Investigation of the association between imbalance of the intestinal flora and infantile spasms: a pilot case-control study
Introduction
Infantile spasm (IS) is a rare and specific form of epilepsy that constitutes a serious threat to the health of infants. IS affects approximately 0.31 in 1,000 live births (1), and typically manifests as a cluster of spasms, hypsarrhythmia on electroencephalograms, and developmental delay (2). More than 200 known pathogenic causes of IS have been identified; however, the specific pathogenic mechanisms underlying IS have yet to be illuminated (3).
At present, adrenocorticotropic hormone (ACTH) is the first-line treatment for IS. However, the effects of ACTH are unsatisfactory, with the treatment attaining success in only 60% of cases. Furthermore, the mechanisms underlying the effects of ACTH on IS are not fully understood. Studies have suggested that ACTH may exert its effects on IS by creating negative feedback on the hypothalamus-pituitary-adrenal (HPA) axis and by inhibiting the release of corticotropin-releasing hormone (CRH) (4). Previous research has revealed IS to differ from other types of epilepsy and that dysfunction of the HPA axis is involved in its onset.
Several studies (5-7) have shown that the intestinal flora (IF) may affect brain function via mechanisms involving the brain-gut axis, and that imbalance of the IF may be associated with the occurrence of epilepsy. It is possible that the IF may participate in the epileptogenic process by mediating the pro-excitatory effects of peripheral inflammation via immune system activation. This process could occur through a series of mechanisms, including proinflammatory cytokine and chemokine release, modulation of neural networks via the production of neurotransmitters (particularly serotonin, γ-aminobutyric acid, and glutamate), and activity involving the balance between excitation and inhibition (E/I balance). The IF can also induce its effects by dysregulating the endocannabinoid system, adjusting the permeability of the gut barrier (for instance, by increasing the levels of lipopolysaccharide), and adjusting both neuroendocrine pathways (e.g., the HPA axis) and neural pathways (e.g., vagus afferents and the enteric nervous system) (5). However, no previous study has investigated the link between the IF and IS.
Here, we attempted to investigate the specific association between the IF and IS. The present study focused on children with IS who were being treated with ACTH in the Pediatrics Department of the First Medical Center of PLA General Hospital and used a group of healthy controls (HCs) for comparison.
We present the following case in accordance with the STROBE reporting checklist (available at http://dx.doi.org/10.21037/tp-20-384).
Methods
Study subjects
This study was conducted in accordance with the Declaration of Helsinki (as revised in 2013). The Ethics Committee of PLA General Hospital (reference no. 20190156) approved the study protocol. For each participant, written informed consent to participate in the study was obtained from the parents or a legal guardian.
From March to September 2019, 23 infants with IS were recruited from the Pediatrics Department of the First Medical Center of PLA General Hospital. All of the included patients met the specific diagnostic criteria for IS, as described by Hrachovy in 2013 (1). The age at onset for all cases was <1 year. The form of onset, the frequency of seizures, electroencephalographic manifestations, and the use of oral antiepileptic drugs (AEDs) prior to admission were recorded for each participant. These data were recorded again for each patient after 14 days of receiving treatment with ACTH (2.5 U/kg, ≤25 U; Biochemical and Pharmaceutical Co., Ltd., Shanghai, China) and magnesium sulfate (0.25 g/kg, ≤2.5 g). Twenty-one healthy infants, aged 6 to 15 months, were also recruited as HCs. None of the participants experienced any obvious problems, such as diarrhea, or respiratory or gastrointestinal infection, in the 4 weeks preceding the study initiation. It was also ensured that none of the subjects had taken any antibiotics or probiotics during the 4 weeks prior to the study commencing.
Collection of fecal samples
Fecal samples were collected from all infants in the study group on the day of admission and after the completion of ACTH treatment. For the collection of samples from the HCs, the infants defecated into containers in a dedicated room in the outpatient department. After collection, all samples were then frozen at –80 °C for analyses.
