Cross-sectional association between physical fitness and cardiometabolic risk in Chilean schoolchildren: the fat but fit paradox
Introduction
Excess weight (overweight and obesity) is a cardiovascular risk factor commonly found in childhood and adult. However, scientific evidence has recently pointed out that these risk factors can be also found in early years (1,2). Excess weight in Chilean children has tripled in recent decades, reaching 23.9% in 2017 (3). Obesity is linked with a high incidence fasting insulin levels (42.0% of all children with obesity have atypical values) and hypercholesterolemia (27.0%) (4,5). Childhood obesity is also related to a higher risk of cardiovascular disease in later in life (6).
Cardiometabolic risk (CMR) score (computed by summing several risk factors) is recognized as a suitable tool for establishing CMR in children (7). CMR is higher with clustering of risk factors such as abnormal cholesterol, hypertension, hypertriglyceridemia, abdominal obesity, in young people (8). This coexistence of these risk variables has been related to increased risk of cardiovascular disease and type 2 diabetes (known as metabolic syndrome) (9). Since CMR clustering in children is linked with higher odds of metabolic syndrome in adulthood (10), efforts are needed to define and quantify the risk of metabolic syndrome in children (11). In this sense, unhealthy dietary patterns and a lack of regular physical activity at moderate-to-vigorous (12) are essential contributors to the early onset of CMR. Sixty percent of the world’s population does not engage regular physical activity as recommended to prevent noncommunicable diseases (13,14). More specifically, Chilean children are predominantly insufficiently active, given that only 1 out of 5 meets with the physical activity recommendations (15).
Physical fitness is considered a powerful marker of health (16), and its evaluations are feasible measures that can contribute to improved follow-up on pediatric health management (17). The independent biological influence of cardiorespiratory fitness (CRF) and muscular fitness (MF) on CMR has been verified in children and adolescents (18,19). Similarly, a meta-analysis by Ruiz et al. (20) found a robust association between CRF and CMR factors in young people, providing cut-points that more accurately define cardiovascular disease risk. The combined effect of both CRF and MF and its interdependence has been less studied. For example, Artero et al. (21) found that MF was negatively associated with CMR, irrespective of CRF. Furthermore, these same authors found an inverse association between CRF clustered metabolic risk, regardless of MF. Moreover, Steene-Johannessen et al. (22) reported that in 2,818 children aged 9–15 years, MF was negatively associated with clustered CMR, regardless of CRF. Despite these benefits, a recent systematic review by Fühner et al. (23) found negative secular trends for physical fitness (e.g., CRF, MF), independently of sex (from 1972 to 2015).
The “fat but fit” paradox refers to those individuals whom in spite of having obesity show a relatively high physical fitness level (24). This paradigm suggests that individuals with both obesity and moderate-to-high CRF do not have a significantly greater risk of mortality from cardiovascular disease than normal weight with low CRF (in adults) (25). In young population, evidence with regards to this paradox is not consistent (25-28). Previous studies have examined the “fat but fit” paradox, revealing that greater levels of physical fitness may diminish the harmful consequences of excess weight on CMR risk (28-30). Despite the above, specific data about the “fat but fit” paradox in prepuberal population is rare (25). However, scientific evidence has indicated that having moderate-to-high CRF levels may mitigate the detrimental metabolic consequences associated with excessive adiposity (both total and central) (31,32). Similarly, physical fitness data (e.g., CRF) has been required from children in low-income and middle- income countries (e.g., Chile) to determine if temporal trends are similar to those found in high-income and upper middle-income countries (33).
Based on the above, the aim of this study was to determine the relationship between CMR across (individual and combined) physical fitness and excess weight status and whether the “fat but fit” paradox is met in schoolchildren from Santiago (Chile). We present the following article in accordance with the STROBE reporting checklist (available at https://tp.amegroups.com/article/view/10.21037/tp-22-25/rc).
Methods
Design and participants
This is a cross-sectional study including children of the “Growth and Obesity Chilean Cohort Study”, which assessed the association of early growth and development with adiposity and metabolic risk (34). To perform this study, 452 healthy Chilean children (185 boys and 267 girls) aged 7 to 9 years were randomly selected from original study. The inclusion criteria of the study were as follows: (I) children attending Chilean National Nursery School Council Program nursery schools from the south area of Santiago (Chile); (II) singletons; (III) birth weight ≥2,500 grams (data retrieved from medical archives); (IV) gestational age 37–42 weeks; (V) no psychological or physical conditions that may severely influence on growth; and (VI) blood data available.
