Hearing characteristics and cochlear implant effects in children with Waardenburg syndrome: a case series
Introduction
Waardenburg syndrome (WS), first reported by the Dutch ophthalmologist Waardenburg in 1951, is a group of phenotypic features resulting from the absence of melanocytes in the skin, hair, eyes, and stria vascularis of the cochlea. WS is mainly an autosomal dominant inheritance with incomplete penetrance disease, and it can also have autosomal recessive inheritance. Inner ear deformities are related to some types of WS, but not all WS (1). About 2–5% of congenital deafness is caused by WS (2).
The incomplete penetrance of WS phenotypes and the diversity of causative genes result in a high degree of clinical and genetic heterogeneity. WS is classified into 4 types according to different accompanying symptoms. The most common types are type I and type II (WS1 and WS2). Except for the absence of ectopic medial canthus, other clinical manifestations of WS2 are the same as those of WS1. The diagnosis of WS1 is mainly based on the criteria developed by Farrer et al. in 1992 (3) and Liu et al. in 1995 (4) and those recommended by the Waardenburg Association. The other 3 types are diagnosed based on WS1. WS-related genes include PAX3 (encoding the paired box 3 transcription factor), MITF (microphthalmia-associated transcription factor), EDN3 (endothelin 3), EDNRB (endothelin receptor type B), SOX10 (SRY-box transcription factor 10) (5), and SNAI2 (snail homolog 2) (6,7). For WS1 (OMIM#193500), PAX3 is the causative gene. The main diagnostic criteria of WS1 include heterochromia iris, sensorineural hearing loss, frontal white hair, ectopic medial canthus (W ≥1.95), and first-degree relatives suffering from WS. The secondary criteria of WS1 are high and wide nose root, skin vitiligo, straight eyebrows, early gray hair, and poor nasal alar development (8). To diagnose WS1, 2 major criteria or 1 major criterion plus 2 secondary criteria must be met (9). WS2 (OMIM#193510, 608890, 611584) has pathogenic genes including MITF, SNAI2, and SOX10. Clinical manifestations of WS2 are the same as WS1, except for the absence of ectopic medial canthus. Two main criteria should be used for the diagnosis of WS2. For type III WS (WS3, OMIM#144820), also known as Klein-Waardenburg syndrome, PAX3 is the pathogenic gene. Besides clinical manifestations of WS1, limb muscle spasm and joint contractures can occur in WS3 (10,11). Type IV WS (WS4, OMIM#277580, 613265, 613266), also known as Shah-Waardenburg syndrome, has pathogenic genes EDNRB, EDN3, and SOX10. Besides typical manifestations of WS, WS4 can also combine with Hirschsprung’s disease, which is accompanied by gastrointestinal malformations such as neonatal intestinal obstruction (12).
In this paper, we analyzed the clinical characteristics of 5 WS children with severe sensorineural hearing loss diagnosed and treated in our center in the past 5 years, as well as their recovery of auditory and speech abilities after cochlear implantation. We present the following article in accordance with the AME Case Series and STROBE reporting checklists (available at https://tp.amegroups.com/article/view/10.21037/tp-22-271/rc).
Methods
This is a retrospective case series. The study was conducted in accordance with the Declaration of Helsinki (as revised in 2013). The study was approved by Shanghai Children’s Hospital, School of Medicine, Shanghai Jiao Tong University (No. 2022R062-E01). Informed consent was taken from all the patients’ parents or legal guardians. Five patients were recruited from the Department of Otolaryngology, Shanghai Children’s Hospital from 2017 to 2022, and these WS cases were followed up for 6 to 24 months. Audiology tests included otoacoustic emission (OAE), auditory brainstem response (ABR), and multiple auditory steady-state evoked responses (ASSR). Preoperative CT and MRI were performed to evaluate inner ear development and brain development. Nucleus CI512 cochlear implants were implanted under general anesthesia with a retroauricular incision and transfacial recess approach to implant through the round window. The WS index is used to objectively evaluate whether the patient has ectopic inner canthus (W ≥1.95). W = (2A-0.2119B-3.909)/C + (2A-0.2749B-3.909)/B + A/B, where A is the intercanthal distance, B is the interpupillary distance, and C is the lateral intercanthal distance.
