A comparative study between traditional head measurement and structured light three-dimensional scanning when measuring infant head shape
Introduction
Head-shape problems are common among newborns and young children. Foreign studies have reported that the incidence of plagiocephaly in infants is 8.2–46.6% (1-3), while the incidence of head problems in 4,456 full-term infants of 0–6 months in a Chinese study was about 56.5% (4). These findings suggest that the incidence of head problems in infants is common in the first few months after birth, and different races, regions, and cultures experience different cranial development.
For general head-shape problems in early life, the first step is diagnosis. For the identification of abnormal head shape in infants, the first and most important factor is to solve any problems related to measurement methods, since the measurements are important for determining the clinical severity, classification, and treatment of plagiocephaly. The second step is prevention and treatment, which involves advocating for early postpartum health education, as this can be conducive to the timely prevention of abnormal head shape.
At present, the most commonly used methods for head-shape measurement on the Chinese mainland are traditional head measurement and structured light three-dimensional (3D) scanning. Although Ifflaender et al. (5) reported the differences in head circumference (HC) measurements between those done by the human hand and those done by 3D scanning, and a Bland-Altman plot showed that the two methods were interchangeable, Ifflaender et al. (5) only compared HC and head volume. No comparison was made between the Cranial Vault Asymmetry Index (CVAI) and the cranial index (CI). In this study, a structured light (Spectra 3D) 3D scanning system and traditional head measurement by hand were used to measure infant skull parameters, and the correlation, consistency, and credibility of the two methods were compared.
We present the following article in accordance with the STROBE reporting checklist (available at https://dx.doi.org/10.21037/tp-21-186).
Methods
The calculation of sample size
The following formula was used to calculate the paired sample size: n=(tα+tβ)2σ2/δ2. In this formula, α=0.05/2, β=0.1, δ is the allowable error, and σ is the standard deviation. Based on data acquired in our previous studies (4), in terms of cranial vault asymmetry index (CVAI), the mean difference between the spreading caliper and 3D measurements was approximately 0.57 mm, and the standard deviation was approximately 2.42 mm. According to literature reports (6), the allowable error is 1 mm, the confidence level is 1−x =0.95, the power of a test (1−β) was 0.9, and the number of samples required was 64. With reference to our previous studies, for the CI, the mean difference between the spreading caliper measurement and the 3D measurement was approximately 0.61 mm, and the standard deviation was approximately 1.71 mm. The allowable error was 1 mm, the confidence level was 1−x = 0.95, the power of a test (1−β) was 0.9, and the number of samples required was 32. Based on the CVAI and the CI, the larger sample size of 64 cases were used.
Subjects
Between May 15, 2020 and July 31, 2020, a total of 76 infants, aged from 3 months to 2.5 years old, visited the Pediatric Child Health Clinic of the Second Affiliated Hospital of the Army Medical University (China), and agreed to participate in the present study. The inclusion criteria were as follows: infants between 3 months and 2.5 years of age, without prior disease, who came to the outpatient clinic for normal child care, and had informed consent signed by their parents. The exclusion criteria were infants younger than 3 months, infants older than 2.5 years of age, and infants whose families did not wish them to be included in the study.
A total of 76 children met the inclusion criteria. Each infant was measured using traditional head measurement procedures and structured light 3D scanning by different operators (operators A and B, respectively) in two different consulting rooms on the same day. Operator A successfully measured 76 infants using a tape measure and a spreading caliper, and operator B used a Spectra 3D scanner system (Vorum Inc., Vancouver, Canada) to scan 71 infants (5 cases of invalid data were excluded, i.e., 3 cases of inaccurate 3D data caused by crying, 1 case of failure to cooperate with the scanning after waking up, and 1 case of data loss caused by scanning instrument failure). In the end, 71 cases with valid data were included in the analysis, as shown in Figure 1. These 71 infants included 39 males and 32 females; 32 cases were delivered naturally, and 39 cases were delivered by cesarean section. The mean age was 7.65 months ± 17.01 days, the minimum exact age was 3 months and 19 days, and the maximum age was 29 months and 20 days. The gestational age was 37.33±0.38 weeks, the minimum gestational age being 26 weeks and the maximum gestational age being 41 weeks plus 2 days.