The final sample size included 452 schoolchildren (59.1% girls) aged 7–9 years. The study was conducted in accordance with the Declaration of Helsinki (as revised in 2013). The study was approved by the Institute of Nutrition and Food Technology Ethics Committee for research in human subjects institutional and/or national research (Act No. 19, 2009). Parents or legal guardians of all participants signed an informed consent form before participation.
Procedures
Anthropometric measurements and pubertal status
Weight was measured in underclothing with an electronic scale (0.1 kg of accuracy) by a body composition analyzer (TANITA BC-418, Tokyo, Japan). Height was measured with a portable stadiometer (SECA 222®), with a 1-mm precision using the Frankfurt standard (35). Body mass index (BMI) was determined by dividing body weight (in kg) by height (in meters squared). Subsequently, the World Health Organization criteria for BMI were used to compute BMI z-score and the prevalence of excess weight (overweight and obesity) was calculated (36). Thus, schoolchildren were divided into: participants with excess weight (high BMI) and participants without excess weight (low BMI). Waist circumference (WC) was measured with a flexible tape (SECA®) (37). All variables were measured twice for each child. The measurements agreed within 0.5 cm for height and WC and 20 g for weight. In addition, waist-to-height ratio (WHtR) was computed by dividing WC by height.
Pubertal status was assessed using the method described by Tanner and Whitehouse (38).
Blood extractions
Blood samples were collected from 08:30 to 10:30 after an 8-h overnight fast. Glucose, insulin and blood lipids were calculated after 8 h of fasting. Ten milliliters of venous blood were gathered, and serum glucose was determined using a commercial kit by the GOD-PAP (Glucose GOD/PAP, Roche Diagnostics, Basel, Switzerland) enzymatic colorimetric method. Insulin was measured by RIA (RIA DCP Diagnostic Products Corporation, LA, USA). Glucose impaired was defined by a fasting glucose ≥100 mg/dL (39). Basal insulin sensitivity was estimated by homeostatic model assessment for insulin resistance (HOMA-IR) [fasting insulin (mIU/dL) * fasting glucose (mmol/L)/22.5] (40). Hyperinsulinemia was defined as baseline insulin ≥10 mIU/dL in children with Tanner I or II (41). Insulin resistance was diagnosed if HOMA-IR ≥2.1, which corresponds to >75th percentile of the observed quartile in Chilean children (42). Cholesterol, high-density lipoprotein (HDL) and triglycerides were determined by dry analytical methodology (Johnson & Johnson Clinical Diagnostics, Inc., NY, USA) using the USA population as a reference (non-HDL cholesterol >145 mg/dL; HDL <40 mg/dL; and triglycerides >130 mg/dL) (43).
The CMR score was defined by the sum of the blood related variables z-scores and WHtR, using the following equation (44): [glycaemia (z-score) + insulin (z-score) + triglycerides (z-score) – HDL (z-score)]/5. These variables were chosen because they are frequently used in adults as criteria to establish metabolic syndrome (7). In addition, scores above the 75th percentile were considered to indicate high risk of CMR (26,45).
Physical fitness
CRF was determined by a sub-maximal test, the 6-minute walk test (6MWT) (46,47). This test included a walking course of 30-m in length. The turnaround points were marked with a cone. The starting line, marked on the floor by brightly colored tape, indicated the beginning and end of each 60-m lap. The result obtained in the 6MWT was divided by height (48), because leg length was not evaluated (49). The values obtained were standardized into z-scores.
MF was assessed using both handgrip strength test and standing long jump test. Handgrip strength was measured using an adjustable handgrip digital dynamometer (Baseline 12-0286®; 100 g accuracy), and the results were expressed in kg. Each child performed handgrip twice by squeezing the dynamometer as hard as possible for at least two seconds. One minute of recovery between squeezes was measured (interchanging the left and right hands). Thus, the best result of two attempts was registered and, therefore, handgrip strength was normalized by body weight (kg) to consider body size differences (50). The values obtained were also converted into z-scores. The standing long jump test was carried out by starting in a flexed semi-squat position before initiating the upward phase and jump. This test was conducted on a 2-m long non-slippery surface, free of obstacles. A starting line was made to identify the initial position from the child must jump. The longest distance in cm was recorded (51) and, therefore, it was also transformed into z-score. MF score was determined as follows: handgrip strength/body weight (z-score) + standing long jump (z-score). Conversely, physical fitness (z-score) was computed as follows: 6MWT/body height (z-score) + handgrip/body weight (z-score) + standing long jump (z-score).