WS cases were assessed for hearing and speech ability before cochlear implantation and at 1, 6, 12, and 24 months after implantation. Auditory ability was evaluated using the infant toddler meaningful auditory integration scale (IT-MAIS). Each item is scored from 0–4 based on the frequency of the child’s reaction: 0 points indicates that the situation never occurs (0%), 1 point indicates that the situation rarely occurs (25%), 2 points indicates an occasional occurrence (50%), 3 points indicates a frequent occurrence (75%), and 4 points indicates that it always occurs (100%). IT-MAIS has 40 points in total, and a higher score means better hearing ability. The score rate is used for statistics. IT-MAIS score (%) = (IT-MAIS score/40) ×100%.
Speech ability was assessed using the meaningful use of speech scale (MUSS) (13). The scale includes 10 open-ended questions, which are answered by parents or guardians who know the child’s situation. They need to provide a detailed description of the child’s speech and behavior in daily life on which points are given. The score for each question is 0 to 4: 0 indicates that the child never uses the vocalizations or speech behaviors; 1 indicates that the child rarely uses the vocalizations or speech behaviors, with a frequency of less than 50%; 2 indicates that the child occasionally uses the vocalizations or speech behaviors with at least 50% frequency; 3 indicates that the child frequently uses the vocalizations or speech behaviors with at least 75% frequency; 4 indicates that the child frequently uses the vocalizations or speech behaviors, with a frequency of 100%. The total score is 40, and a higher score indicates a better ability to produce speech. The MUSS score was converted to a percentage to analyze data, where MUSS score (%) = MUSS score/40 × 100%.
Statistical analysis
IT-MAIS score and IT-MAIS score of each case were presented as percentage of total score, figures were made in GraphPad Prism (version 9.2, San Diego, CA, USA).
Results
Five children, including 2 males and 3 females, were diagnosed with WS and had bilateral severe sensorineural deafness (Table 1). These children were full-term first births. Parents denied consanguineous marriage, there was no history of viral infection during pregnancy, and no family history of genetic diseases or any other special diseases or medication. No patients with similar manifestations were found in the immediate family members going back 3 generations.
Table 1
Characteristics | Case 1 | Case 2 | Case 3 | Case 4 | Case 5 |
---|---|---|---|---|---|
Gender | Female | Male | Male | Female | Female |
Age of implantation (months) | 12 | 8 | 21 | 10 | 9 |
Gene mutation | PAX3 | SOX10 | PAX3 | SOX10 | PAX3 |
Iris heterochromia | Right | Bilateral | Bilateral | Bilateral | Right |
Forehead white hair | No | No | No | No | No |
One-word eyebrow | Yes | No | No | No | No |
High and wide nose | Yes | Yes | No | Yes | No |
A, intercanthal distance (cm) | 4.5 | 3.2 | 4.5 | 3.4 | 4.4 |
B, interpupillary distance (cm) | 5.1 | 6.1 | 5.3 | 6.2 | 5 |
C, lateral intercanthal distance (cm) | 9 | 8.5 | 9.1 | 8.3 | 9 |
W* | 2.05 | 0.91 | 1.97 | 0.93 | 2.01 |
Vitiligo | No | No | No | No | No |
Hirschsprung’s disease | No | Short segment | No | No | No |
ABR (dBnHL) | Bilateral >97 | Bilateral >97 | Bilateral >97 | Bilateral >97 | Bilateral >97 |
Ear CT | Normal | Normal | Normal | CH-IV# | Normal |
Head MRI | Abnormal white matter myelination | Widening of subarachnoid space | Abnormal white matter myelination | Abnormal white matter myelination | Abnormal white matter myelination |
Type | WS1 | WS4 | WS1 | WS2 | WS1 |
Cochlear implant | Bilateral | Right | Right | Bilateral | Bilateral |
*, W = (2A-0.2119B-3.909)/C + (2A-0.2749B-3.909)/B + A/B; #, CH-IV, cochlea with hypoplastic middle and apical turns. ABR, auditory brainstem response; WS, Waardenburg syndrome; CT, computerized tomography; MRI, magnetic resonance imaging.