Procedure
The study was conducted in accordance with the Declaration of Helsinki (as revised in 2013). The study was approved by ethics committee of Second Affiliated Hospital of Army Medical University (No. 2021-044-01) and informed consent was taken from all the infants’ parents or guardians. The tests were performed in accordance with the approved guidelines and regulations of the participating institution. Before the examination, the infants’ guardians received an explanation of the examination methods, tools, precautions, and purpose of the study, and the results were explained following the examination. The guardians were allowed to accompany the infants throughout the study to avoid irritability or crying due to their being in an unfamiliar environment. In this study, operators A and B had received relevant professional training and passed an examination. Within the same half-day period, in different consulting rooms, the infants successively underwent traditional head measurement by operator A using a tape measure, and a spreading caliper (Figure 2A). A caregiver held the infant’s head while operator A used a spreading caliper to measure the skull type (see Figure 2B-2F for details). When recording the data, the operators and nursing staff observed whether the data was accurate, repeated the measurement three times, and took the average as the final result. After the infant had been measured by operator A, they were transferred to a different consulting room where operator B used a structured light 3D scanning system to measure the cranial type (Figure 3A). During this procedure, the infant initially wore a tight-fitting 3D head cover (Figure 3A); then, the middle point of the glabella, the left tragion, the right tragion, and the middle philtrum point were marked (Figure 3B). Next, the Spectra dynamic scanner (Vorum Inc., Vancouver, Canada) was used to do the scan (Figure 3A). The data collected by the scanner were uploaded to a computer and analyzed by the Cranial Comparison Utility (CCU, Vorum Inc.) data analysis program (Figure 3C). The transverse, anteroposterior, left eyebrow–right occipitolateral, and right eyebrow–left occipitolateral diameters, and the HC of the same subject was measured and obtained using the above two methods, and the plagiocephaly and cranial symmetry indexes were determined (7). Operators A and B did not know one another’s measurement results, and their five measurement results were subsequently compared.
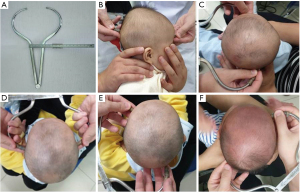
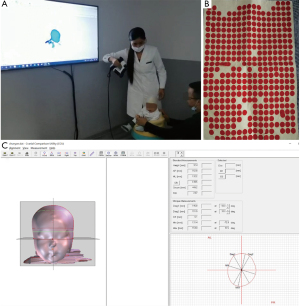
Traditional head measurement
Trained examiners carried out the measurements according to Wilbrand’s standardized measurement scheme (8). The HC measurement tool was a tape measure. The cranial measurement tool was a KWJ124 spreading caliper; the measurement range was 0–300 mm, and the GB5704.3-85 executive production standard was applied (Figure 2A). The infant was placed in an upright position, and if the child’s head did not remain upright by itself, it was held upright by the child’s parent or guardian (Figure 2B-2F).
All cranial data were measured three times, and the average values were taken as the final results. HC was considered to be the length from the upper edge of the eyebrow arch to the occipital tuberosity around the head, while the head anterior–posterior diameter, also known as head length, was taken as the distance from the glabella to the opisthocranion, measured with a spreading caliper; the measuring line had to be parallel to the Frankfurt line (Figure 2B,2C). For the head transverse diameter, also known as head width, the measurement point was 1 cm above the otobasion superius; the linear distance between the measuring points on both sides was measured using a spreading caliper (Figure 2D). The oblique diameter was taken as the linear distance from one frontotemporal point to the opposite herringbone point, in other words, from the right occipital diameter of the left eyebrow (Figure 2E) to the left occipital diameter of the right eyebrow (Figure 2F). With regard to the above two diameters, the long diameter was called diagonal A (DA), and the short diameter diagonal B (DB).
The derivative values were calculated according to the measured values. CVAI is the difference between the oblique diameters of the two sides of the head, denoted as DA–DB (in cm). The CI of symmetry was equal to head width/head length ×100%.
The structured light 3D scanner and its characteristics
A structured light 3D scanner is a device that uses structured light and two cameras to create 3D images (Figure 3). The principle is to project the grating fringe, the thickness (phase) of which changes periodically on the surface of an object. Then, two cameras arranged at a certain angle will input the raster stripe image, which is distorted by the modulation of the object surface to the computer. The data processing system of the scanner superposes and calculates the obtained grating fringe image and, finally, outputs the real 3D surface image. During the scanning procedure, the following four marker points were used to obtain the skull measurement report: (I) the middle point of the glabella, i.e., the midpoint between the eyebrows; (II) the left tragion, i.e., the junction of the foot of the left helix and the tragus; (III) the right tragion, i.e., the junction of the foot of the right helix and the tragus; and (IV) the middle philtrum point, i.e., the middle point between the nose and upper lip.