Physical fitness values (i.e., individual and combined CRF and MF z-scores) were categorized into two groups using a median split of the different results obtained in the physical tests as follows: high physical fitness (z-score) (>50th percentile) and low physical fitness (z-score) (≤50th percentile) (26).
Statistical analysis
Continuous variables are shown as frequencies and percentages for categorical variables and means and standard deviation for continuous variables. All model assumptions were checked (i.e., homoscedasticity, normality). Due to the non-normal distribution of some variables (i.e., handgrip strength, handgrip strength/body weight, triglycerides, insulin, HOMA-IR and BMI), the assumptions for executing an analysis of covariance (ANCOVA) were not met. Because of this, bootstrapping as a reliable technique to determine robust estimations of standard errors and confidence intervals (CIs) was performed. Thus, a robust bootstrapping ANCOVA with similar level of significance (P<0.05) was performed to control for confounding variables. Preliminary analyses showed no significant interactions between sex and CRF (P=0.115), MF (P=0.075), physical fitness (P=0.075), and BMI status (P=0.115) in relation to CMR; therefore, all the analyses were carried out with boys and girls together to increase the statistical power. Four different groups were established related to physical fitness and BMI: (I) low physical fitness/high BMI; (II) low physical fitness/low BMI; (III) high physical fitness/high BMI; and (IV) high physical fitness/low BMI. These groups were also created in relation to individual physical fitness components (i.e., CRF and MF). ANCOVAs were performed to determine mean differences of CMR related to combination of CRF, MF or physical fitness and BMI groups. Similarly, mean differences between groups were assessed by post-hoc analyses with adjustment of the CIs by Bonferroni test and adjusting by age, sex, and maturation stage. Lastly, we examined the odds of having high CMR in relation to the different physical fitness/BMI established groups through binary logistic regression analyses, after adjusting for age, sex, and pubertal status. All analyses were conducted using SPSS software, version 25.0. A two-sided P<0.05 indicates statistical significance.
Results
Table 1 shows the characteristics of the study participants. The age mean was 7.8±0.5 years old. The proportion of excess weight participants was 48.9%.
Table 1
Variables | Total (n=452) |
---|---|
Age (years) | 7.8±0.5 |
Weight (kg) | 28.0±8.1 |
Height (cm) | 126.9±5.7 |
BMI (kg/m2) | 17.9±2.7 |
BMI (z-score) | 0.98±1.16 |
Excess weighta | 221 (48.9) |
WHtR | 0.48±0.05 |
Body fat (%) | 29.8±7.6 |
Glycaemia (mg/dL) | 89.3±6.4 |
Triglyceride (mg/dL) | 95.9±40.9 |
High-density lipoprotein (mg/dL) | 49.8±13.3 |
Insulin (μU/dL) | 5.4±1.3 |
HOMA-IR | 1.2±0.5 |
Standing long jump (cm) | 108.0±16.3 |
Handgrip strength (kg) | 11.5±2.3 |
Handgrip strength/weight | 0.40±0.1 |
6MWT (m) | 625.2±53.7 |
6MWT/height | 503.6±49.8 |
Muscular fitness (z-score) | 1.14±2.1 |
6MWT/height (z-score) | 0.43±1.2 |
Data expressed as mean ± SD or n (%). a, excess weight determined as the sum of overweight and obesity following the World Health Organization criteria (36). 6MWT, 6-minute walk test; BMI, body mass index; HOMA-IR, homeostatic model assessment for insulin resistance; WHtR, waist-to-height ratio.
Figure 1 shows the mean differences in CMR score in relation to different physical fitness categories and excess weight status. Schoolchildren with high physical fitness (individual or combined) showed the lowest CMR mean scores (P for trend <0.001 for CRF, MF, and physical fitness groups). Conversely, schoolchildren with low physical fitness (individual or combined) showed the highest CMR mean scores (P for trend <0.001 for all categories). Additionally, schoolchildren without excess weight and high individual or combined physical fitness status exhibits lower CMR mean scores compared to schoolchildren with excess weight and low physical fitness status (individual or combined) (P for trend <0.001 for CRF, MF, and physical fitness groups). Differences in mean values of CMR in schoolchildren across different physical fitness and BMI groups established is found in Table S1. Moreover, a visual representation of the moderation of CRF, MF and physical fitness in the relationship between BMI groups and CMR can be found in Figure S1.
