Development of a model for early differentiation of adenovirus pneumonia from Mycoplasma pneumoniae pneumonia
Introduction
Community-acquired pneumonia (CAP) is widespread in the world, especially in developing countries. It is also one of the leading causes of death in children <5 years (1). The clinical characteristics and prognoses of CAP caused by various pathogens differ. Mycoplasma pneumoniae (M. pneumoniae) and adenovirus are common pathogens in CAP. M. pneumoniae is responsible for 7–20% of pediatric CAP (2), while adenovirus accounts for 4–10% and can cause severe pneumonia (3). Both pathogens can lead to similar presentations in the early clinical stage, such as prolonged high fever, severe cough, fatigue, no wet rales on auscultation, normal or slightly elevated C-reactive protein (CRP) and white blood cell count (WBC), and lobar consolidation on radiography. These similarities make the differential diagnosis difficult (4). Therefore, it is a priority to identify the pathogen to distinguish adenovirus pneumonia (AVP) from M. pneumoniae pneumonia (MPP). Even if results of microbiologic testing is obtained, however, it has limitations (5). Correctly distinguishing between AVP and MPP is a challenge. In economically and medically underdeveloped places settings, it may not be possible to determine the etiology. Hence, in addition to specific microbiologic testing, simple indicators are urgently needed for differential diagnosis. This could provide an alternative method to assist pediatricians with determining the etiology.
There have been few studies to date, that have compared the clinical characteristics of MPP and AVP in children (6). Previous studies have tended to have a small sample size and were unable to differentiate AVP from MPP at the early stage. We carried out this study to assist in the early identification of AVP and MPP by the combined evaluation of clinical features, biomarkers and radiographic features. In this study, the records of hospitalized children with CAP were analyzed. Low-dose computed tomography (CT) scans were performed in each case to assess the extent and severity of the lung lesions. Logistic regression analysis was used to build a model to predict AVP. We present the following article in accordance with the STARD reporting checklist (available at https://tp.amegroups.com/article/view/10.21037/tp-226/rc).
Methods
Study design
We conducted a retrospective, single-center study. Patients with CAP admitted to the emergency department, pediatric intensive care units, respiratory wards, and infectious wards were screened for eligibility. The study was conducted in accordance with the Declaration of Helsinki (as revised in 2013). Written informed consent was taken from all patients’ parents or legal guardians before enrollment. This study was approved by the Ethics Committee of Xinhua Hospital Affiliated to Shanghai Jiao Tong University School of Medicine (No. XHEC-C-2018-107).
Study population
Data were collected from August 30, 2014 to December 30, 2019 on children aged <14 years hospitalized with CAP in Xinhua Hospital affiliated with the Shanghai Jiao Tong University School, Shanghai, China. According to CAP guidelines (7), children were included if they had the following: (I) evidence of fever, cough, shortness of breath, wheezing; (II) signs of pneumonia, such as dyspnea, cyanosis, moist rales, rhonchus; (III) radiographic findings of pneumonia. Exclusion criteria were as follows: (I) neonatal pneumonia; (II) congenital heart or chronic lung disease; (III) coinfection with pathogens other than M. Pneumoniae and adenovirus; (IV) long-term use of glucocorticoids orimmunosuppressents.
Patients were divided into following two groups: (I) AVP group: Only positive for adenovirus infection and clinical manifestations were consistent; or positive for adenovirus and coinfection with M. pneumoniae; and (II) MPP group: Only positive for M. pneumoniae and clinical manifestations were consistent. Evidence of adenovirus infection (8) was as follows: adenovirus-IgM positive in serum; serum or the bronchoalveolar lavage fluid (BALF) positive for adenovirus on next-generation sequencing (NGS). Evidence of M. pneumoniae infection was as follows: serum M. pneumoniae-IgM ≥1:160, or M. pneumoniae-DNA positive on real-time polymerase chain reaction using a sputum sample, or the BALF positive for M. pneumoniae on NGS. The doctor fully evaluated the medical history and laboratory tests of the two groups.
Data collection
Demographic and clinical data were systematically collected by trained doctors. Data included sex, age, weight, admission season, fever duration, highest temperature, hypoxemia, cough duration before admission, neurological symptoms, rash, duration of hospitalization, and mean arterial pressure. Data of radiographic findings on CT scan were collected. Laboratory data were collected on CRP; complete blood count; inflammation indicators such as interleukin (IL)-1, IL-2 receptor (IL-2R), IL-6, IL-8, IL-10, lactic dehydrogenase (LDH), tumor necrosis factor (TNF)-α, ferroprotein, liver function markers such as total bilirubin, bile acid, alanine aminotransferase, aspartate aminotransferase, alkaline phosphatase, and albumin; kidney function markers such as creatinine; cardiac function; and electrolyte levels. Blood microbiology data were collected on M. pneumoniae-IgM, adenovirus-IgM, human respiratory syncytial virus-IgM, human metapneumovirus-IgM, IgM of influenza viruses A and B. Microbiology data were collected on sputum and BALF, including DNA of M. pneumoniae and Chlamydia, bacterial Gram stain and culture. All the results mentioned above were collected from the inpatient medical record system.
