Editor’s note:
“Rare Diseases Column” is chaired by Dr. Zhanhe Wu from The Children’s Hospital at Westmead, Australia, featuring articles related to rare diseases mostly genetic based, presented in early life disease, with chronic phase but frequently progressive, disabling and life threatening diseases. Article types of original articles, review articles, case reports, perspectives, etc. are welcomed to be submitted to the column.
Phenylketonuria: a review of current and future treatments
Introduction
Phenylketonuria (PKU; OMIM 261600) is an inborn error of metabolism caused predominantly by mutations in the phenylalanine hydroxylase (PAH) gene (1). Mutations in the PAH gene result in decreased catalytic activity affecting the catabolic pathway of phenylalanine (Phe) (Figure 1). PAH is a hepatic enzyme that requires the cofactor tetrahydrobiopterin (BH4) to convert Phe to tyrosine (Tyr) (Figure 1). A deficiency in PAH or its cofactor BH4, results in the accumulation of excess phenylalanine, whose toxic effects can cause severe and irreversible intellectual disability if untreated (1). Other clinical features associated with untreated PKU may include autistic behaviours, motor deficits, eczematous rash and seizures. Behavioural impairment as well as psychiatric disturbances can become apparent with age (3).
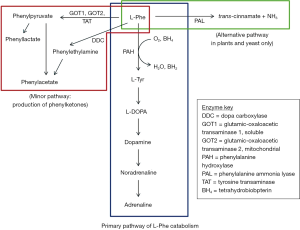
Diagnosis is initially undertaken through newborn screening programs in the first weeks of life and all cases are further screened for BH4 responsiveness (Packman, 2003). PKU has been described in all ethnic groups and its incidence varies widely around the world, affecting of one in every 10,000 births in Caucasians (4), and with the highest incidence being in Northern Europe. Finland has the lowest incidence in Europe with one case in every 100,000 live births, while Turkey has the highest incidence with one in every 4000 births due to high consanguinity within the population (5). In Australia, approximately 25 babies are diagnosed with PKU each year (based on the recorded incidence of new cases).
Currently, there is no cure for PKU, however, the prevailing treatment is predominantly through dietary restriction of Phe to the minimum required for normal growth, supplemented with specifically designed medical foods. The establishment of newborn screening programs along with the prompt institution of dietary treatment has prevented intellectual disability, however, neurophysiological and neuropsychological impairments may still persist in treated PKU patients (6-10). Furthermore, there are often problems associated with dietary therapy, including nutritional deficiencies (11-13) as well as non-compliance due to poor palatability.
More advanced medical foods and formulas and improved palatability, including large neutral amino acids (LNAA) and glycomacropeptides (GMP), have been explored (14-16), however, long term studies are still needed to understand the full impact of these treatments. BH4 therapy has been successful in BH4 responsive patients; however, only about 30% of all PKU patients can benefit from this treatment (17). Enzyme replacement therapy through viral delivery of protein fusions targeting the liver has been promising in recent years (18,19) as well as enzyme substitution therapy where phenylalanine ammonia lyase is used as a substitute to PAH. In this review we aim to summarise recent literature on current treatments and the concerns associated with them, as well as exploring novel approaches, their limitations and possible future therapies.
Current treatments
Dietary therapy
Dietary restriction of phenylalanine remains to be the mainstay of treatment for PKU since its introduction in 1953 by Bickel and colleagues (20). To prevent any irreversible neurological damage that results from excess blood and consequently brain Phe in PKU patients, dietary treatment must commence in the neonatal period and adhered to for life. Patients with PKU must strictly limit their intake of foods rich in protein, such as meats, fish, eggs and dairy products. Low-protein high-starch natural foods such as potatoes, some vegetables (such as peas) can be eaten but only in restricted amounts. Due to the severe restriction of protein intake, PKU patients must be supplemented with medical food substitutes containing the right mix of essential amino acids, vitamins, minerals and trace nutrients (21). Normally, about 90% of the dietary phenylalanine intake is converted into tyrosine; therefore a crucial part of the treatment is tyrosine supplementation (22).
Controversy regarding the continuation of the dietary therapy after childhood was ongoing (23-26), as many practitioners in the early 1970s believed that elevated Phe has no detrimental effect once the brain is fully developed (27). However, a US-based National Collaborative Study for PKU showed that discontinuation of dietary control correlated with a decline in school performance in children and an increase in behavioural and psychosocial problems in adults (28). Furthermore, females with PKU are at high risk of having a child with the so-called maternal PKU syndrome, causing microcephaly, intra-uterine growth restriction, congenital heart defects, a characteristic facial appearance and cognitive impairment in the affected infant (29,30). As a result of these studies, the recommendation was to continue life-long diet therapy.
Problems associated with dietary therapy
While there is no cure for PKU, the current dietary restriction of Phe has been very successful in curtailing intellectual disability and achieving near normal IQ. However, there still remain four major issues with current dietary treatment; (I) dietary compliance due to unpalatability of the diet; (II) persisting neurological or psychosocial issues and poor quality of life despite early intervention; (III) potential nutritional deficiencies resulting from restrictive diet; (IV) financial burden due to the cost of special medical food and dietary supplements.
Adherence to the diet is usually straightforward in the neonatal period and during early childhood, as the child’s parents control the diet. However, dietary compliance becomes increasingly difficult as children approach adolescence due to palatability of the diet. This is clearly reflected by poor control of blood phenylalanine concentrations in a proportion of individuals in this age group (31-33).
Adolescent and adult patients, who have failed to adhere to dietary therapy, although intellectually normal, have been shown to have an increased incidence of anxiety and depression, and there is also evidence of difficulty in forming stable social relationships (34,35). Furthermore, an increased incidence of attention deficit-hyperactivity disorder has been reported in this group (36). Problems in executive functioning at various ages have been identified through neuropsychometric testing (8,9,37-41). Poor concentration, headaches, and sleep disturbances have also been reported (34,40), all of which support previous findings from The National PKU collaborative studies (28,42,43).
Early treated PKU patients with well-controlled Phe levels have normal development and were thought to have a generally normal IQ. However, recent neuropsychological studies indicate that despite strict adherence, early treated PKU patients generally have a lower mean IQ than their unaffected siblings or the normal population (44). Treated PKU patients may also experience suboptimal outcomes in abstract reasoning and problem solving (35). Recent studies showed that the degree of psychological and psychosocial impairments, as well as executive and cognitive functioning, is correlated in adult PKU patients with the life-long variability of Phe levels (39,45,46).
Due to the stringent dietary therapy, nutritional deficiencies are common in PKU patients especially those who do not fully consume the prescribed medical food substitutes. PKU Patients are also likely to be at risk of deficiencies in vitamin B12, vitamin D, calcium (12,47,48), iron (13), and unsaturated long chain fatty acids (10). Such deficiencies may exacerbate the neurological problems and result in reduced bone density in PKU patients (49,50).