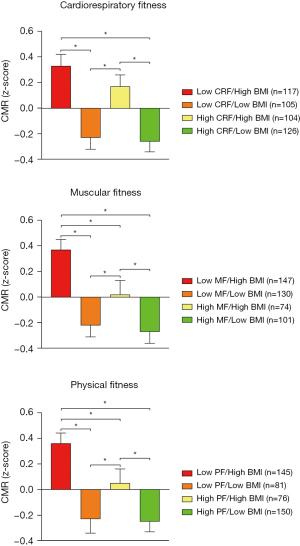
The odds of having CMR in schoolchildren across different physical fitness and BMI groups established are shown in Figure 2. A lower odd of having high CMR was found in schoolchildren without excess weight and with both high physical fitness (both CRF and MF) [odds ratio (OR) =0.08; 95% CI: 0.04 to 0.16], schoolchildren without excess weight schoolchildren and with high physical fitness (OR =0.11; 95% CI: 0.05 to 0.25), and excess weight schoolchildren with high physical fitness (OR =0.43; 95% CI: 0.23 to 0.78), in comparison with those excess weight schoolchildren with both low physical fitness. These lower odds were also found when examined physical fitness individually in both cases.

Discussion
In general, our results indicate that both high CRF and MF are inversely related to CMR score, which suggests that improvements in both BMI and physical fitness could be important for having a low CMR among young Chilean children.
We found no statistically significant differences in CMR score in relation to physical fitness in schoolchildren without excess weight. Thus, we failed to prove the “fat but fit” paradox in our study. Schoolchildren without excess weight and low physical fitness status showed lower CMR mean score than their counterparts with excess weight and high physical fitness status. This is in line with previous studies (26,28,52). For instance, Nyström et al. (28) pointed out that the “fat but fit paradox” was not confirmed by their results since CRF showed a beneficial effect on the metabolic profile only in children with obesity but not in their counterparts with normal weight. Sasayama et al. (26) did not find significant differences for CMR between high BMI/high CRF group and low BMI/low CRF group (in both sexes) in their study among Japanese children. This finding was also in line with a previous study among Australian children by Eisenmann et al. (52). One possible explanation justifying this finding is the mediator role of obesity in the associations of CRF (27) and MF (53) with CMR in schoolchildren, which could explain this finding. Despite the above, caution is required to interpret this finding. As previous studies, we used a non-standardized cut-off points to determine physical fitness groups, which could influence on the results obtained (28,31). Although Tomkinson et al. (54) established sex- and age-specific centiles for CRF from the 20-m Shuttle Run test including over 1 million children and adolescents from 50 countries, most of the data is from high-income and upper middle-income countries. Thus, these same authors have pointed out the need of data from children in low-income and middle-income countries. Similarly, the different methodology applied to determine CRF (6MWT instead of 20-m Shuttle Run test) could also influence on the results obtained. Additionally, we used World Health Organization cut-off points to establish excess weight. In this sense, further studies using more accurate cut-off points for adiposity, as well as studies involving only children with obesity in the high adiposity group are required (28).
On the other hand, it seems that a high MF plays a relevant role in CMR, since the lowest values of CMR mean score and the lowest odds of having high CMR were found when we analyzed MF individually. In this sense, a high MF is related to more adequate skeletal muscle function, which directly influences on the metabolic function (55) and could explain the lower CMR in our analyzed. Furthermore, it is remarkable that we normalized handgrip strength by body weight instead of including absolute handgrip strength, as previously suggested (56,57). This choice could have influenced on the results obtained. In this sense, Castro-Piñero et al. (58) compared the discriminatory ability of handgrip strength in relation to body weight and of absolute handgrip strength and showing that relative handgrip strength had significantly greater discriminatory ability to detect CMR. Our finding suggests that MF in schoolchildren may confer additional benefits to cardiometabolic health. Supporting this notion, most excess weight children are reluctant to participate in aerobic activities; therefore, the promotion of MF through muscle-strengthening activities could be more attractive and easier for excess weight children (59).
Another interesting finding is that we found that children without excess weight and high physical fitness showed lower odds of having high CMR. This finding is in line with other previous studies (26,28-30,52). For instance, Nyström et al. (28) found that CRF decreased the CMR score, with the highest differences in the most Spanish children with obesity analyzed. Likewise, Brouwer et al. (30) showed that a high physical fitness attenuates the association between fatness and CMR (and HOMA-IR) (in adolescent boys). This finding was also supported by Stoner et al. (29), which found that high CRF may moderate the relationship between fatness and CMR in New Zealand children. One possible explanation is that a high CRF, understood as the ability of the respiratory and circulatory systems to provide fuel during maintained physical activity, entails more stable physiological features (i.e., increased capillary density, greater resting energy expenditure) (60). These features triggered by high CRF could also influence on CMR (61), and might be more pronounced in schoolchildren with high physical fitness. In parallel, as previously mentioned, a high MF is related to low CMR (through improvements in skeletal muscle function) (55). Thus, the combination of these two physical fitness components could attenuate the CMR among excess weight schoolchildren. Supporting this notion, a meta-analysis by García-Hermoso et al. (62) found that concurrent aerobic plus strength exercise improves metabolic profiles, body composition and inflammatory state in obese young population.
