Apneic oxygenation with low-flow oxygen cannula for rapid sequence induction and intubation in pediatric patients: a randomized-controlled trial
Introduction
Rapid sequence induction and intubation (RSII) is defined as endotracheal intubation that is performed as soon as possible without face mask ventilation to reduce the risk of pulmonary aspiration. RSII was introduced in the 1960s and has become a standard practice in patients suspected to be at high-risk for gastric regurgitation, including inadequate fasting time, delayed gastric emptying time, or increased intra-abdominal pressure (1). During the 2010s, the incidence of pulmonary aspiration decreased to 2.2–6.0 per 10,000 cases in emergency surgery and 1.4–2.0 per 10,000 cases in elective surgery, respectively (2,3). The incidence of pulmonary aspiration was reported as high as 8% in the emergency department (ED) setting (4). Walker et al. reported 24 cases with pulmonary aspiration after 118,371 anesthesia; none of the aspirations occurred after RSII (3). Gencorelli et al. explored 1,070 patients at high-risk for pulmonary aspiration who received RSII and reported no pulmonary aspiration (5). Furthermore, RSII also improved the first-attempt success rate in the ED and intensive care unit (6,7).
The RSII protocol avoids positive pressure ventilation to prevent regurgitation of gastric contents; however, this puts the patient at risk for hypoxemia (5). The incidence of hypoxemia after classical RSII in the operating room (OR), ED, and intensive care unit (ICU) was reported to range from 7–25% in adults (8), from 14–33% in pediatric patients (9-12), and from 30–59% in pediatric patients aged <2 years (13,14). The one exception was reported by Gencorelli et al. who found an incidence of hypoxemia of 3.6% in the pediatric OR (5).
Apneic oxygenation (ApOx) refers to the passive movement of oxygen to the alveoli in the absence of lung movement by patient effort or positive pressure ventilation (8,15-17). This technique has increased in popularity over the last ten years due to its ability to reduce desaturation during intubation in the ED and ICU, and in healthy patients undergoing elective surgery in adult studies (8,18,19). This technique has also been implemented in obesity, obstetrics, and pediatrics (16,20). In a small number of pediatric studies in a non-RSII condition, ApOx could prolong safe apneic time and decrease the incidence of hypoxemia (21-24).
ApOx techniques include (I) humidified high-flow nasal oxygenation system; (II) traditional low-flow nasal cannula; (III) modified nasopharyngeal airway; (IV) oxygen catheter in pharynx; and (V) laryngoscope side-port (16,17). In this study, we decided to investigate the effectiveness of low-flow nasal cannula during RSII due to its wide availability, affordability, and ease of use. The aim of this study was to examine the effect of ApOx using low-flow nasal cannula during RSII. The primary outcome was the incidence of hypoxemia, defined as oxygen saturation (SpO2) ≤92%. The secondary outcomes were the time to desaturation during RSII, the lowest SpO2, and complications of RSII. We present the following article in accordance with the CONSORT reporting checklist (available at https://tp.amegroups.com/article/view/10.21037/tp-21-484/rc).
Methods
This study was a single center, single-blind, randomized controlled study, conducted at the Faculty of Medicine Siriraj Hospital, Mahidol University, Bangkok, Thailand during February 2020 to March 2021. Siriraj Hospital is the university-based national tertiary referral center for both adult and pediatric referrals. The study was conducted in accordance with the Declaration of Helsinki (as revised in 2013). The study protocol was approved by the Siriraj Institutional Review Board (Si 059/2020), and was registered at thaiclinicaltrials.org (TCTR20210802002). Written informed consent was taken from the parent(s) or legal guardian(s) of all included participants.
Population
Neonates, infants, and children aged up to 7 years with American Society of Anesthesiologists (ASA) physical status 1 to 3 who were scheduled for elective or emergency surgery under general anesthesia with RSII were included. The indication for RSII was determined by the attending anesthesiologist. The exclusion criteria comprised history of heart disease or respiratory disease, expected difficult airway management, fever (body temperature >37.5 ℃), having received preoperative oxygen supplementation, and/or allergy to any study drug. Participants who experienced inadvertent mask ventilation, postinduction hypotension, unexpected difficult airway, gastric content regurgitation, or critical cardiovascular disturbance were withdrawn from the study. Participants with esophageal intubation were also excluded from the analysis.
