples. The results suggested that the concentration levels of ICOSL in peripheral blood and BALF samples exhibited a positive correlation with IL-17 levels. It was suggested that ICOSL may regulate IL-17 secretion by Th17 to recruit neutrophils in the airways, degranulate neutrophils, and increase the secretion of inflammatory mediators. These processes increase the adhesion of neutrophils on endothelial cells in the respiratory tract, which in turn are involved in the occurrence and development of asthma.
Conclusions
The present study demonstrated a Th1–Th2–Th17 imbalance of in children with neutrophilic asthma and asthma. The immune imbalance was more severe in the former, which was more difficult to treat and required longer hospitalization time. The concentration level of ICOSL in the peripheral blood and BALF samples of children with neutrophilic asthma was higher than those that in normal subjects. ICOSL may regulate the secretion of Th17 by IL-17, increase neutrophil recruitment in the airway, raise NE and MMP-9 levels, and participate in the development of immunity and inflammation in neutrophilic asthma. The results of the present study may be limited due to the small sample size. Therefore, the pathogenesis of asthma requires further exploration.
Acknowledgments
Funding: Funding was provided by the National Science Foundation of China (grant to Zhengrong Chen, no. 81970027 and 81771676; grant to Li Huang, no. 81971490; grant to Yongdong Yan, no. 81870006), the Science and Technology Projects for the Youth of Suzhou (grant to Li Huang, no. KJXW2015013), the Research Project of Provincial Health and Family Planning Commission (grant to Li Huang, no. H201622), the Social Development Projects of Jiangsu Province (grant to Zhengrong Chen, no. BE2019671), and the Key Lab of Respiratory Disease of Suzhou (grant to Chuangli Hao, no. SZS201714).
Footnote
Reporting Checklist: The authors have completed the MDAR reporting checklist. Available at http://dx.doi.org/10.21037/tp-20-172
Data Sharing Statement: Available at http://dx.doi.org/10.21037/tp-20-172
Conflicts of Interest: All authors have completed the ICMJE uniform disclosure form (available at http://dx.doi.org/10.21037/tp-20-172). The authors have no conflicts of interest to declare.
Ethical Statement: The authors are accountable for all aspects of the work in ensuring that questions related to the accuracy or integrity of any part of the work are appropriately investigated and resolved. This study was conducted after gaining approval from the Ethics Committee of Children’s Hospital of Soochow University (No.2020CS068). Informed consent was obtained from the guardians of all individual participants included in the study. All procedures performed in this study involving human participants were in accordance with the Declaration of Helsinki (as revised in 2013).
Open Access Statement: This is an Open Access article distributed in accordance with the Creative Commons Attribution-NonCommercial-NoDerivs 4.0 International License (CC BY-NC-ND 4.0), which permits the non-commercial replication and distribution of the article with the strict proviso that no changes or edits are made and the original work is properly cited (including links to both the formal publication through the relevant DOI and the license). See: https://creativecommons.org/licenses/by-nc-nd/4.0/.
References
- Keeney GE, Gray MP, Morrison AK, et al. Dexamethasone for Acute Asthma Exacerbations in Children: A Meta-analysis. Pediatrics 2014;133:493-9. [Crossref] [PubMed]
- Adams M, Bassler D. Practice variations and rates of late onset sepsis and necrotizing enterocolitis in very preterm born infants, a review. Transl Pediatr 2019;8:212-26. [Crossref] [PubMed]
- Lasso-Pirot A, Delgado-Villalta S, Spanier AJ. Early childhood wheezers: identifying asthma in later life. J Asthma Allergy 2015;8:63-73. [PubMed]
- Lin J, Xing B, Chen P, et al. Chinese expert consensus-based guideline on assessment and management of asthma exacerbation. J Thorac Dis 2019;11:4918-35. [Crossref] [PubMed]
- Chen X, Qiu C. Respiratory tract mucous membrane microecology and asthma. Ann Transl Med 2019;7:495. [Crossref] [PubMed]
- Davies AR, Hancox RJ. Induced sputum in asthma: diagnostic and therapeutic implications. Curr Opin Pulm Med 2013;19:60-5. [Crossref] [PubMed]
- Zhang J, Zhu Z, Zuo X, et al. The role of NTHi colonization and infection in the pathogenesis of neutrophilic asthma. Respir Res 2020;21:170. [PubMed]
- Steinke JW, Lawrence MG, Teague WG, et al. Bronchoalveolar lavage cytokine patterns in children with severe neutrophilic and paucigranulocytic asthma. J Allergy Clin Immunol 2020. [Epub ahead of print]. [Crossref] [PubMed]
