Anesthetic management of thoracoscopic procedures in neonates: a retrospective analysis of 45 cases
Introduction
Advances in minimally invasive surgery (MIS), primarily miniaturization of equipment, have made neonatal thoracoscopic surgery now not only possible, but increasingly utilized. Neonates with a variety of conditions, including congenital esophageal atresia/tracheoesophageal fistula (EA/TEF) and congenital diaphragmatic hernia (CDH), are currently being managed thoracoscopically with good results (1-4). MIS has the advantage of causing less trauma, resulting in less postoperative pain, faster recovery, shorter hospital stay, and lower incidences of scoliosis caused by thoracic and papillary asymmetry and rib fusion (5-8). MIS in neonates, however, is incredibly challenging primarily due to the patient’s small size and immature physiological systems (particularly the lungs). The principal physiological impasse in thoracoscopic surgery results from CO2 insufflation which limits lung excursion and decreases lung compliance. In addition to the risks of hypoxemia and hypercarbia, venous return to the heart is often compromised resulting in a reduction in cardiac output. Thoracoscopic surgery in neonates poses many challenges to anesthesiologists. The anesthetic management of thoracoscopic procedures in neonates should avoid excess volume of mask oxygen. When hyperventilation may lead to the V/Q ratio imbalance and then aggravate the ventilation barrier. In addition, the inhaled anesthetics which are used should not inhibit pulmonary vasoconstriction. Furthermore, the high volume automatic ventilation used during surgery can cause damage to the lungs. Thus, it should be adjusted to manual ventilation. Finally, inhaled CO2 gas needs to be humidified. Otherwise, a large amount of unhumidified CO2 gas can cause hypothermia. This study retrospectively analyzed 45 cases of thoracoscopic surgery in neonates performed at the Hubei Women & Children’s Hospital from December 2015 to November 2019. Our experience in managing these procedures is summarized.
We present the following article in accordance with the STROBE reporting checklist (available at https://dx.doi.org/10.21037/tp-21-265).
Methods
Subjects
All neonates who underwent thoracoscopic surgery in our institution from December 2015 to June 2020 were enrolled in this study. The patients were aged from 4 hours to 7 days old. The patient selection criteria for the thoracoscopic procedures were as follows: (I) hemodynamically stability; (II) adequate and stable mechanical ventilation if already intubated; (III) no co-existing cyanotic congenital heart disease or other severe congenital malformations; (IV) no serious metabolic disturbances; and (V) bodyweight of 2,000 grams or more (it is technically very difficult to perform thoracoscopic procedures in patients less than 2,000 grams).
Preoperative preparation
All patients were NPO (nil by mouth), had gastrointestinal or esophageal decompression, and had received intravenous fluid replacement and correction of electrolyte imbalances. Patients were supplemented with oxygen to maintain an oxygen saturation (SpO2) of 85% or higher and patients were actively warmed as necessary to maintain normothermia. Neonates demonstrating impending respiratory failure (unable to maintain SpO2 85% or higher with non-invasive oxygen supplement) were preemptively intubated and mechanically ventilated.
Anesthesia technique
Intraoperative monitoring included electrocardiogram (ECG), heart rate (HR), invasive blood pressure [intravenous line and arterial lines were often inserted before patients was taken to the operating room (OR)], pulse oximetry (SpO2), urine output, and body temperature. All respiratory parameters, including ETCO2 (end-tidal CO2) and airway pressures were recorded. During pre-oxygenation with 100% O2, penehyclidine (0.01 mg/kg, an anticholinergic agent) and dexamethasone (1 mg/kg) were administered intravenously. An inhalational induction was performed with sevoflurane (6–8%) and endotracheal intubation was performed under direct laryngoscopy with a 3.0 or 3.5 endotracheal tube (ETT). If the neonate arrived to the OR intubated, induction was performed with sevoflurane via the ETT. Auscultation of breath sounds was used to adjust the position of the ETT above the level of the carina. Maintenance of anesthesia was achieved with sevoflurane (0.5–1.0 MAC) and an intravenous infusion of remifentanil (0.2–0.3 mg/kg/min) using a computerized micro-pump. One-lung ventilation was achieved with a technique that involved advancing the ETT into the non-operative main bronchus (blindly but with confirmation by breath sounds) and insufflating the operative hemithorax with CO2 (to a pressure of 4–6 mmHg). This provided the surgical team with a workable exposure of the operative field. Pressure-controlled ventilation (PCV) was used during surgery with peak pressures maintained at 15–25 cmH2O. Respiratory frequency was set at 35–55 times/minute, inhaled oxygen concentration at 60–80%, and inspiratory/expiratory ratio (I:E ratio) was maintained at 1:1–1.5. Dopamine or dobutamine was administered as necessary to maintain hemodynamic stability.