Study protocol
Study subjects were randomized to either the ApOx group or the classical group (1:1 ratio allocation) using computer-generated block size of four randomization by an independent statistician. The random allocation sequence was kept separately in sealed opaque envelopes with sequential numbers. PS opened the envelope containing each patient group assignment before induction of anesthesia, then assigned participants to interventions. Anesthetic personnel in the OR could not be blinded because a nasal cannula was used for the intervention. As such, only the patients and parents were blinded to the group allocation.
Upon arrival in the OR, standard monitoring was applied prior to induction. All participants were premedicated with midazolam 0.1 mg/kg and fentanyl 1 mcg/kg intravenously. When mask acceptance was expected, awake preoxygenation was administered with 100% oxygen 6 liters per minute (LPM) using a tight-fitting facemask connected to the circle circuit of the anesthetic machine for 3 minutes. Rapid sequence induction of anesthesia was conducted with thiopental 5 mg/kg followed by either succinylcholine 1.5 mg/kg or rocuronium 1.2 mg/kg. The choice of rapid-onset muscle relaxant was according to anesthesiologist discretion. After induction, asleep oxygenation was administered with jaw thrust maneuver and 100% oxygen 6 LPM via tight-fitting facemask for 1 minute, by which time muscle relaxation was expected to be fully achieved. No cricoid pressure was applied in this study.
Intervention allocation
In the ApOx group, a nasal cannula with no oxygen flow was applied prior to awake preoxygenation with facemask. ApOx was initiated at the end of asleep oxygenation. The oxygen flow rate was administered according to World Health Organization (WHO) recommendation for maximum flow rate via nasal cannula, as follows: 1 LPM in age 0–1 month, 2 LPM in age 1–12 months, and 4 LPM in age 1–7 years (25). In the classical group, a nasal cannula was not applied, and intubation was performed without ApOx. SpO2 was focused upon until reaching the primary endpoint. The primary endpoint was defined as hypoxemia (SpO2 decreased to 92%) or successful intubation. Study participants were intubated by pediatric anesthesia fellows or senior anesthesia residents.
In case of desaturation, the procedure was discontinued as soon as the SpO2 reached 92%. Rescue procedure comprised jaw thrust and positive pressure ventilation with pressure below 20 cmH2O via facemask. Intubation was then reperformed after the SpO2 increased to 100%. The lowest SpO2 was recorded as the secondary endpoint in patients with desaturation. Timing of intervention is described in Figure 1.
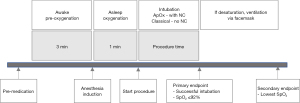
Data collection
Demographic and clinical data that were collected included age, weight, height, gender, indication for RSII, and baseline SpO2. Adequate preoxygenation was defined as patient cooperation with breathing via tight-fitting facemask for 3 minutes that facilitated successful capnographic monitoring. The primary outcome was the incidence of hypoxemia, defined as SpO2 ≤92%. The secondary outcomes were the time to desaturation during RSII, the lowest SpO2, and complications of RSII. Procedure time was defined as the duration from the end of asleep oxygenation until achievement of the primary endpoint. If primary endpoint was hypoxemia, procedure time was called time to desaturation. If primary endpoint was successful intubation, procedure time was called time to successful intubation. Complication was evaluated after successful intubation, including bradycardia, hypotension, airway injury, and pulmonary aspiration. Sign of regurgitation of gastric content was recorded.
Sample size calculation
The sample size was calculated using nQuery Advisor® version 6.0 (Statistical Solutions Limited; GraphPad Software, Inc., San Diego, CA, USA). Soneru et al. applied ApOx with nasal cannula 5 LPM in children aged 0–8 years and reported an incidence of desaturation (SpO2<95%) in the ApOx and control groups of 9% and 43%, respectively (21). Using this data, we estimated that the incidence of hypoxemia in the present study (SpO2 ≤92%) would be 10% and 40% in the ApOx and classical groups, respectively. Using a type I error of 0.05 and a power of 80%, the calculated sample size was 32 participants in each arm.