Case 1 in this series was 12 months old and had PAX3 mutation, bilateral extremely severe sensorineural deafness, heterochromia of the right eye, medial canthal shift (W=2.05), high and wide nasal root, and one-word eyebrows. Case 1 met 3 major criteria and 2 minor criteria, and was classified as WS1. Case 2 was 8 months old and had SOX10 mutation, bilateral heterochromia, and high and wide nasal roots, accompanied by Hirschsprung’s colon, and the case had been surgically treated in another hospital. Case 2 was classified as WS4. Case 3 was 21 months old and had PAX3 mutation, bilateral extremely severe sensorineural hearing loss, bilateral iris heterochromia, and ectopic medial canthus (W=1.97). The case met 3 major criteria and was classified as WS1. Case 4 had SOX10 mutation, bilateral extremely severe sensorineural deafness, bilateral iris heterochromia, and high and wide nasal root. Two major criteria were met, and the case was considered as WS2. Case 5 had PAX3 mutation, bilateral extremely severe sensorineural hearing loss, right iris heterochromia, and ectopic medial canthus (W=2.01). The case met 3 major criteria and was classified as WS1.
WS infants failed the hearing screening of both ears at birth. Hearing examination showed that the ABR in both ears of the 5 WS cases was greater than 97 dBnHL, and OAE failed in both ears. Ear CT examination showed bilateral cochlea with hypoplastic middle and apical turns (CH-IV) (14) (Figure 1A,1B), vestibular enlargement, and semicircular canal dysplasia in Case 4 (Figure 1C,1D). No obvious abnormality was found in the other 4 cases. In the head MRI examination, the subarachnoid space was widened in Case 2, and the other 4 cases showed abnormal white matter myelination.
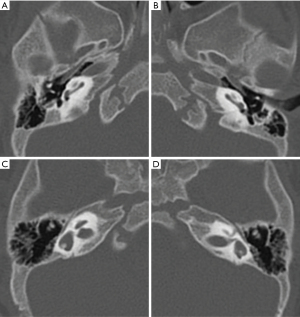
These 5 WS cases underwent cochlear implantation at ages ranging from 8 to 21 months (Table 1). Postoperative CT showed that the implants were in a good position. WS cases were evaluated for hearing and speech ability before the operation and at 1, 6, 12, and 24 months after the operation. Case 4 is currently 6 months old, so there is no follow-up evaluation. The results showed that the IT-MAIS and MUSS scores of WS cases increased with hearing age (Figure 2A,2B), and IT-MAIS scores were lower than those of normal hearing children of the same age.
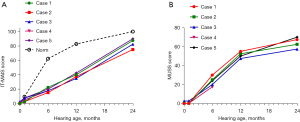
Discussion
Children with WS in this case series had hearing loss. WS cases may have cochlear hypoplasia, vestibular enlargement, and semicircular canal dysplasia, as well as pathogenic mutations. With cochlear implantation, WS cases showed improvement of hearing and speech ability.
The theory of neural crest hypoplasia is the most widely accepted etiological hypothesis of WS. Neural crest cells are a group of pluripotent cells that gradually evolve into melanocytes through proliferation, migration, and differentiation. Melanocytes migrate to the dermis, epidermis, and iris and choroid of the eyes, and inner ear blood vessels to convert into melanocytes to produce melanin (2).
