Early supplementation of folate and vitamin B12 improves insulin resistance in intrauterine growth retardation rats
Introduction
Infants whose weight is < the 10th percentile for gestational age are classified as small for gestational age (SGA), often caused by intrauterine growth retardation (IUGR) (1). Low birth weight infants with IUGR are closely related to adulthood insulin resistance (2,3). Recent studies have shown that SGA infants already had changes in insulin sensitivity during the neonatal period (4,5).
At present, there are many studies on the harm of IUGR, yet the interventional strategies are still limited. Epigenetic modifications such as DNA methylation may be the key mechanism of insulin resistance in IUGR (6). In the same genetic background, food is closely related to epigenetic changes. Animals in the early postnatal period are in a period of rapid development, and epigenetic modifications can be affected by changing the external environment such as food (7,8). Epigenetic forms established during the fetal period can be altered by nutritional interventions early in the postnatal life to improve the metabolic system that is not yet fully mature (9). Folate, vitamin B12, and homocysteine (Hcy), the main members of the methionine cycle, participate in nucleic acid synthesis and protein metabolism in the body (10). It has been reported that raised maternal plasma Hcy concentrations can predict small size at birth, a risk factor for type 2 diabetes mellitus. An association between maternal vitamin B12, folate and Hcy status during pregnancy was studied, showing in utero vitamin B12 and folate deficiency could predict about propensity for greater adiposity and insulin resistance in the offspring (11). Therefore, early nutrition may have a certain effect on later childhood insulin sensitivity (12).
In this study, we established IUGR rat model by both low protein and restricted diet feeding during pregnancy, as well as investigated the effects of supplementation with high folate and vitamin B12 diets in the early postnatal period on the changes in blood glucose, insulin, Hcy, triglyceride and cholesterol levels in IUGR rats. To explore whether early supplementation of folic acid and vitamin B12 would improve insulin resistance in IUGR rats. We present the following article in accordance with the ARRIVE reporting checklist (available at https://tp.amegroups.com/article/view/10.21037/tp-21-498/rc).
Methods
Subjects and grouping
This study was carried out in strict accordance with the recommendations in the Guide for the Care and Use of Laboratory Animals of the Peking University. The protocol was approved by the Committee on the Ethics of Animal Experiments of the Peking University Third Hospital (protocol number: LA2015190). Healthy Sprague-Dawley rats were purchased from the Laboratory Animal Science Department of Peking University Health Science Center (Beijing, China). In the previous study, the IUGR rat model was established by low protein diet throughout pregnancy, but some fetal rats were completely restricted in development and could not survive, which reduced the success rate of the experiment. Therefore, this study was improved and we successfully established IUGR rat model by both low protein and restricted diet feeding during the second and third trimester of pregnancy. Eighteen females, 2.5 months old and weighing 200–250 g, were mated with males, and caged at a ratio of 1:2. The first day of conception was to see the vaginal plug. On the 12th day of conception, 18 pregnant rats were randomly divided into a low (8%) protein diet group (n=12) for IUGR and a standard (20%) diet group (n=6) in a 2:1 ratio using a computer based random order generator. All of rats were kept in individually ventilated cages at a temperature of 24 ℃, humidity of 50%, and a 12/12-hour light/dark cycle with the lights. The standard diet group had unlimited free diet, while the low-protein diet group was restricted to 40% of the diet amount required by the standard diet group.
After the pregnant rats gave birth naturally, the weight of newborn pups within 6 hours of birth was weighed using an electronic balance to the nearest 0.01 g. There were 49 pups with the mean weight of 7.01±0.35 g in the control group, and 90 pups with the mean weight of 4.44±0.58 g in the low-protein group. Pups with the body wight of 2 standard deviations (SD) below the mean of the control group were defined as the IUGR pups, resulting in a total of 84 IUGR pups, and the remaining 6 pubs were excluded because of their high birth weight. IUGR pups were randomly divided into two groups (42 rats/group) in a 1:1 ratio using a computer based random order generator: IUGR intervention group (IUGR-I) and IUGR non-intervention group (IUGR-NI). The lactating female rats in the IUGR-I group were fed with high folate (32 mg/kg) and vitamin B12 (0.16 mg/kg) diet, 4 times that of the standard diet (Table 1). The lactating female rats in the IUGR-NI and in the control were fed with standard diet (folate, 8 mg/kg; vitamin B12, 0.04 mg/kg). The offspring of the three groups were weaned 21 days after birth, and fed with the standard diet to 120 days. Dietary assignments were done by an animal technician who was not involved in the outcome evaluation.