Statistical analysis
Statistical analysis was performed using SPSS Statistics version 21 (SPSS, Inc.; IBM Corporation, Armonk, NY, USA). Demographic and clinical data are reported using descriptive statistics. Continuous variables are reported as mean plus/minus standard deviation for normally distributed data (Student’s t-test), and as median and interquartile range for non-normally distributed data (Mann-Whitney U test). Categorical variables are reported as number and percentage (chi-square test or Fisher’s exact test). Incidence of desaturation, time to primary endpoint, and lowest SpO2 were compared between two groups. Survival analysis for time to the primary endpoint is described by Kaplan-Meier curve with log-rank test. Post hoc analysis was performed in patients considered to be at high risk for desaturation, including infants, those with abdominal distension, and procedure time longer than 30 seconds. A P value (2-sided) less than 0.05 was considered to be statistically significant.
Results
From February 2020 to March 2021, 64 pediatric participants were included in the study—32 in the ApOx group, and 32 in the classical group. All subjects received their allocated intervention, completed the follow-up, and were included in the final analysis by original assigned groups. A CONSORT flow diagram is shown in Figure 2. Patient characteristics, all of which were not significantly different between groups, are summarized in Table 1. Adequate preoxygenation was achieved in 30 (93.8%) and 29 (90.6%) participants in ApOx and classical groups, respectively (P=1.000).
Table 1
Characteristics | Apneic oxygenation (n=32) | Classical (n=32) |
---|---|---|
Age (months), median (P25–P75) | 12.5 (2.0–37.3) | 16.0 (3.3–59.0) |
Age group, n (%) | ||
Neonate | 7 (21.9) | 6 (18.8) |
Infant | 9 (28.1) | 8 (25.0) |
1–7 years | 16 (50.0) | 18 (56.2) |
Weight (kg), mean ± SD | 10.0±6.6 | 9.4±5.3 |
Height (cm), mean ± SD | 75.7±21.4 | 76.3±25.8 |
Male gender, n (%) | 17 (53.1) | 18 (56.3) |
ASA physical status, n (%) | ||
1E | 1 (3.1) | 2 (6.2) |
2 | 13 (40.6) | 12 (37.5) |
2E | 1 (3.1) | 3 (9.4) |
3 | 9 (28.2) | 9 (28.2) |
3E | 8 (25.0) | 6 (18.7) |
Emergency, n (%) | 10 (31.3) | 11 (34.4) |
Indication, n (%) | ||
Abdominal distension | 17 (53.1) | 16 (50.0) |
Gastroesophageal reflux or full stomach | 15 (46.9) | 16 (50.0) |
Type of surgery, n (%) | ||
Major abdominal | 20 (62.5) | 16 (50.0) |
Thoracotomy | 0 (0.0) | 2 (6.3) |
Gastrointestinal endoscopy | 9 (28.1) | 9 (28.1) |
Minor procedure | 3 (9.4) | 5 (15.6) |
Baseline O2 saturation (%), mean ± SD | 99.4±1.4 | 99.9±0.5 |
SD, standard deviation; ASA, American Society of Anesthesiologists; O2, oxygen.
There was no significant difference in the incidence of hypoxemia (SpO2 ≤92%) between the ApOx and classical groups. Eight participants (25%) in each group experienced desaturation (P=1.000). The incidence of desaturation below 90%, 88%, and 85% also showed no statistically significant difference between groups. Table 2 reports data specific to the primary and secondary endpoints between groups. In desaturated patients, the median (P25–P75) time to desaturation was 29.5 (20.3–54.0) and 35.0 (22.8–60.0) seconds in the ApOx and classical groups, respectively (P=0.527). The median (P25–P75) lowest SpO2 was 91.0% (88.0–92.0)% and 88.5% (83.5–89.5)% in the ApOx and classical groups, respectively (P=0.079). In non-desaturated patients, the median (P25–P75) time to successful intubation was 40.5 (32.3–50.0) and 35.5 (28.5–41.8) seconds in the ApOx and classical groups, respectively (P=0.069.) The lowest SpO2 compared between groups is shown in Figure 3.