Postoperative management
All patients were taken to the neonatal intensive care unit (NICU) intubated after surgery to avoid respiratory muscle fatigue and respiratory failure. They were weaned off ventilatory support and extubated when extubation parameters were met. Intravascular volume status was closely monitored to maintain normovolemia and hemodynamic stability.
Data acquisition
Arterial blood gas (ABG) analysis was performed at the time of arrival at the OR (T
Statistical analysis
Data analysis was performed using SPSS21 software (IBM, Armonk, New York, USA). Data sets are presented as mean ± standard deviation (SD), and repeated measurements of variance analysis were used for statistical processing. P<0.05 was considered statistically significant.
Ethical statement
This retrospective study was approved by the Institutional Review Board of Hubei Women & Children’s Hospital. The study was conducted in accordance with the Declaration of Helsinki (as revised in 2013). All patients gave their informed consent.
Results
A total of 45 neonates were enrolled in this study, including 28 (62.2%) males and 17 (37.8%) females. The ages ranged from 4 hours to 7 days with gestational ages at birth between 33.0 and 40.0 weeks. Mean birth weight was 2,922±432.6 grams (range, 2,000–3,700 grams). CDH accounted for 55.6% (25/45) and EA/TEF accounted for 44.4% (20/45) of all cases. All patients survived the surgery. Vital signs, ABG, and respiratory parameters at each time point are shown in Figures 1 and 2. Operative times ranged from 70 to 445 minutes (mean 233.3± 112.4 minutes). After surgery, all patients were returned to the NICU intubated and mechanically ventilated.
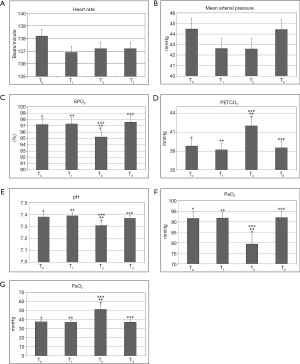
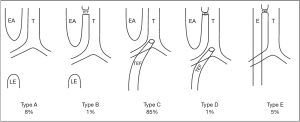
As shown in Tables 1 and 2, our surgical team developed the following technique to establish an artificial pneumothorax for thoracoscopic surgery. The insufflation pressure is increased slowly to 4–6 mmHg with the CO2 flow rate controlled at 1–2 L/minute. This allows the neonate more time to adapt to the gradual CO2 insufflation-induced pneumothorax, reducing the impact on respiratory and circulatory function. In our experience, this technique was effective at minimizing the adverse effects of pneumothorax on the respiratory and circulatory systems. Out of the 45 neonates, 14 patients experienced episodes of intraoperative hypoxemia (SPO2 <90%), an incidence of 31.1%. One patient entered the OR with a SpO2 of 87% and developed hypoxemia intraoperatively about 2 hours after the commencement of the operation, with the lowest SpO2 reading at 74%. Immediately after surgery, the SpO2 recovered to 82%. Most episodes of hypoxemia responded to prompt intervention and were of short duration.
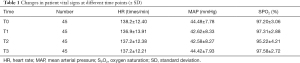
Full table
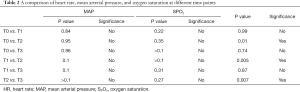
Full table
The data and comparison of end-expiratory carbon dioxide partial pressure, pH, arterial oxygen partial pressure, and carbon dioxide partial pressure at different time points were showed in Tables 3 and 4. Hypercapnia, based on ABG 30 minutes after CO2 insufflation/pneumothorax establishment, was detected in 25 cases (55.6%). Mild hypothermia occurred in one patient (lowest body temperature was 35.3 °C in a 2 kg neonate). One patient developed an obstructed ETT which occurred twice during surgery. After repeated suctioning and no improvement in ventilation, the ETT was replaced and ventilation improved. The procedure in this case was very long due to surgical technical difficulties which necessitated the conversion from thoracoscopy to open thoracotomy. One of the 45 neonates developed persistent pulmonary hypertension postoperatively. The family requested no further treatment and the patient expired. Postoperatively, one patient developed a pulmonary infection and was transferred to a cardiac hospital due to a co-existing congenital cardiac malformation. Three patients were transferred to their local hospitals for further care after initial recovery. One patient developed a pulmonary hemorrhage, right-side pneumothorax, and left lung atelectasis one day after surgery. The family of this patient requested no further treatment and the patient expired. The remaining 40 cases fully recovered and were discharged, and all returned for follow-up visits.