Response evaluation
At the end of the course of treatment, we evaluated short-term efficacy as a specific response. A “complete response” (CR) denoted no spasms, a “partial response” (PR) denoted a reduction in seizure frequency by >50%, and “no response” (NR) denoted that the frequency of seizures had been reduced by <50% or had not been reduced at all.
Sequencing of 16S rRNA genes
For the analysis of fecal samples from the IS and HC groups, at least 200 mg of fecal material was collected into an Ex-DNA/RNA Extraction Kit (Tianlong Technology Co., LTD., Xi’an, China). Then, a polymerase chain reaction (PCR) was performed to amplify the V3-V4 hypervariable regions of the bacterial 16S rRNA gene using universal primers (319 forward 5´-ACTCCTACGGGAGGCAGCAG-3´; 806 reverse 5´-GGACTACHVGGGTWTCTAAT-3´) incorporating FLX Titanium adaptors and a barcode sequence. Subsequently, we pooled the purified amplicons in equimolar amounts and performed paired-end sequencing on a MiSeq platform (Illumina, San Diego, CA, USA) in accordance with standard protocols described by Majorbio Bio-Pharm Technology (Shanghai, China). Raw FastQ files were demultiplexed and quality-filtered using QIIME (q2; Version 1.50; https://qiime2.org). Then, trimmed sequences were clustered into operational taxonomic units (OTUs) with a 97% similarity cut-off using q2-deblur (https://qiime2.org). Finally, the taxonomic assignment of OTUs was carried out using naïve Bayes classifiers against a model provided by QIIME (gg-13-8-99-515-806-nb-classifier.qza).
Bioinformatics analysis and functional gene annotations
QIIME was also used for the calculation of diversity for the 16S rRNA gene sequencing analysis. Differences in α diversity were calculated using diversity indices (Chao1, Shannon, and Simpson). In contrast, β diversity was determined using weighted UniFrac phylogenetic distance matrices and visualized in principal component analysis (PCA) plots. Statistically significant differences in the relative abundance of genera were identified using linear discriminant analysis (LDA) effect size (LEfSe). Only LDA values >2 were considered to be significantly enriched.
OTUs, as well as the representative sequence obtained by QIIME2, were annotated using Tax4Fun2 (R version 3.5.3; R Institute for Statistical Computing, Vienna, Austria), with reference to the Kyoto Encyclopedia of Genes and Genomes (KEGG) database. PCA and permutational multivariate analyses of variance (Adonis) were also carried out for the comparison of overall differences.
Sample size
Prior to performing the experiments, we determined that a sample size of 21 individuals was needed in each group in order to determine significant differences with a statistical power of 80% with a probability of a type I error of 5%.
Statistical analyses
All statistical analyses were performed in SPSS 21.0 (IBM, Armonk, NY, USA), with P<0.05 considered as the criterion for significance. Descriptive data were presented as the mean ± standard deviation if the data were normally distributed (as determined by the Kolmogorov-Smirnov test) and as the median (with 25th and 75th percentiles) if the data were not normally distributed. The independent-samples t-test was used to identify significant differences in normally distributed data, while the Mann-Whitney rank-sum test was used for data with non-normal distribution. Frequency data were compared using the Chi-squared test and the Fisher’s exact probability method. Differences in metabolism between the 2 groups were identified through PCA and Adonis analyses.
Results
Comparison of clinical data
A total of 23 patients with IS were enrolled in the study. Following 14 days of treatment with ACTH, 18 patients who had a CR or PR to ACTH were identified as the ACTH-response group (ACTH-response group), and the remaining 5 patients, who had no response to ACTH, were identified as the no response group (ACTH-NR group). No significant differences were found between the ACTH-response group and the ACTH-NR group, or between the IS and HC groups, with respect to sex, age, birth mode, gestational age, feeding mode, body mass index (BMI), the number of AEDs, or the provision of supplementary food (P>0.05, Tables 1 and 2).