Table 1
Ingredient | Standard diet | Low-protein diet | Standard diet with high folate and vitamin B12 |
---|---|---|---|
Corn (%) | 38.95 | 38.95 | 38.95 |
Corn starch (%) | 31.11 | 43.49 | 31.11 |
Casein (%) | 16.73 | 4.34 | 16.73 |
Flour (%) | 5 | 5 | 5 |
Vegetable oil (%) | 3.76 | 3.76 | 3.76 |
CaCO3 (%) | 0.38 | 0.38 | 0.38 |
Ca(HCO3)2 (%) | 3.23 | 3.23 | 3.23 |
Microelement (%) | 0.5 | 0.5 | 0.5 |
Salt (%) | 0.3 | 0.3 | 0.3 |
Fiber (%) | 0.05 | 0.05 | 0.05 |
Protein (%) | 20 | 8 | 20 |
Folic acid (mg/kg) | 8 | 8 | 32 |
Vitamin B12 (mg/kg) | 0.04 | 0.04 | 0.16 |
Calories (kcal/g) | 30.50 | 30.50 | 30.50 |
IUGR, intrauterine growth retardation.
Date collection and measurement
In this study, male rats were studied in order to avoid hormonal and gender influence. At 21, 60 and 120 days, 8 male pups were randomly picked from each group. We have chosen a small sample size because this study studied the effects of folic acid and vitamin B12 on insulin resistance in IUGR rats for the first time, which was a preliminary exploration of the follow-up mechanism. Isoflurane (2% inhalation) was used for anesthesia, and all efforts were made to minimize pain. After fasting for 12h, blood was collected from the inner canthal vein for fasting plasma glucose (FBG), fasting insulin (FINS), triglyceride and cholesterol. Hcy was measured at 120 days. FBG and FINS levels were measured using a blood glucose meter (Roche, German) and an enzyme-linked immunosorbent insulin assay kit (Mercodia, Sweden), respectively. Hcy, triglyceride and cholesterol were measured using the HITACHI 7150 automatic biochemical analyzer (Hitachi, Japan). To calculate insulin resistance index, we used the HOMA-IR (Homeostasis Model of Assessment-Insulin Resistance) score [FPG (mmol/L) × FINS (mU/L)]/22.5.
Statistical analysis
Statistical analysis was performed using SPSS 20.0 software. Model assumptions were checked by using the Shapiro-Wilk normality test. Data are presented with mean ± SD. Continuous variables between two groups were compared by Student’s t-test. The comparison among three groups was performed using analysis of variance, and the further pairwise comparison was performed using the Student-Newman-Keuls method. Correlation between homocysteine and fasting blood glucose, insulin, HOMA-IR, triglyceride, and cholesterol levels was assessed using Pearson analysis. P<0.05 indicates that the difference is statistically significant.
Results
Birth weight of offspring rat
We established IUGR rat model by both low protein and restricted diet feeding during pregnancy. The average birth weight of offspring rat in the low protein and restricted diet group [(4.44±0.58) g] was lower than that in the control group [(7.03±0.56) g]. The difference was statistically significant (t=15.75, P<0.05). There were 84 IUGR pups, and the incidence of IUGR pups was 93.33% in this study.