The high cost of special medical foods and formula, as well as frequent visits to health professionals, pose a substantial financial burden on PKU patients and their carers (51-54). The main components of PKU treatment cost are the special formula foods and amino acid supplements (52), and depending on the age of the patient it has been estimated to cost £4,000 per year in the UK (52). Furthermore, effective dietary compliance may be hindered by lack of health insurance or non-coverage of certain expenses by insurance companies in countries without a nationalised health system (55). In spite of the success of dietary therapy for the treatment and management of PKU patients, there is mounting evidence regarding issues with dietary compliance and nutritional deficiencies. All of these findings suggest the need to develop novel interventions that may improve the outcome for patients with PKU (4,22,56,57).
Tetrahydrobiopterin (BH4) therapy
In the 1970s it became apparent that there was a subset of patients with hyperphenylalaninaemia who developed neurological complications despite prompt adherence to dietary therapy (58). This subgroup of patients (known to have atypical PKU) turned out to have mutations that cause defects in BH4 synthesis or recycling (59). Synthetic biopterin compounds were made available in the late 70s (60) and the benefit of administering this preparation in patients with atypical PKU was first demonstrated by Schaub et al. (1978) (61). These patients also require neurotransmitter precursors (L-dopa/carbidopa and 5-hydroxytryptophan) as part of their treatment, and for dihydropteridine reductase deficiency, folinic acid supplementation, reviewed in (62).
The distinction between PKU due to PAH deficiency and BH4 deficiency is clarified through the performance of a BH4 loading test, and the quantitation of the neurotransmitters (dopamine, noradrenaline and adrenaline) and their metabolites and pterins in urine and/or CSF (63).
The BH4 loading test is considered positive when initial plasma Phe concentrations decrease by at least 30% after 8 h (64) or by 50% after 24 h. Using the above protocol for the oral loading test, 60-70% of patients with mild PKU responded significantly (65). Using an extended protocol of more than 24 hrs with repeated administration of 10 mg BH4/kg/day, enabled the detection of additional mild or moderate PKU patients who are considered to be slow responders (66).
In recent years, an additional synthetic analogue to BH4, sapropterin, has been developed, which can also be used to treat this subset of patients. The BH4 loading test was first used to differentiate between patients with elevated Phe levels either due to PAH deficiency or BH4 deficiency. Kure and colleagues demonstrated for the first time that a subset of PAH-deficient PKU patients were BH4 responsive (67), however this response is noted predominantly in mild to moderate cases of PKU (68-71). The responsiveness to BH4 therapy is likely to be associated with mutations in the PAH gene resulting in some residual enzyme activity, however genotype-phenotype correlations are inconsistent (65). Treatment with the cofactor BH4 or sapropterin (72) in BH4 responsive PKU patients has proven successful in significantly increasing Phe tolerance allowing patients to relax their diet and in some cases discontinuing the Phe free diet altogether (73,74). However, for 90% of patients with classical PKU, who comprise about 50-80% of patients detected by newborn screening (PAHdb; http://www.pahdb.mcgill.ca), BH4 therapy has no beneficial effects (75). These patients may therefore benefit from alternative treatments for which efficacy is not dependent on genetic variations in the PAH gene.
Large neutral amino acids (LNAA)
The LNAAs, Phe, tyrosine, tryptophan, and the branched-chain amino acids share the same amino acid transport system across the blood–brain barrier. Therefore, at high concentrations, phenylalanine in the blood will compete with other LNAAs for transport across the blood–brain barrier (76). Large neutral amino acid supplementation has been shown to reduce cerebral Phe concentrations despite the observed increase in plasma Phe levels (76). However, others have shown decreased blood Phe levels in PKU patients with LNAA supplementation (10,77-79) suggesting that LNNAs not only compete with Phe for transport across the blood-brain barrier (76), but may also exert its effect by competing with Phe for active transport across the intestinal mucosa (77,78,80).
Supplementation of tyrosine and tryptophan has shown improved metabolism of dopamine and serotonin in PKU patients, however studies with larger doses of tyrosine and tryptophan did not show positive outcomes (22) LNAA treatment appears to have a beneficial effect on executive functioning (10), however this treatment is really only suitable for adults who are not adhering to a low Phe diet (10,40,81). Furthermore, clinical data regarding long term outcome using this treatment strategy are not yet available (3,10,40,81), requiring additional studies to prove the safety and efficacy of this treatment (82).
Glycomacropeptides (GMP)
GMP is a protein derived from cheese whey that is naturally low in Phe and is rich in valine, isoleucine and threonine (83). GMP, manufactured to sufficient purity and supplemented with the essential amino acids tyrosine, tryptophan, arginine, cysteine and histidine can be a useful adjunct to the Phe restricted diet (14-16). Studies suggest that PKU patients find foods containing GMP more palatable than their usual amino acid formula, preferring a diet supplemented with GMP (14,16,84). The potential benefits of having GMP in the PKU diet have been explored and data showed that the GMP diet significantly reduced ureagenesis, improved protein retention and Phe utilisation (16). Another study found that consuming the GMP diet for breakfast promoted satiety as reflected by decreased levels of the postprandial ghrelin concentration (associated with greater feelings of fullness) when compared to an amino acid diet (85).
It has been shown that PKU mice display an increase in energy expenditure which is consistent with increased metabolic activity seen in PKU mice (15). This increase in metabolic activity is accompanied by an increase in liver mass (86), both of which are caused by the catabolism of excess Phe to phenylketones as well as energy lost from urinary excretion of Phe-derived organic acids (15). Another finding from this study was that mice fed a high Phe (casein) diet or low Phe amino acid diet may exhibit systemic inflammation showing increased plasma concentrations of interferon-gamma (IFN-γ), interleukin-1beta (IL-1β) and granulocyte macrophage colony-stimulating factor (GM-CSF). Interestingly, the GMP diet attenuated these immune responses and decreased metabolic activity in the PKU mice as well as significantly reduced plasma Phe concentrations. The authors conclude that the GMP diet provides a more natural and physiological low Phe source of intact protein as compared with the synthetic amino acid diet (15). These data are promising and could be used as an alternative to the amino acid diet, however, further studies are needed to investigate the effect of the GMP diet on systemic inflammation in human subjects and to further evaluate the safety and efficacy of GMP consumption for long term.
Alternative treatments
Gene therapy
In gene therapy a functional recombinant PAH gene is targeted to the liver, since the activity of PAH is primarily in the liver. Several types of viral vectors, including adenoviral, and adeno-associated viral vectors, have been examined for their potential to correct PKU in mouse models or to correct cultured hepatocytes derived from these mouse models. Fang et al. (1994) infused a recombinant adenoviral vector containing the human PAH-cDNA into the liver through the portal vein of PKU mice. Within one week, complete normalization of the serum phenylalanine levels was achieved in these PKU mice (87). However, the therapeutic effect of the adenoviral vector ceased after a few weeks and repeated administration did not recapitulate the original results due to development of an immune response (87). For this treatment to be effective, adenoviral vectors needed to be further modified in order to eliminate the adenoviral genes responsible for the immune responses (88).