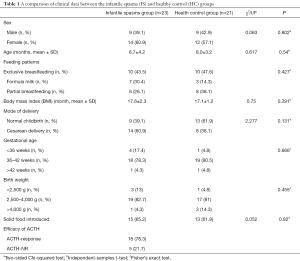
Full table
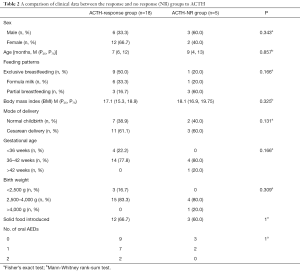
Full table
IF diversity and abundance of the fecal bacterial community
There were no significant differences between the IS and HC groups in terms of the α and β diversity of fecal microbiota (P>0.05). The Mann-Whitney rank-sum test failed to identify any significant differences between the IS and HC groups at the genus level (P>0.05). However, LEfSe revealed that the IS group had reduced populations of Lactobacillus, Roseburia, and Lachnospira, along with an increased population of Clostridium (LDA>2), compared with the control group (Figure 1).
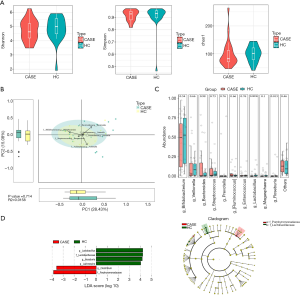
The ACTH-response and ACTH-NR groups showed no significant differences in terms of the α diversity of fecal microbiota (P>0.05); however, there was a significant difference in the β diversity of the 2 groups (P<0.05). The Mann-Whitney rank-sum test failed to identify any significant differences between the IS and HC groups at the genus level (P>0.05). However, in the ACTH-response group, LEfSe demonstrated reductions in the populations of Odoribacter, Phascolarctobacterium, Anaerotruncus, Mitsuokella, and Robinsoniella, as well as an increase in the population of Bifidobacterium (LDA>2), in comparison to the ACTH-NR group (Figure 2).
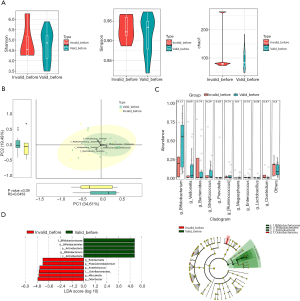
In the IS group, no significant differences were observed in the pre- and post-treatment α and β diversity of fecal microbiota (P>0.05). Also, the Mann-Whitney rank-sum test failed to identify any significant differences between the IS and HC groups at the genus level (P>0.05). LEfSe revealed a reduction in the population of Staphylococcus following ACTH treatment (LDA >2) (Figure 3).
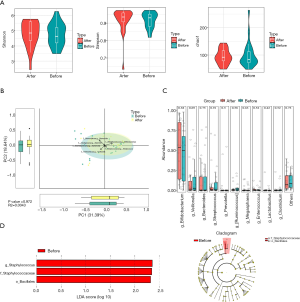
In the IS groups, no significant differences were observed in the α and β diversity of fecal microbiota before and after treatment (P>0.05). Furthermore, neither the Mann-Whitney rank-sum test nor LEfSe identified any significant differences (P>0.05; LDA >2) (Figures 4 and 5).
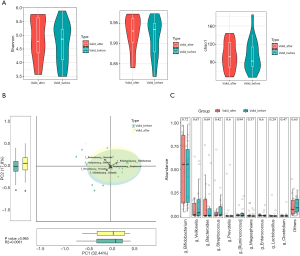
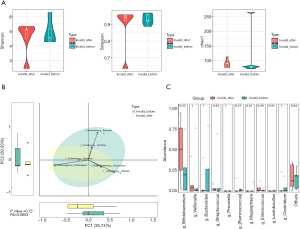
KEGG-based comparison of metabolic pathways between the IS group and HC group based on IF testing
PCA and Adonis were used to determine global metabolic differences in 1st-, 2nd-, and 3rd-level KEGG pathways. Mann-Whitney rank-sum tests were also carried out on 6 1st-level pathways, 45 2nd-level pathways, and 326 3rd-level pathways. The pathway associated with lipoic acid metabolism showed significant differences between the IS group and the HC group. Patients in the IS group showed upregulation of certain genes, including lipB and lipA (P<0.05, Figure 6).