In Case 4, cochlear hypoplasia was observed, accompanied by vestibular enlargement and semicircular canal dysplasia, and SOX10 was mutated. The most common inner ear malformations in patients with WS are enlargement of the vestibular aqueduct and malformation of the semicircular canals (dilated or absent) (15,16). The incidence of vestibular dysfunction has not been accurately described, and either temperature or rotation tests can suggest vestibular dysfunction, but dizziness is rare (17,18). Song et al. analyzed temporal bone images of 24 WS patients with PAX3 or SOX10 gene mutations and severe deafness (19). Five cases were normal, and 19 cases had bilateral inner ear malformations with SOX10 mutation. The most common malformation was semicircular canal malformation. Among the 19 cases, all had semicircular canal agenesis or hypoplasia, 18 cases had vestibular enlargement or malformation, and 12 cases had small cochlea size and abnormal appearance. Three patients had cochlear nerve underdevelopment, 2 of which were bilateral. No vestibular function tests or vestibular symptoms were discussed in the review.
Mutations in WS-related genes result in a lack of melanocyte-derived intermediate cells in the stria vascularis, which leads to degeneration of the organ of Corti and sensorineural hearing loss. Song et al. reviewed 73 papers with a total of 417 WS patients and found that about 71% of WS patients had hearing loss, mostly bilateral and sensorineural, and only 1 case had mixed hearing loss without conductive hearing loss and typical features on the audiogram (19). The incidence of different WS types varied significantly: WS1 was 52.3%, WS2 was 91.6%, WS3 was 57.1%, and WS4 was 83.5%. In addition, the incidence of hearing loss caused by pathogenic gene mutations also varies. The incidence rates of mutation in the following 3 genes were significantly higher than other pathogenic genes: SOX10 was 96.5%, MITF was 89.6%, and SNAI2 was 100%, which were different compared to the findings of 90 Chinese WS probands (20). WS patients usually do not have cognitive impairment, and hearing loss is usually caused by lesions of the cochlear histology, spiral ganglion neurons are still able to receive stimulation from cochlear implants, therefore cochlear implantation as a supportive treatment of WS is effective (21).
IT-MAIS is a structured questionnaire in which evaluators ask 10 open-ended questions to parents or guardians who are familiar with the child, and scores are given based on the feedback. IT-MAIS was designed based on 4 stages of auditory development, namely perception, discrimination, recognition, and understanding. Questions 1 and 2 are based on sound production of hearing-impaired children after wearing hearing aids. Questions 3 to 6 determine the ability of WS patients to perceive sounds, while questions 7 to 10 explore the ability to distinguish and understand sounds (22). IT-MAIS can effectively reflect the auditory ability of hearing-impaired children in the initial stage of wearing hearing aids. In this disease series, Case 4 had inner ear deformity, and had only been followed up for 6 months by May, 2022. Therefore, Case 4 will be followed up continuously to evaluate the development of hearing and speech ability. IT-MAIS scores of the other 4 cases increased with hearing age, but were still lower than those of normal hearing children in China of the same ages (23). A study has shown that time length after cochlear implantation is a key factor affecting the postoperative outcome (24). The longer the implantation time, the more significant the effects of cochlear implants, the better the auditory and speech abilities, and the smaller the difference between WS patients and normal hearing children.
The development of hearing ability in children with cochlear implantation is slower than that of normal hearing children. Preoperative auditory rehabilitation, preoperative residual hearing, listening habits, postoperative adjustment, postoperative rehabilitation, and parental cooperation will affect the performance of WS children with cochlear implantation (25). Since hearing is the basis of language, hearing-impaired infants and young children who receive early intervention have higher scores in language and communication skills (26,27). Besides, the golden period for the development of the central auditory system is younger than 3.5 years old, and early surgery ensures that the child can develop language and communication skills based on hearing ability, which may help achieve a better postoperative rehabilitation effect (28,29). However, pediatric CI users have higher electrically evoked compound action potentials (ECAPs) thresholds than adult CI users over 96 months follow-up (30), which means a decline in neural response and might need an increasing postoperative electrical stimulation over a long time.
Conclusions
Children with WS often have different degrees of hearing loss, and temporal bone imaging may show abnormal development of the cochlea, vestibule, and semicircular canals. WS children with severe sensorineural hearing loss can achieve better hearing and speech development from early cochlear implantation. Future studies should include more cases from multiple centers and follow up cases over a longer period of time.