The recombinant adeno-associated virus (rAAV) vectors have gained increasing attention as a safe vector for viral gene transfer. They are non-pathogenic, can transduce non-quiescent cells and have the ability to establish long-term transgene expression in a wide variety of tissues (89). Furthermore, the vectors do not carry any viral genes, and therefore elicit minimal immune responses (89). A study showed that an rAAV carrying the mouse PAH cDNA delivered to the liver by portal vein injection reduced blood phe levels in PKU mice (90). However, this reduction was only noted in male PKU mice. Normalisation of phe levels in PKU mice as well as reversal of hypopigmentation was demonstrated, however three times more vector was needed for female PKU mice to achieve an equivalent reduction in serum phe levels as that seen in male mice (90). Furthermore, correction did not persist beyond 40 weeks with blood phe returning to pretreatment levels (90). These authors suggested that the gender dependent response to treatment may be due to the differences in androgen-dependent pathways, however, this mechanism is not clearly understood (90).
The use of self-complementary AAV vectors resulted in normalisation of hyperphenylalaninaemia for up to 80 weeks in both males and females (19). Although correction in females was achieved, gender specific differences were also apparent using this vector, and therefore larger doses of the vector had to be used for females (19).
The transfer of foreign genes using non-viral vectors into tissues or organs by direct injection of naked plasmid-DNA has been achieved leading to transgene expression and therapeutic responses (91,92). The advantage of using non-viral vectors over viral delivery systems is that there is no size limitation of the DNA insert, and no potential cytopathic side-effects. However, its efficacy is hampered by the very low gene transfer rate and transient transgene expression (92). A recent study addressed some of these issues by using minicircle (MC) naked-DNA vectors devoid of any viral or bacterial sequences (18). This study demonstrated expression of the murine Pah complementary DNA (cDNA) and normalisation of blood Phe levels as well as reversal of hypopigmentation for 1 year in a PKU mouse model (18). The use of MC-DNA may offer improved safety and efficacy as a potential genetic treatment for liver diseases (18). This study provides a step forward with regard to increasing the efficiency and stability of the AAV vectors and use for gene transfer. However, new strategies are required to extend gene transfer efficacy. Furthermore, most vectors do have some genotoxicity at the expense of stability through mitosis (19). The possibility of having gender dependent treatment may lead to having multiple treatment protocols, which would potentially add complexity and confusion. More research is required to create more robust and stable vectors before implementing AAV mediated therapies.
Enzyme therapy
The development of PAH-based fusion proteins to specifically target PAH to the liver has been explored as a novel enzyme replacement therapy (93), where a decrease in plasma Phe levels for several hours after intravenous administration in mice treated with PAH-based fusion proteins (93). It is likely that multiple injections would be required, making this approach less practical from a clinical perspective. Furthermore, the animal model used in this experiment was not an orthologous model to human PKU. In fact, normal C57BL6 mice were utilized in this experiment. The authors concluded that their approach has the potential as an alternative treatment for PKU (93), however such conclusions could really only be reached based on data from mouse models orthologous to human PKU.
Phenylalanine ammonia-lyase (PAL)
Enzyme substitution therapy using PAL (E.C.4.3.1.5) has also been suggested as a possible therapeutic approach for PKU. PAL is an enzyme that catalyses the conversion of Phe to transcinnamic acid and insignificant amounts of ammonia (Figure 1). Unlike the mammalian enzyme (PAH), PAL is a monomer and requires no cofactors (94). It is the alternative metabolic pathway found in higher plants and yeast. In plants, it is mainly involved in defence mechanisms (95), while in micro-organisms, it has a catabolic role, allowing them to utilise L-phenylalanine (L-Phe) as a sole source of carbon and nitrogen (96). PAL is abundant in yeast, especially in the red yeast Basidiomycetes family Rhodotorula (96). The biological half-life of PAL was approximately 21 hours in several mammalian species (including mice) after a single intravenous injection, but diminished significantly upon repeated administration (96).
The first attempt to investigate the use of PAL as a treatment for PKU was over three decades ago (97-99). Early studies using PAL administered in enteric-coated gelatin capsules to PKU patients, showed reductions in Phe levels (99). Injecting PAL from Rhodosporidium toruloides into a PKU mouse model lowered blood Phe levels in treated PKU mice (100). However, following repeated injections of the PAL enzyme, an immune response was elicited resulting in a significant decrease in half-life. In addition, PAL was found to have low activity in gastric secretions due to protease degradation when administered orally (100). To overcome these issues, more efficient forms of PAL have been made by site-directed mutagenesis and chemical modification with polyethylene glycol (PEG). These modifications have led to the specific activity of PAL being maintained, and reduced its immunogenicity, with prolonged plasma half-life in the PKU mouse model (101-106).
The effect of different PEGylation formulations and protocols on long-term in vivo PAL efficacy has been explored (104). The use of a recombinant anabaena variabilis (AV) PEG-PAL formulation showed complete normalisation of plasma phe levels and total suppression of an immune response, as well as reduced brain phe levels in the PKU mouse model. In addition, reversal of PKU-associated hypopigmentation was achieved (104). This PAL formulation has started phase II clinical trials (105). Despite the progress of this PAL formulation into phase III clinical trials (http://investors.bmrn.com/releasedetail.cfm?ReleaseID=769256), pre-clinical studies indicate that weekly injections are required to sustain a significant decrease in the phe levels in the PKU mice for up to one year (104). Moreover, response to PEG-PAL dosing regimens was gender-dependent in the mouse model, similar to results observed in mice undergoing genome-targeted PAH gene therapy (90,107). Hence, a different PEG-PAL dosing regimen is required for female mice (104). In summary, although this therapy achieved phenylalaninaemia and reversal of PKU induced hypopigmentation in mice, repeated injections of this agent would be invasive and burdensome to the patients and their families, and in fact there have been reports of adverse immune reactions (108). Moreover, physiological stress, pain, inconvenience, cost, and risks of infection are associated with repeated injections.
Oral administration is one of the most convenient ways of delivering drugs, as it is less invasive than intravenous or subcutaneous injections. However, in general oral delivery of enzymes have been complicated by the low gastric pH, proteolytic digestion and circulation time in the gastrointestinal tract; resulting in insufficient bioavailability (100). To increase oral bioavailability of enzymes, various strategies have been used, such as encapsulation of the protein (99,109,110), or the use of live microorganisms as delivery systems (100,111,112). An oral form of rAV-PEG-PAL was tested in mice with PKU and results showed that dose response was not gender dependent (105). These data also indicated that administering three doses of 1 IU of rAV-PEG-PAL over a six-hour period significantly lowered plasma Phe as compared with a single dose of 3 IU (105). The authors concluded that the effect of the PEG-PAL used, while effective in lowering the plasma phe levels, is not long lasting and requires the administration of the agent at regular intervals (105). Approaches which use genetically modified (GM) probiotics have gained wide attention, and have already proved successful during clinical trials in the treatment of such disorders as inflammatory bowel disease, especially Crohn’s disease (113,114). Animal research is continuing to examine the safety and efficacy of this approach for the treatment of other disorders (115-117).
Probiotics
Probiotics are defined as “live microorganisms which, when administered in adequate amounts, confer a health benefit on the host” (118). The most common microorganisms used as probiotics belong to two types of lactic acid producing microorganisms, the Bifidobacteria and the lactic acid bacteria (LAB) (119). LAB are present in fermented foods and have been consumed by humans without any obvious adverse effects for thousands of years (120). Several probiotic products (e.g., Yakult™) have been on the market for over two decades, and have an excellent safety record.