The measurement of the HC and cranial length is also called the anteroposterior diameter, i.e., the anterior–posterior (AP) length. The measurement of cranial width using the left and right tragus markers is also known as the transverse diameter, i.e., the middle-line (ML) cranial breadth. To display the diagonal difference, the left and right diagonal distance was measured at an angle of 30°, and the cephalic ratio (CR) was calculated. The highest value was selected. The screened values of the AP length, ML cranial breadth, CR, HC, diagonal distance, and CVAI were recorded.
The structured light 3D scanning was carried out in a quiet room. The room was equipped with blackout curtains to prevent outdoor light from entering the room. All the infants were scanned in the sitting position. A knitted headcover that exposed the face was put on the infant’s head, with the collar pulled as low as possible to expose the neck, and matching sticker dots were stuck on the left and right tragus. It took the Spectra 3D scanner three to 6 min to do a scan, and operator B used the Spectra 3D dynamic scanning system to collect 3D images of the subject’s head (Figure 3A). When the scanning was completed, and after obtaining a 3D image of the subject’s head, marker points were placed at the center of the infant’s eyebrows, left and right tragus, and the chin before the measurements were taken (Figure 3B). The data were then saved, and data analysis was conducted using the CCU analysis software (Figure 3C). The software produced a report that included measurements of the patient’s head, including CVAI data, and this report was filed with the subject’s records.
Statistical analysis
Pearson’s correlation coefficient was calculated for the traditional head measurements and structured light 3D scanning measurements. The accuracy was evaluated using a Bland–Altman plot and calculation deviation. The data were analyzed using IBM SPSS Statistics 24.0 software, and P<0.05 was considered statistically significant.
Results
The correlation between traditional head measurement and structured light 3D scanning
Pearson’s correlation coefficient between the traditional head measurement and structured light 3D scanning for 71 cases was calculated using a paired t-test. The average difference (95% CI) was within 0.633–0.988. Pearson’s r revealed that the correlation between the two approaches was very high, the r value was 0.793–0.980, and the correlation coefficients of the transverse diameter, anteroposterior diameter, HC, and the CI of symmetry were all higher than 0.9 (the correlation coefficient of CVAI was the lowest among these at 0.793). The P values of the above measurement data were all <0.001, suggesting that they were closely related (Table 1).
Table 1
Items | Traditional head measurement | Structured light 3D scanning | Median difference (95% CI) | r value | P value |
---|---|---|---|---|---|
Transverse diameter (cm) | 12.31±0.85 | 12.82±0.82 | 0.942–0.984 | 0.969 | <0.001 |
Anteroposterior diameter (cm) | 14.13±0.81 | 14.74±0.81 | 0.969–0.988 | 0.980 | <0.001 |
Oblique diameter D1 (cm) | 13.21±0.73 | 14.20±0.70 | 0.812–0.948 | 0.898 | <0.001 |
Oblique diameter D2 (cm) | 13.58±0.71 | 14.65±0.71 | 0.819–0.948 | 0.892 | <0.001 |
Circumference (cm) | 42.99±2.15 | 43.65±2.00 | 0.892–0.989 | 0.954 | <0.001 |
Plagiocephaly index (mm) | 4.03±3.03 | 4.84±3.62 | 0.633–0.887 | 0.793 | <0.001 |
Cranial index (%) | 87.28±6.20 | 87.13±5.62 | 0.923–0.980 | 0.960 | <0.001 |
The consistency between traditional head measurement and structured light 3D scanning
Figure 4 shows the Pearson’s r and the Bland-Altman plot of head shape data measured using traditional head measurement and the structured light 3D scanner. The correlation between the two measurement types was confirmed; the Pearson’s r values were 0.954 (P<0.001, HC), 0.793 (P<0.001, CVAI), and 0.960 (P<0.001, CI of symmetry). The Bland-Altman plot shows the relationship between the difference and mean of the HC, and the plagiocephaly and cranial indexes. For the CVAI and CI of symmetry between the two different measurement methods, the scatter points were essentially in the 95% consistency bound interval. These results indicate that there was little difference between the average values of the measurement data of the two groups. In addition, the system error of the two measurement methods was small, and overall, they showed good consistency. These results all suggest that using traditional head measurement and using a structured light 3D scanner are both suitable approaches for the measurement of infant head shape.