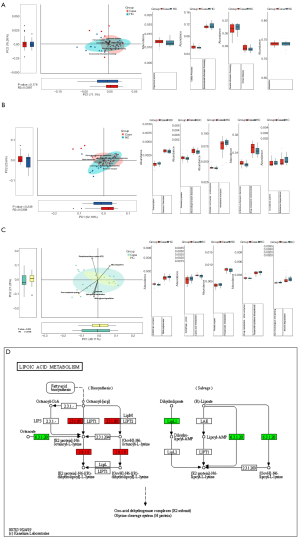
Discussion
In recent years, an increasing number of studies have shown that several mechanisms work together to form the brain-gut axis, thus creating a link between the IF and the brain. As the largest mammalian ecosystem, the intestinal tract normally contains in excess of 100 trillion microbes. This population of microbes can affect the physiological function of the host (8). The theory underlying the microbial-intestinal-brain axis proposes that the IF and the brain have a bidirectional regulatory mechanism that features a complex neuroendocrine pathway (9). Studies have also reported a close relationship between the occurrence of some diseases in the central nervous system, including epilepsy, and the IF (10,11). The pathogenesis of IS may be related to the excessive release of CRH resulting from dysfunctional activity in the HPA axis (4). Recent research has shown that abnormalities in the IF may lead to dysfunction of both the HPA axis and the immune system (12). Consequently, it appears that dysregulation of the IF may also be involved in the occurrence of IS.
The theory underlying the microbial-intestinal-brain axis may help to explain an association of IF with epilepsy. Medel-Matus and colleagues previously showed that the transplantation of gut microbiota from stressed rats to non-stressed rats resulted in a marked reduction in the epilepsy threshold of non-stressed rats and the prolongation of their epilepsy (13). Several clinical studies have also demonstrated significant differences in the IF between patients with treatment-resistant epilepsy and healthy populations. However, several authors have presented different proposals with regard to diversity and the specific classification of these differences. In their study of patients with epilepsy, Peng et al. found that the IF of patients with drug-refractory epilepsy showed elevated levels of α diversity. In particular, the ratio of Bifidobacteria to Lactobacillus was significantly lower in the IF of patients with drug-refractory epilepsy than in HCs, while patients with drug-sensitive epilepsy were more likely to be healthier than those with drug-refractory epilepsy (14). Xie et al. reported that the α diversity of the intestinal microbiota in infants with refractory epilepsy did not differ significantly from that in healthy subjects; however, they did note a significant difference in β diversity (15). Compared with HCs, infants with refractory epilepsy had significantly higher proportions of Bacteroidetes, Prevotella, and Bifidobacterium, and significantly lower proportions of Proteobacteria and Cronobacter (15). Also, in another study, Huang et al. reported a higher proportion of Clostridium in the IF of children with epilepsy (16).
In the present study, we found no significant differences in the α and β diversity of IF between patients with IS and HCs. Hence, our current findings are not entirely consistent with those of previous reports. We also found that the population of Lactobacillus was significantly reduced in children with IS, while the population of Clostridium was significantly higher. Furthermore, we also observed reductions in the populations of Roseburia and Lachnospira. Previously, we reported that mice experiencing prenatal stress undergo excessive activation of the HPA axis (17). This action increases the rate of CRH release and results in a marked reduction in the population of Lactobacillus in the IF (18), and an abundance of the Lactobacillus genus is significantly correlated with the responsiveness of the HPA axis to stress (19). In another study, Peng et al. reported that the addition of Lactobacillus could reduce the excessive activation of the HPA axis (20). Previously, we also reported that IS may result from abnormalities in the HPA axis (17). We therefore speculate that differences in the IF may constitute 1 of the causes of IS and that the mechanisms involved may have an association with abnormalities in the HPA axis. Our current analysis of metabolic pathways highlighted the upregulated expression of genes associated with lipoic acid synthesis in children with IS, a phenomenon which will lead to the production of excessive levels of lipoic acid. Tolunay et al. reported that overproduction of lipoic acid resulted in status epilepticus and that this condition could not be alleviated by a large dose of levetiracetam, midazolam, or dilantin (21). Therefore, we suspect that metabolic changes arising from changes in the IF may be a key trigger of IS.