Radiographic examination and confirmation
All the children received a low dose chest CT within 48 h after admission. Low-dose CT was defined as 256-slice CT at a dose of less than 0.3 mSv/a. Radiographic evidence of pneumonia was defined as the presence of multilobar infiltration, bilateral pneumonia, ground-glass attenuation, interstitial reticulation opacities, consolidation, pleural effusion, atelectasis (8,9). The radiographic characteristics were collected from the inpatient medical record system.
Definitions
CAP guidelines issued by the Infectious Diseases Society of America (IDSA) (9).
The Pediatric Logistic Organ Dysfunction-2 score (PELOD-2) was used to describe the severity of organ dysfunction (10).
Early stage: the period before microbiologic testing were reported.
Hypoxemia: sustained saturation of peripheral oxygen (SpO2) <90% at sea level.
Severe pneumonia: the definition comes from the CAP guidelines (9) but excluded the criterion for Multilobar infiltration. Major criteria for the assessment of the severity of pneumonia were: invasive mechanical ventilation, fluid refractory shock, acute need for noninvasive positive pressure ventilation, and hypoxemia requiring FiO2 greater than inspired concentration or flow feasible in the general care area. The minor criteria were: respiratory rate higher than World Health Organization classification for age, apnea, increased work of breathing (retractions, dyspnea, nasal flaring, grunting), PaO2/FiO2 ratio <250, altered mental status, hypotension, presence of effusion, comorbid conditions (immunosuppression, immunodeficiency), and unexplained metabolic acidosis.
Statistical analyses
Categorical variables were expressed as frequencies and percentages. The chi-square test was used to compare groups. Continuous variables were tested for normality. Non-normally distributed continuous data were presented as the median (interquartile range). Comparisons among groups were performed using the Kruskal–Wallis test. A logistic regression model was established to predictive AVP. In the model, we introduced clinically and statistically significant variables with P<0.05 from the previous univariate analysis. Forward stepwise regression was used to select variables in the multivariable analysis. Results were reported as odds ratios (ORs) with 95% confidence intervals (CIs). Receiver-operating characteristic curve analysis was used to assess predictors of AVP identified by the multivariable logistic regression model, and each respective area under the curve (AUC) was calculated to evaluate the discriminant ability of the prediction model. All statistical analyses were performed using Empower R (http://www.empowerstats.com, X&Y Solutions, Inc, Boston MA, USA) and R software (http://www.T-project.org). Two-tailed P values <0.05 were considered statistically significant for all analyses.
Results
General characteristics and clinical features
Data were collected on 8,520 children aged <14 years during the study period. A total of 1,074 patients met the inclusion criteria, including 198 patients with AVP and 876 patients with MPP. The study flow chart is presented in Figure 1. AVP and MPP occurred all year round, but the incidence rate was highest in the spring (>40% in both groups) (Table 1). The patients in the AVP group were mainly infants and toddlers, while the MPP group had more pre-school age children. Correspondingly, the body weight of AVP group was significantly lower than that of MPP group (P<0.01). The proportion of boys in the AVP group was 63.6%, which was significantly higher than that in the MPP group (46.0%, P<0.01). In both groups, the clinical manifestations included a high fever and a prolonged cough. Approximately half of the children in each group developed moist rales during the course of the disease. The duration of fever was significantly longer in the AVP group than in the MPP group (P<0.01), while patients in the MPP group had a significantly higher prevalence of irritant dry cough than the AVP group (P<0.01) (Table 1).
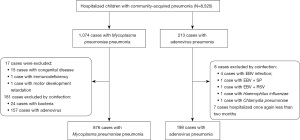
Table 1
Characteristics | AVP (N=198) | MPP (N=876) | P value |
---|---|---|---|
Male | 126 (63.6) | 403 (46.0) | <0.01 |
Age, years | 2.0 (1.1–3.2) | 5.0 (3.0–7.0) | <0.01 |
Weight, kg | 15.0 (12.0–19.0) | 19.5 (15.0–25.0) | <0.01 |
Season | 0.60 | ||
Spring | 84 (42.4) | 363 (41.4) | |
Summer | 54 (27.3) | 214 (24.4) | |
Autumn | 46 (23.2) | 214 (24.4) | |
Winter | 14 (7.1) | 85 (9.7) | |
Fever duration, days | 10.0 (7.0–17.0) | 8.0 (6.0–10.0) | <0.01 |
Highest temperature ≥39 ℃ | 155 (78.3) | 693 (79.1) | 0.80 |
Hypoxemia | 11 (5.6) | 16 (1.8) | <0.01 |
Cough duration before admission, days | 9.0 (5.0–15.0) | 7.0 (5.0–10.0) | <0.01 |
Dry cough | 26 (13.1) | 205 (23.4) | <0.01 |
Night cough | 45 (22.7) | 215 (24.5) | 0.59 |
Wheezing | 21 (10.6) | 89 (10.2) | 0.12 |
Moist rales | 109 (55.1) | 394 (45.0) | 0.01 |
Severe pneumonia | 55 (27.8) | 22 (2.5) | < 0.01 |
PELOD-2 score ≥2 score | 32 (17.4) | 15 (1.7) | < 0.01 |
Hospitalization length, days | 8.0 (5.0–13.0) | 7.0 (5.0–9.0) | 0.02 |
Age, weight, fever duration, cough duration, hospitalization length are reported as median (interquartile range). The remaining variables are reported as n (%). AVP, adenovirus pneumonia; MPP, Mycoplasma pneumoniae pneumonia; PELOD-2, pediatric logistic organ dysfunction-2 score.