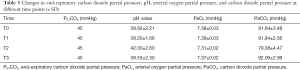
Full table
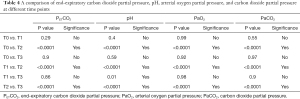
Full table
Discussion
At present, there are no widely accepted criteria to guide the suitability of neonates for thoracoscopic surgery. Minimally invasive surgery may lead to incomplete treatment of the disease and may cause repeated attacks in the later stage. A study by Becmeur et al. indicated that respiratory distress and pulmonary hypertension caused by severe pulmonary dysplasia were considered contraindications for thoracoscopy in patients with CDH (9,10). CDH is a syndrome that includes pulmonary hypoplasia, lung immaturity, left heart hypoplasia, and persistent pulmonary hypertension of the newborn (11). CDH is often a physiological emergency rather than a surgical emergency. There are 5 subtypes of EA/TEF as illustrated in Figure 2, with Type III being the most common. Many patients with EA/TEF also suffer from aspiration or aspiration pneumonia as well as electrolyte disturbances. Detailed preoperative preparation includes fasting and gastrointestinal decompression, maintaining normothermia, infection prevention, oxygen supplementation, assisted ventilation when necessary, hemodynamic augmentation, and application of pulmonary surfactant as indicated. These patients should be closely monitored and assessed to provide the best preoperative conditions possible (12).
Neonates are prone to develop atelectasis and hypoxemia because of their low body weight, small tidal volumes, low functional residual capacity, and high closing volumes. Many neonates with CDH and EA/TEF also exhibit some degree of pulmonary dysplasia and pulmonary soiling. CO2 insufflation during surgery collapses the lung on the affected side which further compromises pulmonary function contributing to the hypercapnia and hypoxemia frequently encountered during thoracoscopic surgery (13,14). The main ventilation strategies for neonatal thoracoscopic surgery are the use of PCV, maintaining peak airway pressure at 15–25 cmH2O with a respiratory rate of 35–55 times/minute, a fraction of inspired oxygen (FiO2) of 60–80%, I:E ratio of 1:1–1.5, and intermittent airway suctioning to clear secretions.
At lower peak and mean airway pressure, PCV has been shown to provide more effective ventilation compared to volume-controlled ventilation (VCV), and that this may have a protective effect on the lungs (15). Tuğrul et al. reported that intrapulmonary shunt is higher using VCV than with PCV. The mechanism for this may be that the decelerating inspiration observed with the PCV mode reduces airway pressure allowing volatile anesthetic agents to be distributed more evenly. The reduction in peak airway pressure in the PCV mode may also decrease the risk of barotrauma. It has also been shown that PCV is associated with better arterial oxygenation than VCV during one-lung ventilation (16). However, because PCV cannot guarantee a constant tidal volume (due to changes in compliance), anesthesiologists need to closely monitor for changes in minute ventilation. Peak airway pressure should be maintained at the lowest possible levels to minimize the incidence of barotrauma. In 1995, Wung et al. proposed that the ideal airway pressure should not exceed 25 cmH2O (17). In our patients, peak airway pressure was set at 15–25 cmH2O. FiO2 was adjusted according to oxygenation status. The literature provides no consensus as to the ideal FiO2 in these neonates during induction and maintenance of general anesthesia. Some studies have indicated that neonates should not receive 100% oxygen to avoid oxidative damage. However, a low FiO2 can also be problematic because it may lead to hypoxemia (18). We maintained oxygen saturations at less than or equal to 96% in an attempt to prevent both hyper- and hypoxemia. To accomplish this, the FiO2 during thoracoscopy was maintained at 60–80% which was adjusted as needed according to the ventilation and oxygenation status. The respiratory frequency was adjusted based on PETCO2 and PaCO2, usually set at 30–35 breathes/minute. The I:E ratio was set at 1:1–1.5 to allow time for adequate exhalation. If hypoxemia and/or hypercapnia was encountered, mechanical obstruction of the ETT must first be ruled out. Frequent airway suction can correct this problem. Intermittent lung expansion may also be required. If ventilation and oxygenation status does not improve with these maneuvers, bilateral lung ventilation should be resumed until the etiology of hypoxemia is identified and corrected. Strategies to prevent and treat hypoxemia included airway suctioning to remove secretions, adjustment of respiratory parameters such as changing the I:E ratio, increasing FiO2, applying positive end-expiratory pressure (PEEP), alveolar recruitment with a Valsalva maneuver, and