Additionally, we discovered a significant difference in the β diversity of the IF between children who responded to ACTH treatment and those who did not. Compared with the ACTH-NR group, the ACTH-response group had reduced populations of Odoribacter, Phascolarctobacterium, Anaerotruncus, Mitsuakella, and Robinsoniella. In a previous study, Golubeva et al. showed that stressed prenatal mice exhibited excessive activation of the HPA axis, accompanied by a significant increase in the population of Anaerotruncus (18). We also found a significantly lower proportion of Bifidobacterium in the ACTH-NR group. Some studies have indicated that bacteria from this genus can result the excessive activation of the HPA axis (22). We also recorded and analyzed the number of AEDs but found no significant differences between the 2 groups. Subgroup N03A of the Anatomical Therapeutic Chemical classification system of drugs created by the World Health Organization lists a series of AEDs. The 16 representative medicines in subgroup N03A were tested and none exhibited a clear antimicrobial effect. Therefore, we speculate that differences in the IF might lead to an imbalance in the HPA axis, which is involved in the pathogenic process leading to IS. This imbalance may be more obvious between the ACTH-response and ACTH-NR groups, as the administration of ACTH did not appear to alleviate this issue, resulting in treatment failure in some patients.
Two approaches can be used to regulate the composition of the IF in the treatment of epilepsy: a ketogenic diet (KD) and probiotics (23). A KD is a low-carbohydrate diet which has been demonstrated to have anti-epileptic effects. A range of ketones can be produced during treatment, and these products serve as potent energy sources for gut microbiota (24). A previous study of children with epilepsy showed that after 1 week on a KD, 21% of the children were seizure-free and 43% had >50% seizures (15). The authors also analyzed the IF of the children and reported that treatment with a KD led to a reduction in the proportion of bacteria from the Bacteroidetes and Actinobacteria phyla, and a reduction in bacteria from the Proteobacteria phylum (15). These changes in the IF were believed to be the predominant factor underlying seizure control. Zhang et al. showed that the proportion of bacteria from the beneficial Bacteroidetes phylum was increased in pediatric patients who showed drug-resistance to AEDs, and KD treatment led to a significant reduction in seizure activity within 6 months (25). In another study, Olson et al. observed the seizure-protective effects of a KD by transplanting the fecal microbiota of KD-fed mice into germ-free mice; they noted that the antiepileptic effects disappeared upon the administration of antibiotics (26). In a study of 45 patients with drug-resistant symptomatic epilepsy, a “probiotic cocktail” of beneficial bacteria led to a reduction in seizures of >50% in 29% of patients (27). These findings suggest that regulating the composition of the IF with exogenous interventions could reduce or prevent epilepsy, although further research on this subject is needed.
The main limitation of this study was that it involved a small cohort of patients. This limitation was exacerbated by the patients being divided into groups according to their response to treatment with ACTH. Further research should involve the use of animal models of IF transplantation. Combining such work with metabolomics analysis could also help to provide a far deeper understanding of the pathological mechanisms underlying IS than that we have currently.
Conclusions
Our work suggests that an imbalance in the IF and a change in metabolic pathways may be involved in the pathogenesis of IS. Differences in the composition of the IF appear to affect the efficacy of ACTH treatment. Consequently, the regulation of the IF composition may be beneficial for the treatment of IS and represents a potential strategy for adjuvant therapy.