Acknowledgments
Funding: This work was funded by Shanghai Children’s Hospital, School of Medicine, Shanghai Jiao Tong University.
Footnote
Reporting Checklist: The authors have completed the AME Case Series and STROBE reporting checklists. Available at https://tp.amegroups.com/article/view/10.21037/tp-22-271/rc
Data Sharing Statement: Available at https://tp.amegroups.com/article/view/10.21037/tp-22-271/dss
Conflicts of Interest: All authors have completed the ICMJE uniform disclosure form (available at https://tp.amegroups.com/article/view/10.21037/tp-22-271/coif). The authors have no conflicts of interest to declare.
Ethical Statement: The authors are accountable for all aspects of the work in ensuring that questions related to the accuracy or integrity of any part of the work are appropriately investigated and resolved. The study was conducted in accordance with the Declaration of Helsinki (as revised in 2013). The study was approved by Shanghai Children’s Hospital, School of Medicine, Shanghai Jiao Tong University (No. 2022R062-E01). Informed consent was taken from all the patients’ parents or legal guardians.
Open Access Statement: This is an Open Access article distributed in accordance with the Creative Commons Attribution-NonCommercial-NoDerivs 4.0 International License (CC BY-NC-ND 4.0), which permits the non-commercial replication and distribution of the article with the strict proviso that no changes or edits are made and the original work is properly cited (including links to both the formal publication through the relevant DOI and the license). See: https://creativecommons.org/licenses/by-nc-nd/4.0/.
References
- Kontorinis G, Goetz F, Lanfermann H, et al. Inner ear anatomy in Waardenburg syndrome: radiological assessment and comparison with normative data. Int J Pediatr Otorhinolaryngol 2014;78:1320-6. [Crossref] [PubMed]
- Pingault V, Ente D, Dastot-Le Moal F, et al. Review and update of mutations causing Waardenburg syndrome. Hum Mutat 2010;31:391-406. [Crossref] [PubMed]
- Farrer LA, Grundfast KM, Amos J, et al. Waardenburg syndrome (WS) type I is caused by defects at multiple loci, one of which is near ALPP on chromosome 2: first report of the WS consortium. Am J Hum Genet 1992;50:902-13. [PubMed]
- Liu XZ, Newton VE, Read AP. Waardenburg syndrome type II: phenotypic findings and diagnostic criteria. Am J Med Genet 1995;55:95-100. [Crossref] [PubMed]
- Pingault V, Zerad L, Bertani-Torres W, et al. SOX10: 20 years of phenotypic plurality and current understanding of its developmental function. J Med Genet 2022;59:105-14. [Crossref] [PubMed]
- Shelby MV. Waardenburg Syndrome Expression and Penetrance. J Rare Dis Res Treat 2017;2:31-40. [Crossref] [PubMed]
- Huang S, Song J, He C, et al. Genetic insights, disease mechanisms, and biological therapeutics for Waardenburg syndrome. Gene Ther 2021; Epub ahead of print. [Crossref] [PubMed]
- Astakhov YS, Tultseva SN, Lisochkina AB, et al. Ophthalmologic manifestations of Waardenburg syndrome. Vestn Oftalmol 2019;135:91-9. [Crossref] [PubMed]
- Gowda VK, Srinivas S, Srinivasan VM. Waardenburg Syndrome Type I. Indian J Pediatr 2020;87:244. [Crossref] [PubMed]
- Klein D. Albinisme partiel (leucisme) avec surdi-mutite, blepharophimosis et dysplasie myo-osteo-articulaire. Helv Paediatr Acta 1950;5:38-58.
- Goodman RM, Lewithal I, Solomon A, et al. Upper limb involvement in the Klein-Waardenburg syndrome. Am J Med Genet 1982;11:425-33. [Crossref] [PubMed]
- Touraine RL, Attié-Bitach T, Manceau E, et al. Neurological phenotype in Waardenburg syndrome type 4 correlates with novel SOX10 truncating mutations and expression in developing brain. Am J Hum Genet 2000;66:1496-503. [Crossref] [PubMed]
- Robbins AM, Osberger MJ. Meaningful use of speech scale (MUSS). Indianopolis: Indiana University School of Medicine, 1990.