Comparisons of serum parameters between the control and the IUGR offspring
IUGR pups who were supplemented with high folate and vitamin B12 during lactation were classified as the intervened IUGR group (IUGR-I); otherwise, the non-intervened IUGR group (IUGR-NI). Comparisons of fasting glucose, insulin, HOMA-IR, triglyceride, cholesterol and Hcy levels between the control, the IUGR-NI and the IUGR-I group are shown in Figure 1. At day 21, there was no difference in fasting glucose, insulin, HOMA-IR, triglyceride, and cholesterol levels across the three groups (P>0.05).
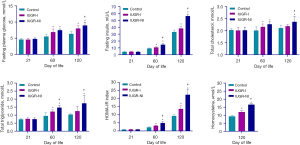
At day 60, insulin, HOMA-IR and triglyceride levels in the IUGR-I group were remarkably lower than those in the IUGR-NI group, although they were still higher than those in the control group (F=38.34, P=0.02; F=49.48, P=0.02; F=17.93, P<0.001, respectively). Fasting glucose and cholesterol levels in the IUGR-I and the IUGR-NI group were higher than those in the control group, but there was no significant difference between the IUGR-I and IUGR-NI group (F=17.14, P=0.01; F=8.34, P<0.001, respectively).
At day 120, the levels of fasting glucose, insulin, HOMA-IR and Hcy in the IUGR-I group were obviously lower than those in the IUGR-NI group, but still higher than those in the control group (F=21.60, P<0.001; F=164.46, P<0.001; F=75.15, P<0.001; F=35.46, P<0.001, respectively). The levels of triglyceride and cholesterol in the IUGR-NI group were dramatically higher than those in the IUGR-I group and control group, but there was no significant difference between the IUGR-I and control group (F=9.80, P<0.001; F=6.12, P<0.001, respectively).
Correlation between homocysteine and fasting glucose, insulin, HOMA-IR, triglyceride, and cholesterol levels in IUGR-I group and IUGR-NI group
Homocysteine levels were positively correlated with fasting glucose, HOMA-IR levels (r=0.863, P=0.006; r=0.725, P=0.042, respectively) in IUGR-I group at day 120 (Table 2). Homocysteine levels were not significantly correlated with insulin, triglyceride and cholesterol levels in IUGR-I group at day 120 (Table 2).
Table 2
Pearson correlation | Homocysteine | |
---|---|---|
r | P value | |
Fasting plasma glucose | 0.863* | 0.006 |
Fasting insulin | 0.165 | 0.697 |
Total cholesterol | 0.646 | 0.083 |
Total triglyceride | 0.604 | 0.113 |
HOMA-IR index | 0.725* | 0.042 |
*, correlation is significant at the 0.05 level. HOMA-IR, Homeostasis Model of Assessment-Insulin Resistance; IUGR-I, intrauterine growth retardation pups were intervened by supplementation with high folate and vitamin B12 during lactation.
There was a positive correlation between homocysteine and fasting glucose levels (r=0.721, P=0.044) in IUGR-NI group at day 120 (Table 3). Homocysteine levels were not significantly correlated with HOMA-IR, insulin, triglyceride, and cholesterol levels in IUGR-NI group at day 120 (Table 3).
Table 3
Pearson correlation | Homocysteine | |
---|---|---|
r | P value | |
Fasting plasma glucose | 0.721* | 0.044 |
Fasting insulin | 0.147 | 0.728 |
Total cholesterol | 0.469 | 0.241 |
Total triglyceride | 0.424 | 0.296 |
HOMA-IR index | 0.585 | 0.127 |
*, correlation is significant at the 0.05 level. HOMA-IR, Homeostasis Model of Assessment-Insulin Resistance; IUGR-NI, non-intervened intrauterine growth retardation pups.