Table 2
Outcomes | Apneic oxygenation (n=32) | Classical (n=32) | P |
---|---|---|---|
Incidence of desaturation ≤92%, n (%) | 8 (25.0) | 8 (25.0) | 1.000 |
Incidence of desaturation ≤90%, n (%) | 4 (12.5) | 7 (21.9) | 0.320 |
Incidence of desaturation ≤88%, n (%) | 3 (9.4) | 4 (12.5) | 1.000 |
Incidence of desaturation ≤85%, n (%) | 0 (0.0) | 3 (9.4) | 0.238 |
Desaturated patient | (n=8) | (n=8) | |
Time to desaturation (s), median (P25–P75) | 29.5 (20.3–54.0) | 35.0 (22.8–60.0) | 0.527 |
Lowest SpO2 (%), median (P25–P75) | 91.0 (88.0–92.0) | 88.5 (83.5–89.5) | 0.079 |
Non-desaturated patient | (n=24) | (n=24) | |
Time to successful intubation (s), median (P25–P75) | 40.5 (32.3–50.0) | 35.5 (28.5–41.8) | 0.069 |
Lowest SpO2 prior to intubation (%), median (P25–P75) | 100 (98.3–100) | 100 (99.25–100) | 0.580 |
A P value (2-sided) <0.05 indicates statistical significance. SpO2, oxygen saturation.
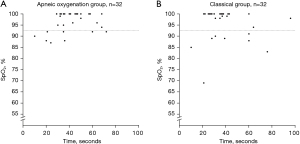
Survival analysis of patients maintaining SpO2 >92% compared between the ApOx and classical groups is described by Kaplan-Meier curve in Figure 4 (P=0.753). Cases of successful intubation before this threshold was met were censored.
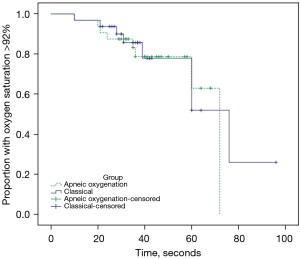
The primary outcome was reexamined in post hoc analysis for subgroup population. We explored infants, participants with abdominal distension, and procedure time ≥30 seconds because these subgroups are at theoretically higher risk for desaturation. However, post hoc analysis revealed no differences in the incidence of desaturation. There were 30 infants (16 in the ApOx group, and 14 in classical group). The incidence of desaturation was 4 (25.0%) and 2 (14.3%) patients, respectively (P=0.657). There were 33 participants with abdominal distension (17 in ApOx group, and 16 in classical group). The incidence of desaturation was 6 (35.3%) and 3 (18.8%) patients, respectively (P=0.438). There were 29 participants who had a procedure time ≥30 seconds (26 in ApOx group, and 23 in classical group). The incidence of desaturation was 4 (15.4%) and 5 (21.7%) patients, respectively (P=0.716). The calculated post-hoc statistical power for infants, participants with abdominal distension, and procedure time ≥30 seconds were 10.4%, 17.9%, and 8.3%, respectively.
No complications from RSII, including post-induction hypotension, bradycardia requiring atropine, unexpected difficult airway, airway injury, esophageal intubation, or gastric content regurgitation, was observed in this study. Complication from the nasal cannula in ApOx group, including epistaxis and skin irritation, was observed. None of the complication was found in this study.
Discussion
ApOx with low-flow oxygen cannula did not significantly reduce the incidence of oxygen desaturation ≤92% after RSII in a pediatric anesthesia setting. However, there was a non-statistically significant trend toward a reduced incidence of hypoxemia less than 90%, 88%, and 85% in the ApOx group (Table 2). This finding compares to that from a meta-analysis of adult studies (8,18,19), that found that ApOx decreased the incidence of hypoxemia despite high study heterogeneity relative to regular low-flow nasal cannula and humidified high-flow nasal cannula. Holyoak et al. reported that nasal cannula with oxygen flow ≥15 LPM reduced the incidence of desaturation (18). White et al. reported an insufficient amount of data to support any specific flow rate for regular nasal cannula in adults (19).