Methodological considerations
Our results should be interpreted with certain caution because of the presence of some limitations in this study. Firstly, due to the cross-sectional design of this study, it is not possible to determine cause-and-effect relationships. Secondly, the present lack of consensus in young people in relation to the definition of CMR might limit our results. Thirdly, the 6MWT is a submaximal test to evaluate CRF, and it is not comparable with another aerobic field-tests (e.g., 20-m Shuttle Run test). However, the physical tests that we have used are simple to apply and well accepted among young children. Finally, we did not adjust our analysis by important confounders such as socio-economic status and dietary behavior. Conversely, there are some strengths that must be declared. For instance, we contribute to the scientific literature by adding information about physical fitness from schoolchildren in a low- to middle-income country (i.e., Chile). Similarly, we used a narrow age range of schoolchildren and standardized methods to collect the data.
Conclusions
This study suggests that improvements in both BMI and physical fitness could be important for having a low CMR among young Chilean children. Additional studies with different designs (e.g., longitudinal) are necessary to a further assessment of the independent and combined effects of CRF, MF and BMI in the prevention of CMR among schoolchildren.
Acknowledgments
The authors would like to thank the participation of all the children and schools implicated in this study. AGH is a Miguel Servet Fellow (Instituto de Salud Carlos III—CP18/0150). JFLG is a Postdoctoral Fellow (Universidad de Castilla-La Mancha—ID 2021-UNIVERS-10414).
Funding: This research was funded by Fondecyt (grant No. 1100206).
Footnote
Reporting Checklist: The authors have completed the STROBE reporting checklist. Available at https://tp.amegroups.com/article/view/10.21037/tp-22-25/rc
Data Sharing Statement: Available at https://tp.amegroups.com/article/view/10.21037/tp-22-25/dss
Conflicts of Interest: All authors have completed the ICMJE uniform disclosure form (available at https://tp.amegroups.com/article/view/10.21037/tp-22-25/coif). AGH serves as an unpaid editorial board member of Translational Pediatrics from August 2021 to July 2023.The other authors have no conflicts of interest to declare.
Ethical Statement: The authors are accountable for all aspects of the work in ensuring that questions related to the accuracy or integrity of any part of the work are appropriately investigated and resolved. The study was conducted in accordance with the Declaration of Helsinki (as revised in 2013). The study was approved by the Institute of Nutrition and Food Technology Ethics Committee for research in human subjects institutional and/or national research (Act No. 19, 2009). Parents or legal guardians of all participants signed an informed consent form before participation.
Open Access Statement: This is an Open Access article distributed in accordance with the Creative Commons Attribution-NonCommercial-NoDerivs 4.0 International License (CC BY-NC-ND 4.0), which permits the non-commercial replication and distribution of the article with the strict proviso that no changes or edits are made and the original work is properly cited (including links to both the formal publication through the relevant DOI and the license). See: https://creativecommons.org/licenses/by-nc-nd/4.0/.
References
- Pérez-Escamilla R, Segura-Pérez S, Lott M. Feeding Guidelines for Infants and Young Toddlers: A Responsive Parenting Approach. Nutr Today 2017;52:223-31. [Crossref]
- Pacheco LS, Blanco E, Burrows R, et al. Early Onset Obesity and Risk of Metabolic Syndrome Among Chilean Adolescents. Prev Chronic Dis 2017;14:E93. [Crossref] [PubMed]
- Junta de Auxilio Escolar y Becas de Chile. Prevalencia de Obesidad en escolares de Primero Básico. Mapa Nutricional. Santiago (Chile), 2017.