Acknowledgments
We would like to thank Aegicare (Shenzhen) Technology for providing strong technical support. We would also like to thank the AME editing Group for assistance with language checking.
Funding: This work was funded by the National Natural Science Foundation of China (Reference: 81671279) and the Medical Big Data and Artificial Intelligence Research and Development Project of Chinese PLA General Hospital (Reference: 2019MBD-004).
Footnote
Reporting Checklist: The authors have completed the STROBE reporting checklist. Available at http://dx.doi.org/10.21037/tp-20-384
Data Sharing Statement: Available at http://dx.doi.org/10.21037/tp-20-384
Conflicts of Interest: All authors have completed the ICMJE uniform disclosure form (available at http://dx.doi.org/10.21037/tp-20-384). The authors have no conflicts of interest to declare.
Ethical Statement: The authors are accountable for all aspects of the work in ensuring that questions related to the accuracy or integrity of any part of the work are appropriately investigated and resolved. The study was conducted in accordance with the Declaration of Helsinki (as revised in 2013). This study involved human participants, and the study protocol was approved by the Ethics Committee of PLA General Hospital (Reference no. 20190156). The parents or legal guardians of each participant provided written informed consent for participation in this study.
Open Access Statement: This is an Open Access article distributed in accordance with the Creative Commons Attribution-NonCommercial-NoDerivs 4.0 International License (CC BY-NC-ND 4.0), which permits the non-commercial replication and distribution of the article with the strict proviso that no changes or edits are made and the original work is properly cited (including links to both the formal publication through the relevant DOI and the license). See: https://creativecommons.org/licenses/by-nc-nd/4.0/.
References
- Hrachovy RA, Frost JD Jr. Infantile spasms. Handb Clin Neurol 2013;111:611-8. [Crossref] [PubMed]
- Iype M, Saradakutty G, Kunju PA, et al. Infantile spasms: A prognostic evaluation. Ann Indian Acad Neurol 2016;19:228-35. [Crossref] [PubMed]
- Wallace A, Allen V, Park K, et al. Infantile Spasms and Injuries of Prematurity: Short-Term Treatment-Based Response and Long-Term Outcomes. J Child Neurol 2017;32:861-6. [Crossref] [PubMed]
- Yang XL, Chen B, Zhang XQ, et al. Upregulations of CRH and CRHR1 in the Epileptogenic Tissues of Patients with Intractable Infantile Spasms. CNS Neurosci Ther 2017;23:57-68. [Crossref] [PubMed]
- De Caro C, Iannone LF, Citraro R, et al. Can we 'seize' the gut microbiota to treat epilepsy? Neurosci Biobehav Rev 2019;107:750-64. [Crossref] [PubMed]
- Dahlin M, Prast-Nielsen S. The gut microbiome and epilepsy. EBioMedicine 2019;44:741-6. [Crossref] [PubMed]
- Safak B, Altunan B, Topcu B, et al. The gut microbiome in epilepsy. Microb Pathog 2020;139:103853 [Crossref] [PubMed]
- Liang S, Wu X, Jin F. Gut-Brain Psychology: Rethinking Psychology From the Microbiota-Gut-Brain Axis. Front Integr Neurosci 2018;12:33. [Crossref] [PubMed]
- Cooper AJ, Jeitner TM. Central Role of Glutamate Metabolism in the Maintenance of Nitrogen Homeostasis in Normal and Hyperammonemic Brain. Biomolecules 2016;6. [Crossref] [PubMed]
- Braakman HMH, van Ingen J. Can epilepsy be treated by antibiotics? J Neurol 2018;265:1934-6. [Crossref] [PubMed]