- Sennaroğlu L, Bajin MD. Classification and Current Management of Inner Ear Malformations. Balkan Med J 2017;34:397-411. [Crossref] [PubMed]
- Madden C, Halsted MJ, Hopkin RJ, et al. Temporal bone abnormalities associated with hearing loss in Waardenburg syndrome. Laryngoscope 2003;113:2035-41. [Crossref] [PubMed]
- Higashi K, Matsuki C, Sarashina N. Aplasia of posterior semicircular canal in Waardenburg syndrome type II. J Otolaryngol 1992;21:262-4. [PubMed]
- Black FO, Pesznecker SC, Allen K, et al. A vestibular phenotype for Waardenburg syndrome? Otol Neurotol 2001;22:188-94. [Crossref] [PubMed]
- Hageman MJ, Oosterveld WJ. Vestibular findings in 25 patients with Waardenburg's syndrome. Arch Otolaryngol 1977;103:648-52. [Crossref] [PubMed]
- Song J, Feng Y, Acke FR, et al. Hearing loss in Waardenburg syndrome: a systematic review. Clin Genet 2016;89:416-25. [Crossref] [PubMed]
- Wang G, Li X, Gao X, et al. Analysis of genotype-phenotype relationships in 90 Chinese probands with Waardenburg syndrome. Hum Genet 2022;141:839-52. [Crossref] [PubMed]
- Polanski JF, Kochen AP, de Oliveira CA. Hearing and speech performance after cochlear implantation in children with Waardenburg syndrome. Codas 2020;32:e20180295. [Crossref] [PubMed]
- Zimmerman-Phillips S, Robbins AM, Osberger MJ. Assessing cochlear implant benefit in very young children. Ann Otol Rhinol Laryngol Suppl 2000;185:42-3. [Crossref] [PubMed]
- Xu TQ, Chen XQ, Wang H. A normative study of auditory development in Mandarin-speaking infants. Zhonghua Er Bi Yan Hou Tou Jing Wai Ke Za Zhi 2013;48:908-12. [PubMed]
- Yoshida H, Takahashi H, Kanda Y, et al. Long term speech perception after cochlear implant in pediatric patients with GJB2 mutations. Auris Nasus Larynx 2013;40:435-9. [Crossref] [PubMed]
- Chen XQ, Wang L, Kong Y, et al. Auditory capability evaluation for children after cochlear implantation using meaningful auditory integration scale. Zhonghua Er Bi Yan Hou Tou Jing Wai Ke Za Zhi 2006;41:112-5. [PubMed]
- Yoshinaga-Itano C, Sedey AL, Wiggin M, et al. Language Outcomes Improved Through Early Hearing Detection and Earlier Cochlear Implantation. Otol Neurotol 2018;39:1256-63. [Crossref] [PubMed]
- Jiang F, Alimu D, Qin WZ, et al. Long-term functional outcomes of hearing and speech rehabilitation efficacy among paediatric cochlear implant recipients in Shandong, China. Disabil Rehabil 2021;43:2860-5. [Crossref] [PubMed]
- Miyamoto RT, Kirk KI, Svirsky MA, et al. Communication skills in pediatric cochlear implant recipients. Acta Otolaryngol 1999;119:219-24. [Crossref] [PubMed]
- Yamazaki H, Suehiro A, Ueda Y, et al. Early Cochlear Implantation Allows Deaf Children to Control Voice Pitch and Loudness independently. Otol Neurotol 2022;43:e56-63. [Crossref] [PubMed]
- Brown CJ, Abbas PJ, Etlert CP, et al. Effects of long-term use of a cochlear implant on the electrically evoked compound action potential. J Am Acad Audiol 2010;21:5-15. [Crossref] [PubMed]