There are extensive reports in the literature regarding the beneficial effects of probiotics in certain conditions. For example, the combined probiotic preparation VSL#3 is used in remission maintenance therapy for ulcerative colitis (121). Lactobacillus plantarum decreases pain and flatulence in patients with irritable bowel syndrome (122,123), and Lactobacillus GG significantly improved dermatitis in infants with atopic eczema and cow’s milk allergy (124). Other reported benefits include, cholesterol lowering effects (125); reduction of small bowel bacterial overgrowth in renal failure (126) and as a vehicle for oral vaccine administration (127,128).
Lactococcus lactis (L. lactis) is a Gram-positive LAB bacterium widely used in the food industry for the production of buttermilk and cheese; hence they are generally regarded as safe (GRAS) organisms and it has been proposed for use as a probiotic (129). L. lactis can survive passage through the stomach acid (130,131), however, depending on the strain of Lactococcus, only 10-30% survive in the duodenum (130,131). L. lactis MG1363 and L. fermentum KLD are very sensitive to bile and have a rather low survival in the ileum. In the presence of bile, these bacteria release their intracellular contents making them potential candidates as vectors to deliver metabolic activity to the duodenum (132). Efficient genetic tools have been developed for L. lactis to produce both heterologous and homologous gene expression (133). Advances in genetic engineering of probiotics have made it possible to increase their beneficial effect potentially targeting a wider range of disorders.
Genetically modified (GM) probiotic for the production and delivery of metabolic enzymes
Metabolic deficiencies may cause the accumulation of metabolites to toxic levels, which potentially results in further damage to the body. Studies using GM probiotics expressing metabolic enzymes have been explored. E. coli expressing Klebsiella aerogenes urease when administered to rats with experimental renal failure resulted in significant reduction in plasma urea, uric acid and creatinine (134,135). The co-expression of fusion protein HSP65 and tandem repeats P277 prevented hyperglycemia, improved glucose tolerance and reduced insulitis in non-obese diabetic mice (136). L. Lactis expressing Staphylococcus hyicus lipase significantly improved lipid digestion in pigs with pancreatic insufficiency (116).
PKU is another example of a metabolic disorder that may benefit from the use of probiotic to deliver the PAL enzyme to the intestine. PAL has been expressed in GM organisms (100,111,137-139) and oral delivery of PAL in microorganisms to treat PKU in animal models has already been explored (100,111,137-139). The first study was conducted in 1999 and showed that oral administration of PAL expressed by genetically engineered E. coli to PKU mice resulted in 31% reduction in plasma phe levels after one hour and a further 44% reduction was noted after two hours (100). They also showed that PAL contained within E. coli was resistant to proteolytic inactivation by intestinal enzymes in vitro (100). Although proof of principle had been achieved, there are safety concerns regarding the use of E. coli for delivering enzymes to the gastrointestinal tract. Non pathogenic E. coli, a minor component of the Proteobacteria division, comprises 8% of the normal gut flora (140). However, there is a potential risk that the engineered E. coli could interact with its wild type equivalent and could become pathogenic in humans, or may alter the composition of luminal microbiota, leading to pathological consequences. Furthermore, E. coli preferentially colonises the large intestine, however for most dietary amino acids, including Phe, absorption takes place in the distal region of the small intestine (141).
Liu et al. (2002) (111) sub-cloned and expressed PAL cDNA from parsley into L. Lactis, which exerts its beneficial effects primarily in the small intestine. The PAL expressing L. lactis was orally administered to rats with hyperphenylalaninaemia. Results from this study showed a significant decrease in phenylalanine levels of the treated group as compared to the control on day 9 of the treatment (111). Although a significant reduction in Phe was achieved in treated rats, the animals used for this study did not represent an orthologous model to human PKU. This necessitates further studies to be done on orthologous models of human PKU, such as the mouse Pahenu2 model. These data suggest that GM probiotics may be useful as an alternative therapy for PKU and may ultimately allow PKU patients to relax their diet. However, more studies are needed to evaluate the safety and efficacy of these GM modified probiotics in a short as well as long-term setting.
Summary
The maturation of newborn screening programs along with the prompt institution of a low-Phe diet successfully prevents intellectual disability in early treated PKU patients. Dietary compliance remains to be the main issue as patients approach adolescence and adulthood resulting in poor blood Phe level control and leading to suboptimal outcomes in psychosocial and cognitive assessments. BH4 therapy has been successful, however only a minor proportion of PKU patients benefit from this treatment. Contrasting data has been generated from studies using LNAA as an alternative to dietary therapy and it has been only recommended for adult PKU patients who do not adhere to the diet. The incorporation of GMP into low-Phe diet has improved palatability, variety and convenience of the diet and has resulted in a better control of blood Phe levels providing a more physiological form of amino acid to be consumed by PKU patients. Although studies using GMP as an alternative to the amino acid supplement has been promising, long-term studies are needed to evaluate the safety and efficacy. Studies using gene therapy have been progressing and are still ongoing and the use of the alternative enzyme, PAL (PEGylated form) as an enzyme substitution therapy has reached phase III clinical trials, however the efficiency of the PAL reduced over time and immune reactions were elicited in some patients. The use of probiotics to produce and deliver the PAL enzyme has gained more attention, and studies are ongoing regarding the safety and efficacy of this approach. All these data highlight the need to find alternative therapies for PKU that could be used safely and efficiently in all PKU patients regardless of genotype, phenotype or gender.
Acknowledgements
We would like to thanks the NSW PKU Association, the Rotary Club of Pennant Hills, the Metabolic Dietary Disorders Association, Australian Rotary Health and Commercialisation Australia for their financial support for our PKU research.
Footnote
Conflicts of Interest: The authors have no conflicts of interest to declare.
References
- Scriver CR, Kaufman S. Hyperphenylalaninemia: phenylalanine hydroxylase deficiency. In: Scriver CR, Beaudet AL, Sly SW, et al. editors. The Metabolic and Molecular Bases of Inherited Disease. 8 ed. New York, NY: McGraw-Hill, 2001:1667-724.
- Ho G, Christodoulou J. Phenylketonuria: translating research into novel therapies. Transl Pediatr 2014;3:49-62.