The oxygen flow rate used in this pediatric study was according to WHO recommendation and as follows (25): 1 LPM in age 0–1 month, 2 LPM in age 1–12 months, and 4 LPM in age 1–7 years, all of which are lower than the flow rates used in other studies. Soneru et al. studied nasal cannula 5 LPM in the OR (21), and Napolitano et al. studied nasal cannula 5 LPM in age <12 months, 10 LPM in age 1–7 years, and 15 LPM in age ≥8 years (NEAR4KIDS consensus) in the pediatric intensive care unit (PICU) (22). ApOx in both studies was shown to prolong safe apneic time and decrease the incidence of hypoxemia in a non-RSII setting. Vukovic et al. studied nasal cannula 4 LPM in age 0–2 years, 6 LPM in age 2–12 years, and 8 LPM in age >12 years in an ED setting (26). They reported differences in the lowest SpO2 between the ApOx and classical groups. Riva et al. compared safe apneic time between low-flow O2 0.2 L/kg/min, THRIVE 100% O2 2 L/kg/min, and THRIVE 30% O2 2 L/kg/min [transnasal humidified rapid-insufflation ventilatory exchange (THRIVE)] (24). They found that THRIVE flow did not extend safe apneic time compared to low flow. Other studies that studied low-flow oxygen cannula are shown in Table 3 (12,21-24,26-29). Future physiologic studies are needed to determine the oxygen flow rate in children that is equivalent to nasal cannula 15 LPM in adults, or the minimal flow required to meet metabolic demand. Even though non-humidified oxygen flow higher than the WHO recommendation might lead to dry airway and reduced patient comfort, the duration of ApOx is brief and the patient is unconscious. However, this risk could outweigh the benefit of reducing the incidence of hypoxemia during intubation.
Table 3
Study | Type | Setting | RSII | Number of patients | Intervention | Age (years) | Outcome |
---|---|---|---|---|---|---|---|
Olayan et al. 2018, (27) | RCT | OR | Yes | 30 | ApOx vs. no oxygen; NC 3 LPM |
1–8 | No significant difference for time to first event (desaturation or successful intubation). Difference in lowest SpO2 between two groups (ApOx 100%, no O2 99%, P=0.004) |
Overmann et al. 2019, (12) | Retrospective cohort | ED (pre-post) | Yes | 305 | ApOx vs. no oxygen; NC <3 y 2 LPM, 3–8 y 4 LPM, >8 y 6 LPM |
0–18 | No difference in incidence of desaturation <90% (ApOx 22%, no O2 14%) |
Vukovic et al. 2019, (26) | Prospective cohort | ED (pre-post) | Yes/no | 149 | ApOx vs. no oxygen; NC up to 2 y 4 LPM, >2 to ≤12 y 6 LPM, >12 y 8 LPM |
0–22 | Difference in lowest SpO2 (ApOx 100%, no O2 93%, P<0.001) |
Mortimer et al. 2016, (28) | Prospective observational | ED, PICU | Yes | 44 | ApOx only; NC <4 y 5 LPM, 4–12 y 10 LPM, 12–18 y 15 LPM |
0–18 | Incidence of desaturation <80% = 23% in aged <4 years, 0 in aged 4–12 years, and 8% in aged 12–18 years |
Ayanmanesh et al. 2021, (29) | Prospective observational | OR | Yes | 79 | ApOx only; THRIVE 2 L/kg/min |
1–15 | Incidence of desaturation <95% = 11.4% |
Soneru et al. 2019, (21) | Prospective cohort | OR (pre-post) | No | 356 | ApOx vs. no oxygen; NC 5 LPM |
0–8 | NC extend safe apneic time and decrease incidence of desaturation <95% (ApOx 9%, no O2 43%, P<0.001) |
Napolitano et al. 2019, (22) | Prospective cohort | PICU (pre-post) | No | 1373 | ApOx vs. no oxygen; NC <12 m 5 LPM, 1–7 y 10 LPM, ≥8 y 15 LPM |
0–18 | ApOx decreased incidence of moderate desaturation <80% (ApOx 15.4%, no O2 11.8%, P=0.049) |
Humphreys et al. 2017, (23) | RCT | OR | No | 48 | ApOx vs. no oxygen; THRIVE 0–15 kg 2 L/kg/min, 15–30 kg 35 LPM, 30–50 kg 40 LPM, >50 kg 50 LPM |
0–10 | THRIVE prolonged apneic time |
Riva et al. 2018, (24) | RCT | OR | No | 60 | ApOx only; (I) low-flow NC 0.2 L/kg/min, (II) THRIVE 100% O2 2 L/kg/min, (III) THRIVE 30% O2 2 L/kg/min |
0–8 | THRIVE flow did not extend safe apneic time compared with low flow |
ApOx, apneic oxygenation; RSII, rapid sequence induction and intubation; RCT, randomized controlled trial; OR, operating room; ED, emergency department; PICU, pediatric intensive care unit; NC, nasal cannula; LPM, liters per minute; THRIVE, transnasal humidified rapid-insufflation ventilatory exchange; SpO2, oxygen saturation.