- Vásquez FD, Corvalán CL, Uauy RE, et al. Anthropometric indicators as predictors of total body fat and cardiometabolic risk factors in Chilean children at 4, 7 and 10 years of age. Eur J Clin Nutr 2017;71:536-43. [Crossref] [PubMed]
- Amatruda M, Ceravolo MD, Ceravolo G, et al. Cardiovascular risk factors in childhood obesity. J Biol Regul Homeost Agents 2020;34:11-16. Special Issue: Focus On Pediatric Cardiology. [PubMed]
- Cote AT, Harris KC, Panagiotopoulos C, et al. Childhood obesity and cardiovascular dysfunction. J Am Coll Cardiol 2013;62:1309-19. [Crossref] [PubMed]
- Kamel M, Smith BT, Wahi G, et al. Continuous cardiometabolic risk score definitions in early childhood: a scoping review. Obes Rev 2018;19:1688-99. [Crossref] [PubMed]
- Chung ST, Onuzuruike AU, Magge SN. Cardiometabolic risk in obese children. Ann N Y Acad Sci 2018;1411:166-83. [Crossref] [PubMed]
- Weiss R, Dziura J, Burgert TS, et al. Obesity and the metabolic syndrome in children and adolescents. N Engl J Med 2004;350:2362-74. [Crossref] [PubMed]
- Mattsson N, Rönnemaa T, Juonala M, et al. Childhood predictors of the metabolic syndrome in adulthood. The Cardiovascular Risk in Young Finns Study. Ann Med 2008;40:542-52. [Crossref] [PubMed]
- Zimmet P, Alberti KG, Kaufman F, et al. The metabolic syndrome in children and adolescents - an IDF consensus report. Pediatr Diabetes 2007;8:299-306. [Crossref] [PubMed]
- Schuler G, Adams V, Goto Y. Role of exercise in the prevention of cardiovascular disease: results, mechanisms, and new perspectives. Eur Heart J 2013;34:1790-9. [Crossref] [PubMed]
- Guthold R, Stevens GA, Riley LM, et al. Worldwide trends in insufficient physical activity from 2001 to 2016: a pooled analysis of 358 population-based surveys with 1·9 million participants. Lancet Glob Health 2018;6:e1077-86. Erratum in: Lancet Glob Health 2019 Jan;7(1):e36. [Crossref] [PubMed]
- Guthold R, Stevens GA, Riley LM, et al. Global trends in insufficient physical activity among adolescents: a pooled analysis of 298 population-based surveys with 1·6 million participants. Lancet Child Adolesc Health 2020;4:23-35. [Crossref] [PubMed]
- Aguilar-Farias N, Miranda-Marquez S, Martino-Fuentealba P, et al. Chilean Physical Activity Report Card for Children and Adolescents: Full Report and International Comparisons. J Phys Act Health 2018;2020: Epub ahead of print. [Crossref] [PubMed]
- Ortega FB, Ruiz JR, Castillo MJ, et al. Physical fitness in childhood and adolescence: a powerful marker of health. Int J Obes (Lond) 2008;32:1-11. [Crossref] [PubMed]
- Lang JJ, Larouche R, Tremblay MS. The association between physical fitness and health in a nationally representative sample of Canadian children and youth aged 6 to 17 years. Association entre la condition physique et la santé dans un échantillon représentatif à l’échelle nationale d’enfants et de jeunes canadiens de 6 à 17 ans. Health Promot Chronic Dis Prev Can 2019;39:104-11. [Crossref] [PubMed]
- García-Hermoso A, Ramírez-Campillo R, Izquierdo M. Is Muscular Fitness Associated with Future Health Benefits in Children and Adolescents? A Systematic Review and Meta-Analysis of Longitudinal Studies. Sports Med 2019;49:1079-94. [Crossref] [PubMed]
- García-Hermoso A, Ramírez-Vélez R, García-Alonso Y, et al. Association of Cardiorespiratory Fitness Levels During Youth With Health Risk Later in Life: A Systematic Review and Meta-analysis. JAMA Pediatr 2020;174:952-60. [Crossref] [PubMed]
- Ruiz JR, Cavero-Redondo I, Ortega FB, et al. Cardiorespiratory fitness cut points to avoid cardiovascular disease risk in children and adolescents; what level of fitness should raise a red flag? A systematic review and meta-analysis. Br J Sports Med 2016;50:1451-8. [Crossref] [PubMed]
- Artero EG, Ruiz JR, Ortega FB, et al. Muscular and cardiorespiratory fitness are independently associated with metabolic risk in adolescents: the HELENA study. Pediatr Diabetes 2011;12:704-12. [Crossref] [PubMed]