- Chen X, Li Y, Qin L, He R, et al. Neutrophil Extracellular Trapping Network Promotes the Pathogenesis of Neutrophil-associated Asthma through Macrophages. Immunol Invest 2020. [Epub ahead of print]. [Crossref] [PubMed]
- Lachowicz-Scroggins ME, Dunican EM, Charbit AR, et al. Extracellular DNA, neutrophil extracellular traps and inflammasome activation in severe asthma. Am J Respir Crit Care Med 2019;199:1076-85. [Crossref] [PubMed]
- Greally P. Human recombinant DNase for mucus plugging in status asthmaticus. Lancet 1995;346:1423-24. [Crossref] [PubMed]
- Fricker M, Heaney LG, Upham JW. Can biomarkers help us hit targets in difficult-to-treat asthma? Respirology 2017;22:430-42. [Crossref] [PubMed]
- Barnes PJ. Targeting cytokines to treat asthma and chronic obstructive pulmonary disease. Nat Rev Immunol 2018;18:454-66. [Crossref] [PubMed]
- Wood LG, Baines KJ, Fu J, et al. The neutrophilic inflammatory phenotype is associated with systemic inflammation in asthma. Chest 2012;142:86-93. [Crossref] [PubMed]
- Choi JS, Jang AS, Park JS, et al. Role of neutrophils in persistent airway obstruction due to refractory asthma. Respirology 2012;17:322-9. [Crossref] [PubMed]
- Nair P, Aziz-Ur-Rehman A, Radford K. Therapeutic implications of 'neutrophilic asthma'. Curr Opin Pulm Med 2015;21:33-8. [Crossref] [PubMed]
- Merrill JT. Co-stimulatory molecules as targets for treatment of lupus. Clin Immunol 2013;148:369-75. [Crossref] [PubMed]
- Global Initiative for Asthma GINA Report: Global Strategy for Asthma Management and Prevention (2020). Available online: https://ginasthma.org/gina-reports/
- Simpson JL, Grissell TV, Douwes J, et al. Innate immune activation in nuetrophlic asthma and bronchiectasis. Thorax 2007;62:211-8. [Crossref] [PubMed]
- Endo Y, Hirahara K, Yagi R, et al. Pathogenic memory type Th2 cells in allergic inflammation. Trends Immunol 2014;35:69-78. [Crossref] [PubMed]
- Arakawa H, Hamasaki Y, Kohno Y, et al. Japanese guidelines for childhood asthma 2017. Allergol Int 2017;66:190-204. [Crossref] [PubMed]
- Oh CK, Geba GP, Molfino N. Investigational therapeutics targeting the IL-4/IL-13/STAT-6 pathway for the treatment of asthma. Eur Respir Rev 2010;19:46-54. [Crossref] [PubMed]
- Lee YC, Lee KH, Lee HB, et al. Serum levels of interleukins (IL)-4, IL-5, IL-13, and interferon-gamma in acute asthma. J Asthma 2001;38:665-71. [Crossref] [PubMed]
- Kunzmann V, Bauer E, Feurle J, et al. Stimulation of gammadelta T cells by aminobisphosphonates and induction of antiplasma cell activity in multiple myeloma. Blood 2000;96:384-92. [Crossref] [PubMed]
- Wenzel SE, Schwartz LB, Langmack EL, et al. Evidence that severe asthma can be divided pathologically into two inflammatory subtypes with distinct physiologic and clinical characteristics. Am J Respir Crit Care Med 1999;160:1001-8. [Crossref] [PubMed]
- Jatakanon A, Uasuf C, Maziak W, et al. Neutrophilic inflammation in severe persistent asthma. Am J Respir Crit Care Med 1999;160:1532-9. [Crossref] [PubMed]
- Panettieri RA Jr. The Role of Neutrophils in Asthma. Immunol Allergy Clin North Am 2018;38:629-38. [Crossref] [PubMed]
- Locke NR, Royce SG, Wainewright JS, et al. Comparison of airway remodeling in acute, subacute, and chronic models of allergic airways disease. Am J Respir Cell Mol Biol 2007;36:625-32. [Crossref] [PubMed]
- Östling J, van Geest M, Schofield JPR, et al. IL-17-high asthma with features of a psoriasis immunophenotype. J Allergy Clin Immunol 2019;144:1198-213. [Crossref] [PubMed]
- Halwani R, Sultana A, Vazquez-Tello A, et al. Th-17 regulatory cytokines IL-21, IL-23, and IL-6 enhance neutrophil production of IL-17 cytokines during asthma. J Asthma 2017;54:893-904. [Crossref] [PubMed]
- Xiong H, Wei L, Peng B. IL. -17 stimulates the production of the inflammatory chemokines IL-6 and IL-8 in human dental pulp fibroblasts. Int Endod J 2015;48:505-11. [Crossref] [PubMed]
- Sharpe AH, Freeman GJ. The B7-CD28 superfamily. Nat Rev Immunol 2002;2:116-26. [Crossref] [PubMed]
- Maazi H, Patel N, Sankaranarayanan I, et al. ICOS:ICOS-ligand interaction is required for type 2 innate lymphoid cell function, homeostasis, and induction of airway hyperreactivity. Immunity 2015;42:538-51. [Crossref] [PubMed]
- Matesic D, Lehmann PV, Heeger PS. High-resolution characterization of cytokine-producing alloreactivity in naive and allograft-primed mice. Transplantation 1998;65:906-14. [Crossref] [PubMed]
- Lambrecht BN, Hammad H. The other cells in asthma: dendritic cell and epithelial cell crosstalk. Curr Opin Pulm Med 2003;9:34-41. [Crossref] [PubMed]
- Kopf M, Coyle AJ, Schmitz N, et al. Inducible costimulator protein (ICOS) controls T helper cell subset polarization after virus and parasite infection. J Exp Med 2000;192:53-61. [Crossref] [PubMed]
(English Language Editors: J. Brown and J. Gray)