- Blau N, van Spronsen FJ, Levy HL. Phenylketonuria. Lancet 2010;376:1417-27. [PubMed]
- Matalon R, Michals K. Phenylketonuria: screening, treatment and maternal PKU. Clin Biochem 1991;24:337-42. [PubMed]
- Loeber JG. Neonatal screening in Europe; the situation in 2004. J Inherit Metab Dis 2007;30:430-8. [PubMed]
- Channon S, Cassina C, Lee P. Executive functioning, memory, and learning in phenylketonuria. Neuropsychology 2004;18:613-20. [PubMed]
- Channon S, Lee P. Executive functioning and speed of processing in phenylketonuria. Neuropsychology 2005;19:679-86. [PubMed]
- Huijbregts SC, de Sonneville LM, Licht R, et al. Sustained attention and inhibition of cognitive interference in treated phenylketonuria: associations with concurrent and lifetime phenylalanine concentrations. Neuropsychologia 2002;40:7-15. [PubMed]
- Huijbregts SC, de Sonneville LM, van Spronsen FJ, et al. The neuropsychological profile of early and continuously treated phenylketonuria: orienting, vigilance, and maintenance versus manipulation-functions of working memory. Neurosci Biobehav Rev 2002;26:697-712. [PubMed]
- Schindeler S, Ghosh-Jerath S, Thompson S, et al. The effects of large neutral amino acid supplements in PKU: An MRS and neuropsychological study. Mol Genet Metab 2007;91:48-54. [PubMed]
- Acosta PB, Yannicelli S, Singh R, et al. Intake and Blood Levels of Fatty Acids in Treated Patients With Phenylketonuria. J Pediatr Gastroenterol Nutr 2001;33:253-9. [PubMed]
- Acosta PB, Yannicelli S, Singh R, et al. Nutrient intakes and physical growth of children with phenylketonuria undergoing nutrition therapy. J Am Diet Assoc 2003;103:1167-73. [PubMed]
- Acosta PB, Yannicelli S, Singh RH, et al. Iron status of children with phenylketonuria undergoing nutrition therapy assessed by transferrin receptors. Genet Med 2004;6:96-101. [PubMed]
- Ney DM, Gleason ST, van Calcar SC, et al. Nutritional management of PKU with glycomacropeptide from cheese whey. J Inherit Metab Dis 2009;32:32-9. [PubMed]
- Solverson P, Murali SG, Litscher SJ, et al. Low bone strength is a manifestation of phenylketonuria in mice and is attenuated by a glycomacropeptide diet. PLoS One 2012;7:e45165. [PubMed]
- van Calcar SC, MacLeod EL, Gleason ST, et al. Improved nutritional management of phenylketonuria by using a diet containing glycomacropeptide compared with amino acids. Am J Clin Nutr 2009;89:1068-77. [PubMed]
- Burnett JR. Sapropterin dihydrochloride (Kuvan/phenoptin), an orally active synthetic form of BH4 for the treatment of phenylketonuria. IDrugs 2007;10:805-13. [PubMed]
- Viecelli HM, Harbottle RP, Wong SP, et al. Treatment of phenylketonuria using minicircle-based naked-DNA gene transfer to murine liver. Hepatology 2014;60:1035-43. [PubMed]
- Yagi H, Ogura T, Mizukami H, et al. Complete restoration of phenylalanine oxidation in phenylketonuria mouse by a self-complementary adeno-associated virus vector. J Gene Med 2011;13:114-22. [PubMed]
- Bickel H, Gerrard J, Hickmans EM. The influence of phenylalanine intake on the chemistry and behaviour of a phenyl-ketonuric child. Acta Paediatr 1954;43:64-77. [PubMed]
- Acosta PB, Matalon KM. Nutrition management of patients with inherited disorders of aromatic amino acid metabolism. In: Acosta PB, editors. Nutrition Management of Patients with Inherited Metabolic Disorders. Boston: Jones and Bartlett Publishers, 2010:119-74.
- van Spronsen FJ, Enns GM. Future treatment strategies in phenylketonuria. Mol Genet Metab 2010;99 Suppl 1:S90-5. [PubMed]
- Clayton BE, Moncrieff AA, Pampiglione G, et al. Biochemical and EEG studies in phenylketonuric children during phenylalanine tolerance testc. Arch Dis Child 1966;41:267-72. [PubMed]
- Termination of dietary treatment for phenylketonuria. JAMA 1963;186:127.
- Fisch RO, Torres F, Gravem HJ, et al. Twelve years of clinical experience with phenylketonuria: A statistical evaluation of symptoms, growth, mental development, electroencephalographic records, serum phenylalanine levels, and results of dietary management. Neurology 1969;19:659-66. [PubMed]
- Koff E, Kammerer B, Boyle P, et al. Intelligence and phenylketonuria: Effects of diet termination. J Pediatr 1979;94:534-7. [PubMed]
- Blaskovics ME, Nelson TL. Phenylketonuria and its variations. A review of recent developments. Calif Med 1971;115:42-57. [PubMed]
- Williamson M, Dobson JC, Koch R. Collaborative study of children treated for phenylketonuria: study design. Pediatrics 1977;60:815-21. [PubMed]
- Holtzman NA, Kronmal RA, van Doorninck W, et al. Effect of Age at Loss of Dietary Control on Intellectual Performance and Behavior of Children with Phenylketonuria. N Engl J Med 1986;314:593-8. [PubMed]
- Michals K, Azen C, Acosta P, et al. Blood phenylalanine levels and intelligence of 10-year-old children with PKU in the National Collaborative Study. J Am Diet Assoc 1988;88:1226-9. [PubMed]
- Crone MR, van Spronsen FJ, Oudshoorn K, et al. Behavioural factors related to metabolic control in patients with phenylketonuria. J Inherit Metab Dis 2005;28:627-37. [PubMed]
- Walter JH, White FJ. Blood phenylalanine control in adolescents with phenylketonuria. Int J Adolesc Med Health 2004;16:41-5. [PubMed]
- Walter JH, White FJ, Hall SK, et al. How practical are recommendations for dietary control in phenylketonuria? Lancet 2002;360:55-7. [PubMed]
- Brumm VL, Bilder D, Waisbren SE. Psychiatric symptoms and disorders in phenylketonuria. Mol Genet Metab 2010;99:S59-S63. [PubMed]
- Simon E, Schwarz M, Roos J, et al. Evaluation of quality of life and description of the sociodemographic state in adolescent and young adult patients with phenylketonuria (PKU). Health Qual Life Outcomes 2008;6:25. [PubMed]
- Antshel KM. ADHD, learning, and academic performance in phenylketonuria. Mol Genet Metab 2010;99 Suppl 1:S52-8. [PubMed]
- Brumm VL, Azen C, Moats RA, et al. Neuropsychological outcome of subjects participating in the PKU adult collaborative study: a preliminary review. J Inherit Metab Dis 2004;27:549-66. [PubMed]
- Christ SE, Huijbregts SC, de Sonneville LM, et al. Executive function in early-treated phenylketonuria: Profile and underlying mechanisms. Mol Genet Metab 2010;99 Suppl 1:S22-32. [PubMed]
- Hood A, Grange DK, Christ SE, et al. Variability in Phenylalanine Control Predicts IQ and Executive Abilities in Children with Phenylketonuria. Mol Genet Metab 2014;111:445-51. [PubMed]
- Marsden DL, Rohr FJ, Costas KC. Inborn errors of metabolism: Nutritional Management of Phenylketonuria. In: Benjamin C, editors. Encyclopedia of Human Nutrition. Oxford: Elsevier, 2005:22-7.