- Arony DA, Gazda S, Kitara DL. Could Nodding Syndrome (NS) in Northern Uganda be an environmentally induced alteration of ancestral microbiota? Pan Afr Med J 2018;31:152. [PubMed]
- Frankiensztajn LM, Elliott E, Koren O. The microbiota and the hypothalamus-pituitary-adrenocortical (HPA) axis, implications for anxiety and stress disorders. Curr Opin Neurobiol 2020;62:76-82. [Crossref] [PubMed]
- Medel-Matus JS, Shin D, Dorfman E, et al. Facilitation of kindling epileptogenesis by chronic stress may be mediated by intestinal microbiome. Epilepsia Open 2018;3:290-4. [Crossref] [PubMed]
- Peng A, Qiu X, Lai W, et al. Altered composition of the gut microbiome in patients with drug-resistant epilepsy. Epilepsy Res 2018;147:102-7. [Crossref] [PubMed]
- Xie G, Zhou Q, Qiu CZ, et al. Ketogenic diet poses a significant effect on imbalanced gut microbiota in infants with refractory epilepsy. World J Gastroenterol 2017;23:6164-71. [Crossref] [PubMed]
- Huang C, Li Y, Feng X, et al. Distinct Gut Microbiota Composition and Functional Category in Children With Cerebral Palsy and Epilepsy. Front Pediatr 2019;7:394. [Crossref] [PubMed]
- Shi XY, Zou LP, Yang G, et al. Prenatal stress exposure hypothesis for infantile spasms. Med Hypotheses 2012;78:735-7. [Crossref] [PubMed]
- Golubeva AV, Crampton S, Desbonnet L, et al. Prenatal stress-induced alterations in major physiological systems correlate with gut microbiota composition in adulthood. Psychoneuroendocrinology 2015;60:58-74. [Crossref] [PubMed]
- Ait-Belgnaoui A, Payard I, Rolland C, et al. Bifidobacterium longum and Lactobacillus helveticus Synergistically Suppress Stress-related Visceral Hypersensitivity Through Hypothalamic-Pituitary-Adrenal Axis Modulation. J Neurogastroenterol Motil 2018;24:138-46. [Crossref] [PubMed]
- Peng HH, Tsai TC, Huang WY, et al. Probiotic treatment restores normal developmental trajectories of fear memory retention in maternally separated infant rats. Neuropharmacology 2019;153:53-62. [Crossref] [PubMed]
- Tolunay O, Celik T, Komur M, et al. A rare cause of status epilepticus; alpha lipoic acid intoxication, case report and review of the literature. Eur J Paediatr Neurol 2015;19:730-2. [Crossref] [PubMed]
- Sudo N, Chida Y, Aiba Y, et al. Postnatal microbial colonization programs the hypothalamic-pituitary-adrenal system for stress response in mice. J Physiol 2004;558:263-75. [Crossref] [PubMed]
- Arulsamy A, Tan QY, Balasubramaniam V, et al. Gut Microbiota and Epilepsy: A Systematic Review on Their Relationship and Possible Therapeutics. ACS Chem Neurosci 2020;11:3488-98. [Crossref] [PubMed]
- Veech RL. The therapeutic implications of ketone bodies: the effects of ketone bodies in pathological conditions: ketosis, ketogenic diet, redox states, insulin resistance, and mitochondrial metabolism. Prostaglandins Leukot Essent Fatty Acids 2004;70:309-19. [Crossref] [PubMed]
- Zhang Y, Zhou S, Zhou Y, et al. Altered gut microbiome composition in children with refractory epilepsy after ketogenic diet. Epilepsy Res 2018;145:163-8. [Crossref] [PubMed]
- Olson CA, Vuong HE, Yano JM, et al. The Gut Microbiota Mediates the Anti-Seizure Effects of the Ketogenic Diet. Cell 2018;173:1728-41.e13. [Crossref] [PubMed]
- Gomez-Eguilaz M, Ramon-Trapero JL, Perez-Martinez L, et al. The beneficial effect of probiotics as a supplementary treatment in drug-resistant epilepsy: a pilot study. Benef Microbes 2018;9:875-81. [Crossref] [PubMed]