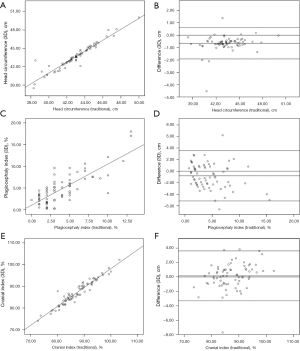
Discussion
The current head-shape measurement methods used with infants are traditional head measurement, structured light 3D scanning, and 3D computed tomography scanning (9). Traditional head measurement is a simple and inexpensive procedure, which makes use of a traditional instrument called a spreading caliper; however, examiners require on-site training to accurately locate the bone markers and prevent the displacement of soft tissue, which can otherwise lead to measurement inconsistencies (10). Mortenson and Steinbok (11) independently studied the reliability and validity of the method by recording the anthropometric parameters obtained when two different clinicians used a spreading caliper to measure the same subjects. Their results suggest that skilled examiners are needed to measure head shape when using a spreading caliper, as the accuracy and proficiency of positioning and carrying out the measurement will affect the verdict of deformity degree. As infants and young children frequently cannot remain sufficiently still, the spreading caliper cannot always be accurately positioned in the same position; for this reason, generally speaking, three measurements are taken, and the average value is used as the final result. The operator of the spreading caliper must receive professional training regarding body surface marking, and the head of the infant sometimes has to be supported and held by a parent or guardian. The 3D scanning method, on the other hand, can save the original 3D image using computer software and can statically illustrate the head shape of the infant, which means that the body surface marking proficiency requirements of the operator are much reduced.
Ifflaender et al. (5) used the repeated measurement method to compare the HC measurements of 282 infants obtained both by hand and by 3D scanning. A Bland-Altman plot showed that the two methods were interchangeable (BIAS −0.45%; 95% limits of agreement: −4.55–3.65%), the average difference between the two measurement methods being close to zero, and the value measured manually was slightly lower (5). Consistent with the results of this study, the HC measured by traditional head measurement was 42.99±2.15 cm and structured light 3D scanning was 43.65±2.00 cm, the 95% CI of the difference in medians was 0.892–0.989 cm (Table 1), and the two were highly correlated (Figure 4).
Three-dimensional scanning has important research value in the field of plagiocephaly correction (12). Conkle et al. (13) reported on the reliability and accuracy of height, HC, and arm circumference measurements of 474 children aged 0–5 years old in Georgia (USA). The results revealed that the reliability of the above indexes derived from repeated 3D measurement and standard anthropometry was within 1 mm; however, 3D scanning overestimated the height and HC by 6 and 3 mm, respectively, and underestimated arm circumference by 2 mm, suggesting that the accuracy of 3D scanning requires additional improvement (13).
With the development of modern technology, structured light 3D scanning systems have undergone significant development. Second-generation systems are represented by handheld 3D scanners (14). These include the Spectra scanner (Vorum Inc., Vancouver, Canada), which was used in this study and is dedicated to medical treatment. Its accuracy has been increased to 0.1 mm, which complies with European Conformity safety certification, and it is harmless to the human body (15). In addition, Burkhardt used MRI to determine the difference between total brain volume and cranial volume measured by 3D laser scanning (16). Although MRI is a safe and radio-free examination technique, it takes a long time (15–20 min) and the instrument is noisy. As a result, it can be difficult to get children to cooperate and maintain a fixed position when awake, affecting the examination results. Therefore, its clinical use is not popular. On the other hand, the spreading calipers and second-generation 3D scanners used in this study present no harm to infants and are, thus, the two most widely-used methods in the field of infant head-shape measurement.
One study (17) reported that, as a minimally invasive measuring technique for neonatal anthropometry, structured light 3D scanning has very good prospects in the head-shape measurement of premature infants or newborns; however, although the study indicated a high correlation between the data of structured light 3D scanning and a tape measure, it only focused on measuring the HC (17). In the present study, the application of structured light 3D scanning and the traditional head measurement of infant cranial type were compared. So, measurements included not only HC but also head width and length, as well as left and right transcranial oblique diameter, which can be used to comprehensively evaluate the development of cranial type in infants. The Bland–Altman plot showed a high correlation and consistency between the two methods (Figure 4). Figure 4B,4D,4F show that the consistency of the two methods was the highest when measuring HC, followed by the CI of symmetry, while the consistency of the plagiocephaly index was lower than that of HC and the CI of symmetry. However, this study found that the 95% confidence interval of traditional head measurement and structured light 3D scanning was between 0.633 and 0.988. Pearson’s correlation coefficient revealed that the correlation between traditional head measurement and structured light 3D scanning measurement was very high (r=0.793–0.980). Furthermore, the correlation coefficients of the transverse diameter, anteroposterior diameter, HC, and the CI of symmetry were more than 0.9 (the correlation coefficient of the CVAI was the lowest at 0.793). The P values of the above measurement data were all <0.001, suggesting that they were closely related (Table 1). In summary, the results of the present study suggest that both methods can be used to measure the head shape of infants with accuracy.