- Welsh MC, Pennington BF, Ozonoff S, et al. Neuropsychology of early-treated phenylketonuria: specific executive function deficits. Child Dev 1990;61:1697-713. [PubMed]
- Azen CG, Koch R, Friedman EG, et al. INtellectual development in 12-year-old children treated for phenylketonuria. Am J Dis Child 1991;145:35-9. [PubMed]
- Koch R, Burton B, Hoganson G, et al. Phenylketonuria in adulthood: A collaborative study. J Inherit Metab Dis 2002;25:333-46. [PubMed]
- Moyle JJ, Fox AM, Arthur M, et al. Meta-Analysis of Neuropsychological Symptoms of Adolescents and Adults with PKU. Neuropsychol Rev 2007;17:91-101. [PubMed]
- DeRoche K, Welsh M. Twenty-five years of research on neurocognitive outcomes in early-treated phenylketonuria: intelligence and executive function. Dev Neuropsychol 2008;33:474-504. [PubMed]
- ten Hoedt AE, de Sonneville LJ, Francois B, et al. High phenylalanine levels directly affect mood and sustained attention in adults with phenylketonuria: a randomised, double-blind, placebo-controlled, crossover trial. J Inherit Metab Dis 2011;34:165-71. [PubMed]
- MacDonald A, Rocha JC, van Rijn M, et al. Nutrition in phenylketonuria. Mol Genet Metab 2011;104 Suppl:S10-8. [PubMed]
- 8. Robert M, Rocha JC, van Rijn M, et al. Micronutrient status in phenylketonuria. Mol Genet Metab 2013;110 Suppl:S6-17.
- Modan-Moses D, Vered I, Schwartz G, et al. Peak bone mass in patients with phenylketonuria. J Inherit Metab Dis 2007;30:202-8. [PubMed]
- Schwahn B, Mokov E, Scheidhauer K, et al. Decreased trabecular bone mineral density in patients with phenylketonuria measured by peripheral quantitative computed tomography. Acta Paediatr 1998;87:61-3. [PubMed]
- Awiszus D, Unger I. Coping with PKU: results of narrative interviews with parents. Eur J Pediatr 1990;149:S45-51. [PubMed]
- Guest JF, Bai JJ, Taylor RR, et al. Costs and outcomes over 36 years of patients with phenylketonuria who do and do not remain on a phenylalanine-restricted diet. J Intellect Disabil Res 2013;57:567-79. [PubMed]
- Wappner R, Cho S, Kronmal RA, et al. Management of phenylketonuria for optimal outcome: a review of guidelines for phenylketonuria management and a report of surveys of parents, patients, and clinic directors. Pediatrics 1999;104:e68. [PubMed]
- Recommendations on the dietary management of phenylketonuria. Report of Medical Research Council Working Party on Phenylketonuria. Arch Dis Child 1993;68:426-7. [PubMed]
- Bilginsoy C, Waitzman N, Leonard CO, et al. Living with phenylketonuria: Perspectives of patients and their families. J Inherit Metab Dis 2005;28:639-49. [PubMed]
- Burton BK, Bausell H, Katz R, et al. Sapropterin therapy increases stability of blood phenylalanine levels in patients with BH4-responsive phenylketonuria (PKU). Mol Genet Metab 2010;101:110-4. [PubMed]
- Enns GM, Koch R, Brumm V, et al. Suboptimal outcomes in patients with PKU treated early with diet alone: Revisiting the evidence. Mol Genet Metab 2010;101:99-109. [PubMed]
- Rey F, Blandin-Savoja F, Rey J. Atypical phenylketonuria with normal dihydropteridine reductase activity. N Engl J Med 1976;295:1138-9. [PubMed]
- Kaufman S, Berlow S, Summer GK, et al. Hyperphenylalaninemia Due to a Deficiency of Biopterin. N Engl J Med 1978;299:673-9. [PubMed]
- Schircks B, Bieri JH, Viscontini M. Preparation and characterisation of pure 5,6,7,8-tetrahydro-L-neopterine and 5,6,7,8-tetrahydro-D-monapterine (author's transl). Helv Chim Acta 1976;59:248-52. [PubMed]
- Schaub J, Däumling S, Curtius HC, et al. Tetrahydrobiopterin therapy of atypical phenylketonuria due to defective dihydrobiopterin biosynthesis. Arch Dis Child 1978;53:674-6. [PubMed]
- Blau N, Hennermann JB, Langenbeck U, et al. Diagnosis, classification, and genetics of phenylketonuria and tetrahydrobiopterin (BH4) deficiencies. Mol Genet Metab 2011;104 Suppl:S2-9. [PubMed]
- Longo N. Disorders of biopterin metabolism. J Inherit Metab Dis 2009;32:333-42. [PubMed]
- Bernegger C, Blau N. High frequency of tetrahydrobiopterin-responsiveness among hyperphenylalaninemias: a study of 1919 patients observed from 1988 to 2002. Mol Genet Metab 2002;77:304-13. [PubMed]
- Blau N, Erlandsen H. The metabolic and molecular bases of tetrahydrobiopterin-responsive phenylalanine hydroxylase deficiency. Mol Genet Metab 2004;82:101-11. [PubMed]
- Shintaku H, Kure S, Ohura T, et al. Long-term treatment and diagnosis of tetrahydrobiopterin-responsive hyperphenylalaninemia with a mutant phenylalanine hydroxylase gene. Pediatr Res 2004;55:425-30. [PubMed]
- Kure S, Hou DC, Ohura T, et al. Tetrahydrobiopterin-responsive phenylalanine hydroxylase deficiency. J Pediatr 1999;135:375-8. [PubMed]
- Burton BK, Grange DK, Milanowski A, et al. The response of patients with phenylketonuria and elevated serum phenylalanine to treatment with oral sapropterin dihydrochloride (6R-tetrahydrobiopterin): a phase II, multicentre, open-label, screening study. J Inherit Metab Dis 2007;30:700-7. [PubMed]
- Fiege B, Blau N. Assessment of tetrahydrobiopterin (rBH4) esponsiveness in phenylketonuria. J Pediatr 2007;150:627-30. [PubMed]
- Muntau AC, Röschinger W, Habich M, et al. Tetrahydrobiopterin as an Alternative Treatment for Mild Phenylketonuria. N Engl J Med 2002;347:2122-32. [PubMed]
- Trefz FK, Scheible D, Frauendienst-Egger G, et al. Long-term treatment of patients with mild and classical phenylketonuria by tetrahydrobiopterin. Mol Genet Metab 2005;86 Suppl 1:S75-80. [PubMed]
- Sanford M, Keating GM. Sapropterin: a review of its use in the treatment of primary hyperphenylalaninaemia. Drugs 2009;69:461-76. [PubMed]
- Lambruschini N, Pérez-Dueñas B, Vilaseca MA, et al. Clinical and nutritional evaluation of phenylketonuric patients on tetrahydrobiopterin monotherapy. Mol Genet Metab 2005;86 Suppl 1:S54-60. [PubMed]
- Trefz FK, Burton BK, Longo N, et al. Efficacy of sapropterin dihydrochloride in increasing phenylalanine tolerance in children with phenylketonuria: a phase III, randomized, double-blind, placebo-controlled study. J Pediatr 2009;154:700-7. [PubMed]