- Steene-Johannessen J, Anderssen SA, Kolle E, et al. Low muscle fitness is associated with metabolic risk in youth. Med Sci Sports Exerc 2009;41:1361-7. [Crossref] [PubMed]
- Fühner T, Kliegl R, Arntz F, et al. An Update on Secular Trends in Physical Fitness of Children and Adolescents from 1972 to 2015: A Systematic Review. Sports Med 2021;51:303-20. [Crossref] [PubMed]
- Ortega FB, Lavie CJ, Blair SN. Obesity and Cardiovascular Disease. Circ Res 2016;118:1752-70. [Crossref] [PubMed]
- Ortega FB, Ruiz JR, Labayen I, et al. The Fat but Fit paradox: what we know and don't know about it. Br J Sports Med 2018;52:151-3. [Crossref] [PubMed]
- Sasayama K, Ochi E, Adachi M. Importance of both fatness and aerobic fitness on metabolic syndrome risk in Japanese children. PLoS One 2015;10:e0127400. [Crossref] [PubMed]
- Díez-Fernández A, Sánchez-López M, Mora-Rodríguez R, et al. Obesity as a mediator of the influence of cardiorespiratory fitness on cardiometabolic risk: a mediation analysis. Diabetes Care 2014;37:855-62. [Crossref] [PubMed]
- Nyström CD, Henriksson P, Martínez-Vizcaíno V, et al. Does Cardiorespiratory Fitness Attenuate the Adverse Effects of Severe/Morbid Obesity on Cardiometabolic Risk and Insulin Resistance in Children? A Pooled Analysis. Diabetes Care 2017;40:1580-7. [Crossref] [PubMed]
- Stoner L, Pontzer H, Barone Gibbs B, et al. Fitness and Fatness Are Both Associated with Cardiometabolic Risk in Preadolescents. J Pediatr 2020;217:39-45.e1. [Crossref] [PubMed]
- Brouwer SI, Stolk RP, Liem ET, et al. The role of fitness in the association between fatness and cardiometabolic risk from childhood to adolescence. Pediatr Diabetes 2013;14:57-65. [Crossref] [PubMed]
- DuBose KD, Eisenmann JC, Donnelly JE. Aerobic fitness attenuates the metabolic syndrome score in normal-weight, at-risk-for-overweight, and overweight children. Pediatrics 2007;120:e1262-8. [Crossref] [PubMed]
- Mesa JL, Ruiz JR, Ortega FB, et al. Aerobic physical fitness in relation to blood lipids and fasting glycaemia in adolescents: influence of weight status. Nutr Metab Cardiovasc Dis 2006;16:285-93. [Crossref] [PubMed]
- Tomkinson GR, Lang JJ, Tremblay MS. Temporal trends in the cardiorespiratory fitness of children and adolescents representing 19 high-income and upper middle-income countries between 1981 and 2014. Br J Sports Med 2019;53:478-86. [Crossref] [PubMed]
- González L, Corvalán C, Pereira A, et al. Early adiposity rebound is associated with metabolic risk in 7-year-old children. Int J Obes (Lond) 2014;38:1299-304. [Crossref] [PubMed]
- Capon T. Standardised anatomical alignment of the head in a clinical photography studio. A comparison between the Frankfort Horizontal and the natural head position. J Vis Commun Med 2016;39:105-11. [Crossref] [PubMed]
- de Onis M, Onyango AW, Borghi E, et al. Development of a WHO growth reference for school-aged children and adolescents. Bull World Health Organ 2007;85:660-7. [Crossref] [PubMed]
- Gómez-Campos R, Andruske CL, Hespanhol J, et al. Waist Circumferences of Chilean Students: Comparison of the CDC-2012 Standard and Proposed Percentile Curves. Int J Environ Res Public Health 2015;12:7712-24. [Crossref] [PubMed]
- Tanner JM, Whitehouse RH. Clinical longitudinal standards for height, weight, height velocity, weight velocity, and stages of puberty. Arch Dis Child 1976;51:170-9. [Crossref] [PubMed]
- American Diabetes Association. Diagnosis and classification of diabetes mellitus. Diabetes Care 2014;37:S81-90. [Crossref] [PubMed]
- Radziuk J. Homeostastic model assessment and insulin sensitivity/resistance. Diabetes 2014;63:1850-4. [Crossref] [PubMed]
- Moschonis G, Karatzi K, Polychronopoulou MC, et al. Waist circumference, trunk and visceral fat cutoff values for detecting hyperinsulinemia and insulin resistance in children: the Healthy Growth Study. Eur J Nutr 2016;55:2331-4. [Crossref] [PubMed]
- Burrows R, Correa-Burrows P, Reyes M, et al. Healthy Chilean Adolescents with HOMA-IR ≥ 2.6 Have Increased Cardiometabolic Risk: Association with Genetic, Biological, and Environmental Factors. J Diabetes Res 2015;2015:783296. [Crossref] [PubMed]