Structured light 3D scanning is more comprehensive than traditional head measurement in terms of obtaining the head-shape data of infants and young children. The former can calculate the skull volume of four quadrants [Q1 volume (A/L), Q2 volume (A/R), Q3 volume (P/R), Q4 volume (P/L)], the displacement difference between the two ears (anterior ear shift), and the height from the base of the head to the top of the skull (vertex height). These data cannot be measured by manual traditional head measurements and are very important for the follow-up correction of infants with an abnormal head shape. Therefore, for infants with an abnormal head shape, structured light 3D scanning is recommended to obtain multi-parameter data for customizing a helmet.
Both structured light 3D scanning and traditional head measurements require the measurement operator to have a degree of proficiency. The former requires higher practical skills from measurement operators alongside the subject’s cooperation. In this study, three infants were crying, one infant could not cooperate after falling asleep, and one infant's data was lost, so they all had to be excluded. Three-dimensional cephalometric scanning can also not be used in infants of three months old with an unstable head hold (because its success requires the infant to be in a sitting position with their head held vertically during measurement). In contrast, the traditional head measurement method does not require a high degree of cooperation from the infant, and so even those who fall asleep can be measured using this approach. Moreover, it is more convenient than 3D scanning and can be completed in as little as 1–2 min.
The marked points of structured light 3D scanning measurement are manually created, and as such, there may be some deviation between the setting points of different operators. Similarly, human error may also occur in traditional head measurements because of the active nature of infants and children. When taking traditional head measurements, it is often difficult to accurately repeat the exact positioning. Due to this, normally three measurements are taken, and the average value is used as the final result.
Compared with traditional head measurement, structured light 3D scanning has certain disadvantages. First, the environmental requirements of structured light 3D scanning are greater than those of traditional head measurements, as it must be conducted in a dark environment because 3D scanning uses visible light. It is also complex to operate, time-consuming, and more technically demanding of the operator, and requires the cooperation of the infant being tested. However, its advantages outweigh those of taking traditional head measurements. Structured light 3D scanning provides more data parameters, such as the volume of the skull, the displacement difference between the two ears, and the height from the base of the head to the top of the skull, and, overall, it is better for deriving the measurements of infants with an abnormal head shape to facilitate customized helmet correction where needed (18).
Conclusions
Based on the results of this study, the authors make the following recommendations for infant head measurements. Both traditional measurement and 3D scanning can be used for infant head measurement. However, for primary units, traditional measurement is better because it is economical, simple, time-saving, widely adaptable, and can be measured regardless of the child’s state, making it the first choice for head shape screening. For units with higher measurement requirements, 3D scanning is better because it provides more and precise parameters than the spreading caliper, which is very useful for the diagnosis of head shape abnormalities and facilitates custom helmet orthopedics. The combination of the two meets the national conditions of Chinese children's head measurement.
Acknowledgments
The authors would like to thank the participating infants and their parents for making this study possible. We also thank all the participants and the staff at the hospital who helped develop the study. Thanks to Nanjing XingXing O&P Technology Co. Ltd. for the help of 3D scanning measurement. And we thank Liz Coles for language editing of this article.
Funding: This work was supported by Chongqing Joint Medical Scientific Research Project of Science and Health (#2021MSXM091).
Footnote
Reporting Checklist: The authors have completed the STROBE reporting checklist. Available at https://dx.doi.org/10.21037/tp-21-186
Data Sharing Statement: Available at https://dx.doi.org/10.21037/tp-21-186
Conflicts of Interest: All authors have completed the ICMJE uniform disclosure form (available at https://dx.doi.org/10.21037/tp-21-186). The authors have no conflicts of interest to declare.
Ethical Statement: The authors are accountable for all aspects of the work in ensuring that questions related to the accuracy or integrity of any part of the work are appropriately investigated and resolved. The study was conducted in accordance with the Declaration of Helsinki (as revised in 2013). The study was approved by ethics committee of Second Affiliated Hospital of Army Medical University (No. 2021-044-01) and informed consent was taken from all the infants’ parents or guardians.