- Gramer G, Burgard P, Garbade S, et al. Effects and clinical significance of tetrahydrobiopterin supplementation in phenylalanine hydroxylase-deficient hyperphenylalaninaemia. J Inherit Metab Dis 2007;30:556-62. [PubMed]
- Pietz J, Kreis R, Rupp A, et al. Large neutral amino acids block phenylalanine transport into brain tissue in patients with phenylketonuria. J Clin Invest 1999;103:1169-78. [PubMed]
- Matalon R, Michals-Matalon K, Bhatia G, et al. Double blind placebo control trial of large neutral amino acids in treatment of PKU: Effect on blood phenylalanine. J Inherit Metab Dis 2007;30:153-8. [PubMed]
- Matalon R, Michals-Matalon K, Bhatia G, et al. Large neutral amino acids in the treatment of phenylketonuria (PKU). J Inherit Metab Dis 2006;29:732-8. [PubMed]
- Sanjurjo P, Aldamiz L, Georgi G, et al. Dietary threonine reduces plasma phenylalanine levels in patients with hyperphenylalaninemia. J Pediatr Gastroenterol Nutr 2003;36:23-6. [PubMed]
- Koch R, Moseley KD, Yano S, et al. Large neutral amino acid therapy and phenylketonuria: a promising approach to treatment. Mol Genet Metab 2003;79:110-3. [PubMed]
- Rocha JC, Martel F. Large neutral amino acids supplementation in phenylketonuric patients. J Inherit Metab Dis 2009;32:472-80. [PubMed]
- Ney DM, Blank RD, Hansen KE. Advances in the nutritional and pharmacological management of phenylketonuria. Curr Opin Clin Nutr Metab Care 2014;17:61-8. [PubMed]
- Etzel MR. Manufacture and use of dairy protein fractions. J Nutr 2004;134:996S-1002S. [PubMed]
- Lim K, van Calcar SC, Nelson KL, et al. Acceptable low-phenylalanine foods and beverages can be made with glycomacropeptide from cheese whey for individuals with PKU. Mol Genet Metab 2007;92:176-8. [PubMed]
- Macleod EL, Ney DM. Nutritional Management of Phenylketonuria. Ann Nestle Eng 2010;68:58-69. [PubMed]
- Wang Z, Ying Z, Bosy-Westphal A, et al. Evaluation of specific metabolic rates of major organs and tissues: comparison between men and women. Am J Hum Biol 2011;23:333-8. [PubMed]
- Fang B, Eisensmith RC, Li XH, et al. Gene therapy for phenylketonuria: phenotypic correction in a genetically deficient mouse model by adenovirus-mediated hepatic gene transfer. Gene Ther 1994;1:247-54. [PubMed]
- Ding Z, Harding CO, Thöny B. State-of-the-art 2003 on PKU gene therapy. Mol Genet Metab 2004;81:3-8. [PubMed]
- Thomas CE, Ehrhardt A, Kay MA. Progress and problems with the use of viral vectors for gene therapy. Nat Rev Genet 2003;4:346-58. [PubMed]
- Mochizuki S, Mizukami H, Ogura T, et al. Long-term correction of hyperphenylalaninemia by AAV-mediated gene transfer leads to behavioral recovery in phenylketonuria mice. Gene Ther 2004;11:1081-6. [PubMed]
- Niidome T, Huang L. Gene therapy progress and prospects: nonviral vectors. Gene Ther 2002;9:1647-52. [PubMed]
- Lechardeur D, Lukacs GL. Intracellular barriers to non-viral gene transfer. Curr Gene Ther 2002;2:183-94. [PubMed]
- Eavri R, Lorberboum-Galski H. A novel approach for enzyme replacement therapy. The use of phenylalanine hydroxylase-based fusion proteins for the treatment of phenylketonuria. J Biol Chem 2007;282:23402-9. [PubMed]
- MacDonald MJ, D’Cunha GB. A modern view of phenylalanine ammonia lyase. Biochem Cell Biol 2007;85:273-82. [PubMed]
- Koukol J, Conn EE. The metabolism of aromatic compounds in higher plants. IV. Purification and properties of the phenylalanine deaminase of Hordeum vulgare. J Biol Chem 1961;236:2692-8. [PubMed]
- Fritz RR, Hodgins DS, Abell CW. Phenylalanine ammonia-lyase. Induction and purification from yeast and clearance in mammals. J Biol Chem 1976;251:4646-50. [PubMed]
- Hoskins JA, Holliday SB, Greenway AM. The metabolism of cinnamic acid by healthy and phenylketonuric adults: A kinetic study. Biomed Mass Spectrom 1984;11:296-300. [PubMed]
- Hoskins JA, Gray J. Phenylalanine ammonia lyase in the management of phenylketonuria: the relationship between ingested cinnamate and urinary hippurate in humans. Res Commun Chem Pathol Pharmacol 1982;35:275-82. [PubMed]
- Hoskins JA, Jack G, Wade HE, et al. Enzymatic control of phenylalanine intake in phenylketonuria. Lancet 1980;1:392-4. [PubMed]
- Sarkissian CN, Shao Z, Blain F, et al. A different approach to treatment of phenylketonuria: Phenylalanine degradation with recombinant phenylalanine ammonia lyase. Proc Natl Acad Sci U S A 1999;96:2339-44. [PubMed]
- Gámez A, Sarkissian CN, Wang L, et al. Development of pegylated forms of recombinant Rhodosporidium toruloides phenylalanine ammonia-lyase for the treatment of classical phenylketonuria. Mol Ther 2005;11:986-9. [PubMed]
- Gámez A, Wang L, Sarkissian CN, et al. Structure-based epitope and PEGylation sites mapping of phenylalanine ammonia-lyase for enzyme substitution treatment of phenylketonuria. Mol Genet Metab 2007;91:325-34. [PubMed]
- Ikeda K, Schiltz E, Fujii T, et al. Phenylalanine ammonia-lyase modified with polyethylene glycol: potential therapeutic agent for phenylketonuria. Amino Acids 2005;29:283-7. [PubMed]
- Sarkissian CN, Gámez A, Wang L, et al. Preclinical evaluation of multiple species of PEGylated recombinant phenylalanine ammonia lyase for the treatment of phenylketonuria. Proc Natl Acad Sci U S A 2008;105:20894-9. [PubMed]
- Sarkissian CN, Kang TS, Gámez A, et al. Evaluation of orally administered PEGylated phenylalanine ammonia lyase in mice for the treatment of Phenylketonuria. Mol Genet Metab 2011;104:249-54. [PubMed]
- Wang L, Gamez A, Sarkissian CN, et al. Structure-based chemical modification strategy for enzyme replacement treatment of phenylketonuria. Mol Genet Metab 2005;86:134-40. [PubMed]
- Abstracts of the 6th Annual Meeting of the American Society of Gene Therapy. June 4-8, 2003. Washington, DC, USA. Mol Ther 2003;7:S1-509. [PubMed]
- Longo N, Thomas J, Wasserstein M, et al. Evaluation of long-term safety and efficacy with rAvPALPEG for control of blood phenylalanine levels in adults with phenylketonuria (PKU). J Inher Metab Dis 2015;38 Suppl 1:S41.