Discussion
Infants born SGA are at an increased risk of metabolic syndrome in later life (13). SGA infants may have metabolic abnormalities at birth, but not all will develop insulin resistance in the future (14). Therefore, the implementation of nutritional intervention at this stage may change the body’s immature metabolic system and improve insulin resistance. Yet, the potential mechanism and interventional strategies are still limited. In our study, high amounts of folate and vitamin B12 were supplemented during lactation for IUGR rats. At 21 days after birth, there was no difference in fasting glucose, insulin, HOMA-IR, triglyceride and cholesterol levels among the control, IUGR-I, and IUGR-NI groups. This suggests that IUGR offspring still maintained normal glucose and lipid metabolism in the early postnatal period. From 60 days after birth, insulin resistance and hyperlipidemia occurred in IUGR offspring. This manifests that the compensatory secretion of insulin increases with age, but not enough to maintain the normal balance of glucose and lipid metabolism, leading to insulin resistance. But insulin resistance and hyperlipidemia were improved significantly by folate and vitamin B12 supplementation, indicating that nutritional intervention in the early postnatal period can improve the immature metabolic system established during the fetal period, and the lactation period can be used as a window of early nutritional intervention.
Specifically, studies in both humans and rats have shown that maternal B12 insufficiency is associated with insulin resistance in offspring (15,16). Huang et al. have suggested that maternal supplementation with high folic acid (4 times the normal diet) during pregnancy and lactation improves glucose intolerance and insulin resistance in male mice offspring fed a high-fat diet (17). Girard et al. have indicated that feed-restricted dairy cows receiving a combined supplement of folic acid and vitamin B12 might have enhanced insulin sensitivity (18).
At present, the mechanism by which folate and vitamin B12 improve insulin resistance is still unclear. It has been shown that high Hcy level might be highly correlated with insulin resistance (19-21). Folate and vitamin B12 may alter insulin sensitivity by regulating Hcy level. Setola et al. have shown that supplementation of folate and vitamin B12 improved insulin resistance, along with decreasing homocysteine levels in patients with metabolic syndrome (22). The main metabolic pathway of Hcy in vivo is the synthesis of methionine dependent on vitamin B12 and folic acid (23). When folic acid and vitamin B12 are deficient, the methionine cycle is impaired, leading to hyperhomocysteine (24,25). In this study, the highest serum Hcy concentration was found in the IUGR group, which was reduced significantly by folate and vitamin B12 supplementation. Moreover, Hcy in IUGR-I group was highly positively correlated with fasting glucose and HOMA-IR. Some clinical studies also showed that supplementation of folic acid and vitamin B12, rather than folic acid alone, is likely to be much more effective at lowering of Hcy concentrations, with potential benefits for improved insulin resistance and reduction of risk of vascular disease (26,27). Liu et al. believed that increased homocysteine level enhanced the activity of protein tyrosine phosphatase and inhibited the phosphorylation of insulin β receptor. Protein tyrosine phosphatase played a negative regulatory role in insulin signaling pathway, resulting in reduced glucose uptake ability of liver cells and insulin resistance (28). These data suggest that a fortification policy based on folate and vitamin B12 during lactation or at early neonatal period may improve insulin resistance by reducing Hcy concentration in IUGR offspring.
However, it was reported that insulin resistance induced by hyperglycemia in patients with type 2 diabetes would not change plasma Hcy level, and there was no correlation between insulin resistance and Hcy level (29). Jimmy has suggested that offspring of mothers with low vitamin B12 and high folate were the most insulin resistant (30). Therefore, there is no consistent conclusion as to whether folic acid and vitamin B12 supplementation can improve insulin resistance. The reason may be different from the design of the experimental scheme, or the animal experiment is different from the clinical situation, or folic acid and vitamin B12 have synergistic or antagonistic effect. In addition, the mechanism of the effect of folic acid and vitamin B12 supplementation on insulin sensitivity needs to be confirmed by further studies.
Conclusions
Taken together, our data indicated that early supplementation of folate and vitamin B12 improved insulin resistance and lipid levels in IUGR rats to some extent, along with decreasing homocysteine levels, but not enough to completely repair glucose and lipid metabolism.
Acknowledgments
Funding: This study was financially supported by National Nature and Science Foundation of China (No. 30901614 to YX, No. 81241026 to XW), Beijing Natural Science Foundation (No. S170003 to YX), Peking University Medical cross research seed fund (Medical-X, No. BMU2020MX010), and Peking University Third Hospital Research Fund for outstanding overseas returnees (No. BYSYLXHG2019005 to YX). The funder had no role in study design, data collection and analysis, decision to publish, or preparation of the manuscript.