- Smith AJ, Turner EL, Kinra S. Universal Cholesterol Screening in Childhood: A Systematic Review. Acad Pediatr 2016;16:716-25. [Crossref] [PubMed]
- Corvalán C, Uauy R, Stein AD, et al. Effect of growth on cardiometabolic status at 4 y of age. Am J Clin Nutr 2009;90:547-55. [Crossref] [PubMed]
- Peterson MD, Zhang P, Saltarelli WA, et al. Low Muscle Strength Thresholds for the Detection of Cardiometabolic Risk in Adolescents. Am J Prev Med 2016;50:593-9. [Crossref] [PubMed]
- ATS Committee on Proficiency Standards for Clinical Pulmonary Function Laboratories. ATS statement: guidelines for the six-minute walk test. Am J Respir Crit Care Med 2002;166:111-7. [Crossref] [PubMed]
- Li AM, Yin J, Au JT, et al. Standard reference for the six-minute-walk test in healthy children aged 7 to 16 years. Am J Respir Crit Care Med 2007;176:174-80. [Crossref] [PubMed]
- Arias Téllez MJ, Soto-Sánchez JP, Weisstaub SG. Physical fitness, cardiometabolic risk and heart rate recovery in Chilean children. Nutr Hosp 2018;35:44-9. [Crossref] [PubMed]
- Oliveira AC, Rodrigues CC, Rolim DS, et al. Six-minute walk test in healthy children: is the leg length important? Pediatr Pulmonol 2013;48:921-6. [Crossref] [PubMed]
- Ervin RB, Fryar CD, Wang CY, et al. Strength and body weight in US children and adolescents. Pediatrics 2014;134:e782-9. [Crossref] [PubMed]
- Castro-Piñero J, Ortega FB, Artero EG, et al. Assessing muscular strength in youth: usefulness of standing long jump as a general index of muscular fitness. J Strength Cond Res 2010;24:1810-7. [Crossref] [PubMed]
- Eisenmann JC, Welk GJ, Ihmels M, et al. Fatness, fitness, and cardiovascular disease risk factors in children and adolescents. Med Sci Sports Exerc 2007;39:1251-6. [Crossref] [PubMed]
- Díez-Fernández A, Sánchez-López M, Gulías-González R, et al. BMI as a mediator of the relationship between muscular fitness and cardiometabolic risk in children: a mediation analysis. PLoS One 2015;10:e0116506. [Crossref] [PubMed]
- Tomkinson GR, Lang JJ, Tremblay MS, et al. International normative 20 m shuttle run values from 1 142 026 children and youth representing 50 countries. Br J Sports Med 2017;51:1545-54. [Crossref] [PubMed]
- Agostinis-Sobrinho C, Abreu S, Moreira C, et al. Muscular fitness, adherence to the Southern European Atlantic Diet and cardiometabolic risk factors in adolescents. Nutr Metab Cardiovasc Dis 2017;27:695-702. [Crossref] [PubMed]
- Fraser BJ, Rollo S, Sampson M, et al. Health-Related Criterion-Referenced Cut-Points for Musculoskeletal Fitness Among Youth: A Systematic Review. Sports Med 2021;51:2629-46. [Crossref] [PubMed]
- López-Gil JF, Weisstaub G, Ramírez-Vélez R, et al. Handgrip strength cut-off points for early detection of cardiometabolic risk in Chilean children. Eur J Pediatr 2021;180:3483-9. [Crossref] [PubMed]
- Castro-Piñero J, Laurson KR, Artero EG, et al. Muscle strength field-based tests to identify European adolescents at risk of metabolic syndrome: The HELENA study. J Sci Med Sport 2019;22:929-34. [Crossref] [PubMed]
- Thivel D, Ring-Dimitriou S, Weghuber D, et al. Muscle Strength and Fitness in Pediatric Obesity: a Systematic Review from the European Childhood Obesity Group. Obes Facts 2016;9:52-63. [Crossref] [PubMed]
- Skrede T, Aadland E, Andersen LB, et al. Does cardiorespiratory fitness moderate the prospective association between physical activity and cardiometabolic risk factors in children? Int J Obes (Lond) 2018;42:1029-38. [Crossref] [PubMed]
- Ekelund U, Anderssen SA, Froberg K, et al. Independent associations of physical activity and cardiorespiratory fitness with metabolic risk factors in children: the European youth heart study. Diabetologia 2007;50:1832-40. [Crossref] [PubMed]
- García-Hermoso A, Ramírez-Vélez R, Ramírez-Campillo R, et al. Concurrent aerobic plus resistance exercise versus aerobic exercise alone to improve health outcomes in paediatric obesity: a systematic review and meta-analysis. Br J Sports Med 2018;52:161-6. [Crossref] [PubMed]