bronchodilator therapy. The pressure of artificial pneumothorax should be kept at the lowest level possible. We maintained a pressure of 4–6 mmHg in our patients. Continuous infusion of CO2 to establish artificial pneumothorax increases the intrathoracic pressure and may cause symptoms similar to tension pneumothorax. Convertino et al. reported that hemodynamic stability was maintained when the intrathoracic pressure was less than 10 mmHg during thoracoscopic surgery (18). Intraoperative hypercarbia was largely due to CO2 insufflation and was treated by hyperventilation. Some studies have reported that infants who undergo thoracoscopic surgery are more likely to develop hypercapnia (19). Sevoflurane (0.5–1.0 MAC) and an intravenous infusion of remifentanil (0.2–0.3 mg /kg/min) were used to maintain general anesthesia. These were adjusted based on hemodynamic parameters. Preoperative fasting in neonates may lead to various degrees of water and electrolyte imbalance and hypovolemia. A small amount of bleeding during surgery may present as a decrease in blood pressure and usually responds well to small fluid boluses (20). Excessive intravenous infusion may lead to increased shunts and potentially, pulmonary edema. In our cohort, dopamine (3–6 mg /kg/min) or dobutamine (3–6 mg/kg/min) was administered as needed to maintain hemodynamic stability only after intravascular volume was determined to be optimized. The temperature regulating mechanisms in neonates are immature. Low ambient temperatures in the OR, blood loss during surgery, cold fluids (both irrigation and intravenous), and continuous insufflation of a large amount of cold CO2 without humidification into the thoracic cavity can all contribute to a decrease in body temperature intraoperatively. Hypothermia increases the incidence of surgical complications and increases oxygen consumption (21). Pre-warming the OR, using forced-air warming devices, a radiant warmer, and fluid warmers are all important in the prevention of hypothermia. Preoperative pulmonary function should be improved. Postoperative anti-infection and respiratory support therapy should be strengthened. Furthermore, it is necessary to adjust the oxygen concentration in the operation in time according to the vital signs of the children to ensure the smooth operation. Continuous monitoring of the child’s temperature is mandatory. Normothermia was maintained in all our patients throughout the perioperative period.
Conclusions
Many factors determine the success of thoracoscopic procedures in neonates. We have described our experience with 45 neonates including the selection criteria, preoperative optimization including thorough patient evaluation, correction of fluid and electrolytes disturbances, and formatting a detailed plan for both the anesthesia and surgery teams. Intraoperative strategies include precise airway and respiratory management, use of a “low pressure-slow insufflation technique” to create an artificial pneumothorax, the use of PCV with peak airway pressure maintained at 20–25 mmHg, close hemodynamic monitoring, and maintenance of normovolemia and normothermia. Postoperative management should include admission of all patients to the NICU, intubated and mechanically ventilated, with careful monitoring and management to allow for early intervention to treat any hemodynamic, respiratory, or surgical complications.
Acknowledgments
Funding: None.
Footnote
Reporting Checklist: The authors have completed the STROBE reporting checklist. Available at https://dx.doi.org/10.21037/tp-21-265
Data Sharing Statement: Available at https://dx.doi.org/10.21037/tp-21-265
Conflicts of Interest: All authors have completed the ICMJE uniform disclosure form (available at https://dx.doi.org/10.21037/tp-21-265). The authors have no conflicts of interest to declare.
Ethical Statement: The authors are accountable for all aspects of the work in ensuring that questions related to the accuracy or integrity of any part of the work are appropriately investigated and resolved. This retrospective study was approved by the Institutional Review Board of Hubei Women & Children’s Hospital. The study was conducted in accordance with the Declaration of Helsinki (as revised in 2013). The patients gave their informed consent.
Open Access Statement: This is an Open Access article distributed in accordance with the Creative Commons Attribution-NonCommercial-NoDerivs 4.0 International License (CC BY-NC-ND 4.0), which permits the non-commercial replication and distribution of the article with the strict proviso that no changes or edits are made and the original work is properly cited (including links to both the formal publication through the relevant DOI and the license). See: https://creativecommons.org/licenses/by-nc-nd/4.0/.