Footnote
Reporting Checklist: The authors have completed the ARRIVE reporting checklist. Available at https://tp.amegroups.com/article/view/10.21037/tp-21-498/rc
Data Sharing Statement: Available at https://tp.amegroups.com/article/view/10.21037/tp-21-498/dss
Conflicts of Interest: All authors have completed the ICMJE uniform disclosure form (available at https://tp.amegroups.com/article/view/10.21037/tp-21-498/coif). The authors have no conflicts of interest to declare.
Ethical Statement:
Open Access Statement: This is an Open Access article distributed in accordance with the Creative Commons Attribution-NonCommercial-NoDerivs 4.0 International License (CC BY-NC-ND 4.0), which permits the non-commercial replication and distribution of the article with the strict proviso that no changes or edits are made and the original work is properly cited (including links to both the formal publication through the relevant DOI and the license). See: https://creativecommons.org/licenses/by-nc-nd/4.0/.
References
- Hansen DN, Odgaard HS, Uldbjerg N, et al. Screening for small-for-gestational-age fetuses. Acta Obstet Gynecol Scand 2020;99:503-9. [Crossref] [PubMed]
- Nakano Y. Adult-Onset Diseases in Low Birth Weight Infants: Association with Adipose Tissue Maldevelopment. J Atheroscler Thromb 2020;27:397-405. [Crossref] [PubMed]
- Metrustry SJ, Karhunen V, Edwards MH, et al. Metabolomic signatures of low birthweight: Pathways to insulin resistance and oxidative stress. PLoS One 2018;13:e0194316. [Crossref] [PubMed]
- Gray IP, Cooper PA, Cory BJ, et al. The intrauterine environment is a strong determinant of glucose tolerance during the neonatal period, even in prematurity. J Clin Endocrinol Metab 2002;87:4252-6. [Crossref] [PubMed]
- Wang X, Cui Y, Tong X, et al. Glucose and lipid metabolism in small-for-gestational-age infants at 72 hours of age. J Clin Endocrinol Metab 2007;92:681-4. [Crossref] [PubMed]
- Banister CE, Koestler DC, Maccani MA, et al. Infant growth restriction is associated with distinct patterns of DNA methylation in human placentas. Epigenetics 2011;6:920-7. [Crossref] [PubMed]
- Feng S, Jacobsen SE, Reik W. Epigenetic reprogramming in plant and animal development. Science 2010;330:622-7. [Crossref] [PubMed]
- Grozinger CM, Fan Y, Hoover SE, et al. Genome-wide analysis reveals differences in brain gene expression patterns associated with caste and reproductive status in honey bees (Apis mellifera). Mol Ecol 2007;16:4837-48. [Crossref] [PubMed]
- Shames DS, Minna JD, Gazdar AF. DNA methylation in health, disease, and cancer. Curr Mol Med 2007;7:85-102. [Crossref] [PubMed]
- Kumar A, Palfrey HA, Pathak R, et al. The metabolism and significance of homocysteine in nutrition and health. Nutr Metab (Lond) 2017;14:78. [Crossref] [PubMed]
- Yajnik CS, Deshpande SS, Jackson AA, et al. Vitamin B12 and folate concentrations during pregnancy and insulin resistance in the offspring: the Pune Maternal Nutrition Study. Diabetologia 2008;51:29-38. [Crossref] [PubMed]
- Regan FM, Cutfield WS, Jefferies C, et al. The impact of early nutrition in premature infants on later childhood insulin sensitivity and growth. Pediatrics 2006;118:1943-9. [Crossref] [PubMed]
- Hwang IT. Long-term care, from neonatal period to adulthood, of children born small for gestational age. Clin Pediatr Endocrinol 2019;28:97-103. [Crossref] [PubMed]
- Godfrey KM, Gluckman PD, Hanson MA. Developmental origins of metabolic disease: life course and intergenerational perspectives. Trends Endocrinol Metab 2010;21:199-205. [Crossref] [PubMed]