The induction technique used in this study was RSII, which is not normally used in elective surgery. Previous studies that reported that ApOx, both high-flow and low-flow, could prolong safe apneic time were all conducted in non-RSII settings (21-24). The patients in those studies normally received preoxygenation via positive pressure ventilation to maximize oxygen concentration in the pulmonary alveoli prior to apnea. Positive pressure ventilation is contraindicated during classical RSII. In contrast, none of the previous randomized controlled trials in pediatric RSII demonstrated that ApOx could improve outcome compared to classical RSII (12,27). After anesthesia induction in our study, asleep oxygenation was applied while the patient became hypoventilated due to anesthetic agents until apnea. The effectiveness of asleep oxygenation is obviously less than that of preoxygenation with positive pressure ventilation prior to apnea in elective surgery. We hypothesize that different technique of preoxygenation and different oxygen reserve prior to apnea during general anesthesia induction in elective surgery versus RSII led to the ineffectiveness of ApOx during the RSII procedure.
This study measured time to desaturation or time to successful intubation. Due to the nature of the RSII technique, we were unable to measure the specific duration of safe apneic time, which is similar to other studies. Our patients must have become apneic at some point within 1 minute of asleep oxygenation, and we were unable to allow the patient to desaturate and then ventilate to measure accurate safe apneic time. Intubation was performed by experienced clinician (senior residents or pediatric anesthesia fellow) to decrease the risk of hypoxemia. The intubation time (mostly within 60 seconds; Table 2) was generally shorter compared to other studies in elective surgery. Patients who were successfully intubated would not experience hypoxemia, so the safe apneic time could not be measured.
Limitations
This study has some mentionable limitations. First, the sample size was calculated from ApOx with low-flow oxygen cannula in elective pediatric surgery, which could have an incidence of hypoxemia different from that of RSII. The sample size calculation did not consider the lowest SpO2 which was the secondary outcome. Second, since this study was conducted in a national tertiary referral center, more than half of study participants had intra-abdominal pathology that could affect functional residual capacity and oxygen reserve. Therefore, our result cannot be generalized to other pediatrics requiring RSII due to inadequate fasting time. Third, the end-tidal oxygen concentration was not available on every anesthetic machine, so the efficacy of preoxygenation could not be objectively measured. Fourth, nadir SpO2 was collected only in patients with desaturation ≤92%. Therefore, in patients who were intubated without hypoxemia, but who then developed late desaturation after intubation would not have been identified and documented. Fifth and last, we did not collect data specific to apneic ventilation since this parameter is not reported in low-flow ApOx.
Conclusions
ApOx using low-flow oxygen cannula did not decrease the incidence of hypoxemia in pediatric patients undergoing RSII. High-quality pediatric studies in ApOx during RSII are limited. Future studies in the appropriate flow for low-flow nasal cannula, the efficacy of ApOx (both low-flow and humidified high-flow oxygen cannula) in an RSII setting among pediatric patients at high-risk for pulmonary aspiration that could not be effectively ventilated prior to apnea, and the related complications of these techniques are needed.
Acknowledgments
The authors gratefully acknowledge the children and their parents for agreeing to participate in this study. The authors would also like to thank Chulaluk Komoltri, PhD for her assistance with statistical analysis, and Miss Arporn Pimtong for administrative support. English language was edited by Kevin P. Jones.