References
- Albanese CT, Rothenberg SS. Experience with 144 consecutive pediatric thoracoscopic lobectomies. J Laparoendosc Adv Surg Tech A 2007;17:339-41. [Crossref] [PubMed]
- Cano I, Anton-Pacheco JL, Garcia A, et al. Video-assisted thoracoscopic lobectomy in infants. Eur J Cardiothorac Surg 2006;29:997-1000. [Crossref] [PubMed]
- Chou HC, Hsu WM. New evolutions in congenital diaphragmatic hernia. Pediatr Neonatol 2010;51:80-2. [Crossref] [PubMed]
- Rothenberg SS. First decade's experience with thoracoscopic lobectomy in infants and children. J Pediatr Surg 2008;43:40-4; discussion 45. [Crossref] [PubMed]
- Byon HJ, Lee JW, Kim JK, et al. Anesthetic management of video-assisted thoracoscopic surgery (VATS) in pediatric patients: the issue of safety in infant and younger children. Korean J Anesthesiol 2010;59:99-103. [Crossref] [PubMed]
- Lao OB, Crouthamel MR, Goldin AB, et al. Thoracoscopic repair of congenital diaphragmatic hernia in infancy. J Laparoendosc Adv Surg Tech A 2010;20:271-6. [Crossref] [PubMed]
- Szavay PO, Obermayr F, Maas C, et al. Perioperative outcome of patients with congenital diaphragmatic hernia undergoing open versus minimally invasive surgery. J Laparoendosc Adv Surg Tech A 2012;22:285-9. [Crossref] [PubMed]
- Vijfhuize S, Deden AC, Costerus SA, et al. Minimal access surgery for repair of congenital diaphragmatic hernia: is it advantageous?--An open review. Eur J Pediatr Surg 2012;22:364-73. [Crossref] [PubMed]
- Becmeur F, Reinberg O, Dimitriu C, et al. Thoracoscopic repair of congenital diaphragmatic hernia in children. Semin Pediatr Surg 2007;16:238-44. [Crossref] [PubMed]
- Gomes Ferreira C, Reinberg O, Becmeur F, et al. Neonatal minimally invasive surgery for congenital diaphragmatic hernias: a multicenter study using thoracoscopy or laparoscopy. Surg Endosc 2009;23:1650-9. [Crossref] [PubMed]
- Hua K, Yang S, Zhang Y, et al. Thoracoscopic surgery for recurrent tracheoesophageal fistula after esophageal atresia repair. Dis Esophagus 2020;33:doaa023.
- Snoek KG, Reiss IK, Greenough A, et al. Standardized Postnatal Management of Infants with Congenital Diaphragmatic Hernia in Europe: The CDH EURO Consortium Consensus - 2015 Update. Neonatology 2016;110:66-74. [Crossref] [PubMed]
- Fishman JR, Blackburn SC, Jones NJ, et al. Does thoracoscopic congenital diaphragmatic hernia repair cause a significant intraoperative acidosis when compared to an open abdominal approach? J Pediatr Surg 2011;46:458-61. [Crossref] [PubMed]
- Li H, Xia H, Zhang X, et al. Risk factors for contralateral recurrence of primary spontaneous pneumothorax after thoracoscopic surgery. Journal of Harbin Medical University 2016;50:134-8.
- Unzueta MC, Casas JI, Moral MV. Pressure-controlled versus volume-controlled ventilation during one-lung ventilation for thoracic surgery. Anesth Analg 2007;104:1029-33. tables of contents. [Crossref] [PubMed]
- Tuğrul M, Camci E, Karadeniz H, et al. Comparison of volume controlled with pressure controlled ventilation during one-lung anaesthesia. Br J Anaesth 1997;79:306-10. [Crossref] [PubMed]
- Wung JT, Sahni R, Moffitt ST, et al. Congenital diaphragmatic hernia: survival treated with very delayed surgery, spontaneous respiration, and no chest tube. J Pediatr Surg 1995;30:406-9. [Crossref] [PubMed]
- Yokoi A, Nishijima E. Long-term complications of esophageal atresia. Nihon Geka Gakkai Zasshi 2009;110:179-83. [PubMed]
- Convertino VA, Parquette BA, Wampler DA, et al. Use of intrathoracic pressure regulation therapy in breathing patients for the treatment of hypotension secondary to trauma. Scand J Trauma Resusc Emerg Med 2017;25:105. [Crossref] [PubMed]
- Breschan C, Likar R. Anesthetic management of surgery in term and preterm infants. Anaesthesist 2006;55:1087-98. [Crossref] [PubMed]
- van Hoorn CE, Costerus SA, Lau J, et al. Perioperative management of esophageal atresia/tracheo-esophageal fistula: An analysis of data of 101 consecutive patients. Paediatr Anaesth 2019;29:1024-32. [Crossref] [PubMed]
(English Language Editor: J. Teoh)