- Krishnaveni GV, Veena SR, Karat SC, et al. Association between maternal folate concentrations during pregnancy and insulin resistance in Indian children. Diabetologia 2014;57:110-21. [Crossref] [PubMed]
- Kumar KA, Lalitha A, Reddy U, et al. Chronic maternal vitamin B12 restriction induced changes in body composition & glucose metabolism in the Wistar rat offspring are partly correctable by rehabilitation. PLoS One 2014;9:e112991. [Crossref] [PubMed]
- Huang Y, He Y, Sun X, et al. Maternal high folic acid supplement promotes glucose intolerance and insulin resistance in male mouse offspring fed a high-fat diet. Int J Mol Sci 2014;15:6298-313. [Crossref] [PubMed]
- Girard CL, Vanacker N, Beaudet V, et al. Glucose and insulin responses to an intravenous glucose tolerance test administered to feed-restricted dairy cows receiving folic acid and vitamin B12 supplements. J Dairy Sci 2019;102:6226-34. [Crossref] [PubMed]
- Dehkordi EH, Sedehi M, Shahraki ZG, et al. Effect of folic acid on homocysteine and insulin resistance of overweight and obese children and adolescents. Adv Biomed Res 2016;5:88. [Crossref] [PubMed]
- Zhang Y, Wang G, Liu J, et al. Impact of hyperhomocysteinemia on insulin resistance in patients with H-type hypertension. Clin Exp Hypertens 2018;40:28-31. [Crossref] [PubMed]
- Li Z, Gueant-Rodriguez RM, Quilliot D, et al. Folate and vitamin B12 status is associated with insulin resistance and metabolic syndrome in morbid obesity. Clin Nutr 2018;37:1700-6. [Crossref] [PubMed]
- Setola E, Monti LD, Galluccio E, et al. Insulin resistance and endothelial function are improved after folate and vitamin B12 therapy in patients with metabolic syndrome: relationship between homocysteine levels and hyperinsulinemia. Eur J Endocrinol 2004;151:483-9. [Crossref] [PubMed]
- Santilli F, Davì G, Patrono C. Homocysteine, methylenetetrahydrofolate reductase, folate status and atherothrombosis: A mechanistic and clinical perspective. Vascul Pharmacol 2016;78:1-9. [Crossref] [PubMed]
- Kaur G, Gaur R, Yadav S, et al. Association of vitamin B12 mediated hyperhomocysteinemia and methylenetetrafolate reductase (C677T) gene polymorphism with cognitive impairment: A population based study from North India. Psychiatry Res 2018;270:123-5. [Crossref] [PubMed]
- Yeh EL, Huang YC, Tsai SF, et al. Relationship between plasma levels of homocysteine and the related B vitamins in patients with hemodialysis adequacy or inadequacy. Nutrition 2018;53:103-8. [Crossref] [PubMed]
- Quinlivan EP, McPartlin J, McNulty H, et al. Importance of both folic acid and vitamin B12 in reduction of risk of vascular disease. Lancet 2002;359:227-8. [Crossref] [PubMed]
- Satapathy S, Bandyopadhyay D, Patro BK, et al. Folic acid and vitamin B12 supplementation in subjects with type 2 diabetes mellitus: A multi-arm randomized controlled clinical trial. Complement Ther Med 2020;53:102526. [Crossref] [PubMed]
- Liu P, Huang J, Zhong L. Role and mechanism of homocysteine in affecting hepatic protein-tyrosine phosphatase 1B. Biochim Biophys Acta Gen Subj 2019;1863:941-9. [Crossref] [PubMed]
- Pouwels MJ, Den Heijer M, Blom HJ, et al. Improved insulin sensitivity and metabolic control in type 2 diabetes does not influence plasma homocysteine. Diabetes Care 2003;26:1637-9. [Crossref] [PubMed]
- Crott JW. Effects of altered parental folate and one-carbon nutrient status on offspring growth and metabolism. Mol Aspects Med 2017;53:28-35. [Crossref] [PubMed]