Funding: This work was supported by a grant from the Siriraj Research Development Fund of the Faculty of Medicine Siriraj Hospital, Mahidol University, Bangkok, Thailand (grant number [IO] R016331029).
Footnote
Reporting Checklist: The authors have completed the CONSORT reporting checklist. Available at https://tp.amegroups.com/article/view/10.21037/tp-21-484/rc
Trial Protocol: Available at https://tp.amegroups.com/article/view/10.21037/tp-21-484/tp
Data Sharing Statement: Available at https://tp.amegroups.com/article/view/10.21037/tp-21-484/dss
Conflicts of Interest: All authors have completed the ICMJE uniform disclosure form (available at https://tp.amegroups.com/article/view/10.21037/tp-21-484/coif). The authors have no conflicts of interest to declare.
Ethical Statement: The authors are accountable for all aspects of the work in ensuring that questions related to the accuracy or integrity of any part of the work are appropriately investigated and resolved. The study was conducted in accordance with the Declaration of Helsinki (as revised in 2013). The study was approved by the Siriraj Institutional Review Board (SIRB) (COA No. 059/2020), and informed consent was taken from the parent(s) or legal guardian(s) of all included participants.
Open Access Statement: This is an Open Access article distributed in accordance with the Creative Commons Attribution-NonCommercial-NoDerivs 4.0 International License (CC BY-NC-ND 4.0), which permits the non-commercial replication and distribution of the article with the strict proviso that no changes or edits are made and the original work is properly cited (including links to both the formal publication through the relevant DOI and the license). See: https://creativecommons.org/licenses/by-nc-nd/4.0/.
References
- Sinclair RCF, Luxton MC. Rapid sequence induction. Continuing Education in Anaesthesia Critical Care & Pain 2005;5:45-8. [Crossref]
- Tan Z, Lee SY. Pulmonary aspiration under GA: a 13-year audit in a tertiary pediatric unit. Paediatr Anaesth 2016;26:547-52. [Crossref] [PubMed]
- Walker RW. Pulmonary aspiration in pediatric anesthetic practice in the UK: a prospective survey of specialist pediatric centers over a one-year period. Paediatr Anaesth 2013;23:702-11. [Crossref] [PubMed]
- Driver BE, Klein LR, Schick AL, et al. The occurrence of aspiration pneumonia after emergency endotracheal intubation. Am J Emerg Med 2018;36:193-6. [Crossref] [PubMed]
- Gencorelli FJ, Fields RG, Litman RS. Complications during rapid sequence induction of general anesthesia in children: a benchmark study. Paediatr Anaesth 2010;20:421-4. [Crossref] [PubMed]
- Mosier JM, Sakles JC, Stolz U, et al. Neuromuscular blockade improves first-attempt success for intubation in the intensive care unit. A propensity matched analysis. Ann Am Thorac Soc 2015;12:734-41. [Crossref] [PubMed]
- Sagarin MJ, Barton ED, Chng YM, et al. Airway management by US and Canadian emergency medicine residents: a multicenter analysis of more than 6,000 endotracheal intubation attempts. Ann Emerg Med 2005;46:328-36. [Crossref] [PubMed]
- Tan E, Loubani O, Kureshi N, et al. Does apneic oxygenation prevent desaturation during emergency airway management? A systematic review and meta-analysis. Can J Anaesth 2018;65:936-49. [Crossref] [PubMed]
- Kemper ME, Buehler PK, Schmitz A, et al. Classical versus controlled rapid sequence induction and intubation in children with bleeding tonsils (a retrospective audit). Acta Anaesthesiol Scand 2020;64:41-7. [Crossref] [PubMed]
- Sun J, Li XH, Zuo YX. Comparison of Incidence of hypoxia during modified rapid sequence induction and an alternative technique: a prospective randomized controlled trial. Int J Clin Exp Med 2015;8:16231-7. [PubMed]