- Hosny EA, Al-Shora HI, Elmazar MM. Oral delivery of insulin from enteric-coated capsules containing sodium salicylate: effect on relative hypoglycemia of diabetic beagle dogs. Int J Pharm 2002;237:71-6. [PubMed]
- Shah RM, D’mello AP. Strategies to maximize the encapsulation efficiency of phenylalanine ammonia lyase in microcapsules. Int J Pharm 2008;356:61-8. [PubMed]
- Liu J, Jia X, Zhang J, et al. Study on a novel strategy to treatment of phenylketonuria. Artif Cells Blood Substit Immobil Biotechnol 2002;30:243-57. [PubMed]
- Ng DT, Sarkar CA. Nisin-inducible secretion of a biologically active single-chain insulin analog by Lactococcus lactis NZ9000. Biotechnol Bioeng 2011;108:1987-96. [PubMed]
- Braat H, Rottiers P, Hommes DW, et al. A phase I trial with transgenic bacteria expressing interleukin-10 in Crohn's disease. Clin Gastroenterol Hepatol 2006;4:754-9. [PubMed]
- Steidler L, Hans W, Schotte L, et al. Treatment of Murine Colitis by Lactococcus lactis Secreting Interleukin-10. Science 2000;289:1352-5. [PubMed]
- de Moreno de LeBlanc A, LeBlanc JG, Perdigón G, et al. Oral administration of a catalase-producing Lactococcus lactis can prevent a chemically induced colon cancer in mice. J Med Microbiol 2008;57:100-5. [PubMed]
- Drouault S, Juste C, Marteau P, et al. Oral Treatment with Lactococcus lactis Expressing Staphylococcus hyicus Lipase Enhances Lipid Digestion in Pigs with Induced Pancreatic Insufficiency. Appl Environ Microbiol 2002;68:3166-8. [PubMed]
- Sidhu H, Allison MJ, Chow JM, et al. Rapid reversal of hyperoxaluria in a rat model after probiotic administration of Oxalobacter formigenes. J Urol 2001;166:1487-91. [PubMed]
- Evaluation of health and nutritional properties of powder milk and live lactic acid bacteria. Food and Agriculture Organization of the United Nations and World Health Organization expert consultation report, FAO, Rome, Italy, 2001. Available online: http://www.isapp.net/Portals/0/docs/News/expertconsultation2001.pdf
- Turpin W, Humblot C, Thomas M, et al. Lactobacilli as multifaceted probiotics with poorly disclosed molecular mechanisms. Int J Food Microbiol 2010;143:87-102. [PubMed]
- Fuller R. Probiotics: The scientific basis. London: Chapman and Hall, 1992:15.
- Venturi A, Gionchetti P, Rizzello F, et al. Impact on the composition of the faecal flora by a new probiotic preparation: preliminary data on maintenance treatment of patients with ulcerative colitis. Aliment Pharmacol Ther 1999;13:1103-8. [PubMed]
- Nobaek S, Johansson ML, Molin G, et al. Alteration of intestinal microflora is associated with reduction in abdominal bloating and pain in patients with irritable bowel syndrome. Am J Gastroenterol 2000;95:1231-8. [PubMed]
- Cash BD. Emerging role of probiotics and antimicrobials in the management of irritable bowel syndrome. Curr Med Res Opin 2014;30:1405-15. [PubMed]
- Steidler L. Genetically engineered probiotics. Best Pract Res Clin Gastroenterol 2003;17:861-76. [PubMed]
- Mital BK, Garg SK. Anticarcinogenic, Hypocholesterolemic, and Antagonistic Activities of Lactobacillus acidophilus. Crit Rev Microbiol 1995;21:175-214. [PubMed]
- Simenhoff ML, Dunn SR, Zollner GP, et al. Biomodulation of the toxic and nutritional effects of small bowel bacterial overgrowth in end-stage kidney disease using freeze-dried lactobacillus acidophius. Miner Electrolyte Metab 1996;22:92-6. [PubMed]
- Pouwels PH, Leer RJ, Boersma WJ. The potential of Lactobacillus as a carrier for oral immunization: Development and preliminary characterization of vector systems for targeted delivery of antigens. J Biotechnol 1996;44:183-92. [PubMed]
- Pouwels PH, Leer RJ, Shaw M, et al. Lactic acid bacteria as antigen delivery vehicles for oral immunization purposes. Int J Food Microbiol 1998;41:155-67. [PubMed]
- Balcázar JL, de Blas I, Ruiz-Zarzuela I, et al. Changes in intestinal microbiota and humoral immune response following probiotic administration in brown trout (Salmo trutta). Br J Nutr 2007;97:522-7. [PubMed]
- Drouault S, Corthier G, Ehrlich SD, et al. Survival, Physiology, and Lysis of Lactococcus lactis in the Digestive Tract. Appl Environ Microbiol 1999;65:4881-6. [PubMed]
- Klijn N, Weerkamp AH, de Vos WM. Genetic marking of Lactococcus lactis shows its survival in the human gastrointestinal tract. Appl Environ Microbiol 1995;61:2771-4. [PubMed]
- Marteau P, Shanahan F. Basic aspects and pharmacology of probiotics: an overview of pharmacokinetics, mechanisms of action and side-effects. Best Pract Res Clin Gastroenterol 2003;17:725-40. [PubMed]
- Nouaille S, Ribeiro LA, Miyoshi A, et al. Heterologous protein expression and delivery systems for Lactococcus Lactis. Genet Mol Res 2003;2:102-11. [PubMed]
- Prakash S, Chang TM. Microencapsulated genetically engineered live E. coli DH5 cells administered orally to maintain normal plasma urea level in uremic rats. Nat Med 1996;2:883-7. [PubMed]
- Prakash S, Chang TM. Artificial cells microencapsulated genetically engineered E. coli DH5 cells for the lowering of plasma creatinine in-vitro and in-vivo. Artif Cells Blood Substit Immobil Biotechnol 2000;28:397-408. [PubMed]
- Ma Y, Liu J, Hou J, et al. Oral administration of recombinant Lactococcus lactis expressing HSP65 and tandemly repeated P277 reduces the incidence of type I diabetes in non-obese diabetic mice. PLoS One 2014;9:e105701. [PubMed]
- Baedeker M, Schulz GE. Overexpression of a designed 2.2 kb gene of eukaryotic phenylalanine ammonia-lyase in Escherichia coli. FEBS Lett 1999;457:57-60. [PubMed]
- Faulkner JD, Anson JG, Tuite MF, et al. High-level expression of the phenylalanine ammonia lyase-encoding gene from Rhodosporidium toruloides in Saccharomyces cerevisiae and Escherichia coli using a bifunctional expression system. Gene 1994;143:13-20. [PubMed]
- Jia SR, Cui JD, Li Y, et al. Production of l-phenylalanine from trans-cinnamic acids by high-level expression of phenylalanine ammonia lyase gene from Rhodosporidium toruloides in Escherichia coli. Biochemical Engineering Journal 2008;42:193-7.
- Frank DN, St Amand AL, Feldman RA, et al. Molecular-phylogenetic characterization of microbial community imbalances in human inflammatory bowel diseases. Proc Natl Acad Sci U S A 2007;104:13780-5. [PubMed]
- Erickson RH, Gum JR Jr, Lindstrom MM, et al. Regional Expression and Dietary Regulation of Rat Small Intestinal Peptide and Amino Acid Transporter mRNAs. Biochem Biophys Res Commun 1995;216:249-57. [PubMed]