Open Access Statement: This is an Open Access article distributed in accordance with the Creative Commons Attribution-NonCommercial-NoDerivs 4.0 International License (CC BY-NC-ND 4.0), which permits the non-commercial replication and distribution of the article with the strict proviso that no changes or edits are made and the original work is properly cited (including links to both the formal publication through the relevant DOI and the license). See: https://creativecommons.org/licenses/by-nc-nd/4.0/.
References
- Bialocerkowski AE, Vladusic SL, Wei Ng C. Prevalence, risk factors, and natural history of positional plagiocephaly: a systematic review. Dev Med Child Neurol 2008;50:577-86. [Crossref] [PubMed]
- Ballardini E, Sisti M, Basaglia N, et al. Prevalence and characteristics of positional plagiocephaly in healthy full-term infants at 8-12 weeks of life. Eur J Pediatr 2018;177:1547-54. [Crossref] [PubMed]
- Aarnivala H, Vuollo V, Harila V, et al. The course of positional cranial deformation from 3 to 12 months of age and associated risk factors: a follow-up with 3D imaging. Eur J Pediatr 2016;175:1893-903. [Crossref] [PubMed]
- Yang W, Hu B, Chen J, et al. Analysis of cranial type characteristics in term infants: a multi-center study. BMC Pediatr 2021;21:20. [Crossref] [PubMed]
- Ifflaender S, Rüdiger M, Koch A, et al. Three-dimensional digital capture of head size in neonates - a method evaluation. PLoS One 2013;8:e61274 [Crossref] [PubMed]
- Lang TA, Altman DG. Basic statistical reporting for articles published in biomedical journals: the "Statistical Analyses and Methods in the Published Literature" or the SAMPL Guidelines. Int J Nurs Stud 2015;52:5-9. [Crossref] [PubMed]
- Aarnivala H, Vuollo V, Heikkinen T, et al. Accuracy of measurements used to quantify cranial asymmetry in deformational plagiocephaly. J Craniomaxillofac Surg 2017;45:1349-56. [Crossref] [PubMed]
- Wilbrand JF, Schmidtberg K, Bierther U, et al. Clinical classification of infant nonsynostotic cranial deformity. J Pediatr 2012;161:1120-5. [Crossref] [PubMed]
- Beaumont CAA, Knoops PGM, Borghi A, et al. Three-dimensional surface scanners compared with standard anthropometric measurements for head shape. J Craniomaxillofac Surg 2017;45:921-7. [Crossref] [PubMed]
- McGarry A, Dixon MT, Greig RJ, et al. Head shape measurement standards and cranial orthoses in the treatment of infants with deformational plagiocephaly. Dev Med Child Neurol 2008;50:568-76. [Crossref] [PubMed]
- Mortenson PA, Steinbok P. Quantifying positional plagiocephaly: reliability and validity of anthropometric measurements. J Craniofac Surg 2006;17:413-9. [Crossref] [PubMed]
- Da Silveira AC, Daw JL Jr, Kusnoto B, et al. Craniofacial applications of three-dimensional laser surface scanning. J Craniofac Surg 2003;14:449-56. [Crossref] [PubMed]
- Conkle J, Suchdev PS, Alexander E, et al. Accuracy and reliability of a low-cost, handheld 3D imaging system for child anthropometry. PLoS One 2018;13:e0205320 [Crossref] [PubMed]
- Han C, Choi H, Jo S, et al. Development of a 3D scanning method to discriminate blocks of Octopus minor with surplus water gain. Food Chem 2020;303:125414 [Crossref] [PubMed]
- Takamatsu A, Hikosaka M, Kaneko T, et al. Evaluation of the Molding Helmet Therapy for Japanese Infants with Deformational Plagiocephaly. JMA J 2021;4:50-60. [PubMed]
- Burkhardt W, Schneider D, Hahn G, et al. Non-invasive estimation of brain-volume in infants. Early Hum Dev 2019;132:52-7. [Crossref] [PubMed]
- Andrews ET, Ashton JJ, Pearson F, et al. Handheld 3D scanning as a minimally invasive measuring technique for neonatal anthropometry. Clin Nutr ESPEN 2019;33:279-82. [Crossref] [PubMed]
- Tamber MS, Nikas D, Beier A, et al. Neurosurgery 2016;79:E632-3. [Crossref]