- Kerrey BT, Rinderknecht AS, Geis GL, et al. Rapid sequence intubation for pediatric emergency patients: higher frequency of failed attempts and adverse effects found by video review. Ann Emerg Med 2012;60:251-9. [Crossref] [PubMed]
- Overmann KM, Boyd SD, Zhang Y, et al. Apneic oxygenation to prevent oxyhemoglobin desaturation during rapid sequence intubation in a pediatric emergency department. Am J Emerg Med 2019;37:1416-21. [Crossref] [PubMed]
- Park RS, Rattana-Arpa S, Peyton JM, et al. Risk of Hypoxemia by Induction Technique Among Infants and Neonates Undergoing Pyloromyotomy. Anesth Analg 2021;132:367-73. [Crossref] [PubMed]
- Rinderknecht AS, Mittiga MR, Meinzen-Derr J, et al. Factors associated with oxyhemoglobin desaturation during rapid sequence intubation in a pediatric emergency department: findings from multivariable analyses of video review data. Acad Emerg Med 2015;22:431-40. [Crossref] [PubMed]
- Lyons C, Callaghan M. Uses and mechanisms of apnoeic oxygenation: a narrative review. Anaesthesia 2019;74:497-507. [Crossref] [PubMed]
- Lyons C, Callaghan M. Apnoeic oxygenation in paediatric anaesthesia: a narrative review. Anaesthesia 2021;76:118-27. [Crossref] [PubMed]
- Else SDN, Kovatsis PG. A Narrative Review of Oxygenation During Pediatric Intubation and Airway Procedures. Anesth Analg 2020;130:831-40. [Crossref] [PubMed]
- Holyoak RS, Melhuish TM, Vlok R, et al. Intubation using apnoeic oxygenation to prevent desaturation: A systematic review and meta-analysis. J Crit Care 2017;41:42-8. [Crossref] [PubMed]
- White LD, Melhuish TM, White LK, et al. Apnoeic oxygenation during intubation: a systematic review and meta-analysis. Anaesth Intensive Care 2017;45:21-7. [Crossref] [PubMed]
- Scott A, Chua O, Mitchell W, et al. Apneic Oxygenation for Pediatric Endotracheal Intubation: A Narrative Review. J Pediatr Intensive Care 2019;8:117-21. [Crossref] [PubMed]
- Soneru CN, Hurt HF, Petersen TR, et al. Apneic nasal oxygenation and safe apnea time during pediatric intubations by learners. Paediatr Anaesth 2019;29:628-34. [Crossref] [PubMed]
- Napolitano N, Laverriere EK, Craig N, et al. Apneic Oxygenation As a Quality Improvement Intervention in an Academic PICU. Pediatr Crit Care Med 2019;20:e531-7. [Crossref] [PubMed]
- Humphreys S, Lee-Archer P, Reyne G, et al. Transnasal humidified rapid-insufflation ventilatory exchange (THRIVE) in children: a randomized controlled trial. Br J Anaesth 2017;118:232-8. [Crossref] [PubMed]
- Riva T, Pedersen TH, Seiler S, et al. Transnasal humidified rapid insufflation ventilatory exchange for oxygenation of children during apnoea: a prospective randomised controlled trial. Br J Anaesth 2018;120:592-9. [Crossref] [PubMed]
- World Health Organization. Oxygen therapy for children: a manual for health workers. Geneva: World Health Organization; 2016.
- Vukovic AA, Hanson HR, Murphy SL, et al. Apneic oxygenation reduces hypoxemia during endotracheal intubation in the pediatric emergency department. Am J Emerg Med 2019;37:27-32. [Crossref] [PubMed]
- Olayan L, Alatassi A, Patel J, et al. Apnoeic oxygenation by nasal cannula during airway management in children undergoing general anaesthesia: a pilot randomised controlled trial. Perioper Med (Lond) 2018;7:3. [Crossref] [PubMed]
- Mortimer T, Burzynski J, Kesselman M, et al. Apneic Oxygenation during Rapid Sequence Intubation in Critically Ill Children. J Pediatr Intensive Care 2016;5:28-31. [Crossref] [PubMed]
- Ayanmanesh F, Abdat R, Jurine A, et al. Transnasal humidified rapid-insufflation ventilatory exchange during rapid sequence induction in children. Anaesth Crit Care Pain Med 2021;40:100817. [Crossref] [PubMed]