Clinical severity
The respiratory disease was more severe in the AVP group. The rate of hypoxemia was 3 times higher in the AVP group than in the MPP group (5.6% vs. 1.8%, P<0.01), and 3 patients in the AVP group were treated with mechanical ventilation. The rate of severe pneumonia was 11-fold higher in the AVP group than that in the MPP group (27.8% vs. 2.5%, P<0.01). In the AVP group, the number of patients with PELOD-2 score ≥2 score was 10-fold higher than in the MPP group (17.4% vs. 1.7%, P<0.01). Consistent with the greater disease severity, the length of hospitalization was significantly longer in the AVP group (P=0.02) (Table 1).
Radiographic and laboratory findings
The pathological features of the respiratory tract disease were seen more clearly on chest CT (Table 2). Multiple lobar infiltrations, bilateral pneumonia, ground-glass attenuation and consolidation was present in >50% of the children in both groups. The AVP group had a wider range of lung lesions, with 90.2% having signs of bilateral lung involvement (P<0.01). In addition, a few children in both groups had atelectasis and small pleural effusions. The WBC and neutrophil counts in both groups were close to normal values, while the levels of CRP and inflammatory cytokines, such as IL-6, IL-8, and TNF-α, were slightly elevated (Table 3), but there were no significant differences between the two groups. IL-2R, IL-10 and LDH were significantly higher in the AVP group than in the MPP group (P<0.01).
Table 2
CT characteristics | AVP (N=198) | MPP (N=876) | P value |
---|---|---|---|
Multilobar infiltration | 144 (73.1) | 653 (76.1) | 0.38 |
Bilateral pneumonia | 164 (82.8) | 461 (53.8) | <0.01 |
Ground-glass attenuation | 126 (64.0) | 595 (74.1) | <0.01 |
Interstitial reticulation opacities | 42 (21.3) | 255 (31.8) | <0.01 |
Consolidation | 105 (53.0) | 750 (85.6) | <0.01 |
Multilobar consolidation† | 7 (17.1) | 215 (24.7) | 0.42 |
Pleural effusion | 27 (13.7) | 149 (17.4) | 0.21 |
Atelectasis | 8 (4.0) | 84 (10.2) | <0.01 |
Variables are presented as n (%). †, consolidation of two or more lobes. AVP, adenovirus pneumonia; MPP, Mycoplasma pneumoniae pneumonia.
Table 3
Biomarkers | AVP (N=198) | MPP (N=876) | P value |
---|---|---|---|
CRP, mg/L | 10.0 (1.0–22.0) | 11.0 (4.0–25.0) | <0.01 |
WBC, 109/L | 7.4 (5.0–9.9) | 6.9 (5.3–9.1) | 0.37 |
Neutrophil, % | 52.5 (34.7–67.0) | 57.5 (44.8–67.8) | <0.01 |
Platelet count, 109/L | 293.0 (218.0–375.0) | 287.0 (223.8–363.0) | 0.69 |
IL-1, pg/mL | 2.5 (2.5–8.8) | 2.5 (2.5–8.7) | 0.95 |
IL-2R, U/mL | 1,247.5 (943.2–1,782.2) | 1,002.5 (741.5–1,417.5) | <0.01 |
IL-6, pg/mL | 12.6 (5.0–62.7) | 13.90 (6.17–70.75) | 0.42 |
IL-8, pg/mL | 28.6 (14.1–131.5) | 23.4 (10.1–191.0) | 0.19 |
IL-10, pg/mL | 7.3 (2.5–15.3) | 2.5 (2.5–9.3) | <0.01 |
LDH, U/L | 376.0 (302.0–548.0) | 342.0 (287.2–409.0) | <0.01 |
TNF-α, pg/mL | 15.6 (11.6–25.8) | 16.3 (11.3–36.3) | 0.33 |
Variables are reported as median (interquartile range). AVP, adenovirus pneumonia; MPP, Mycoplasma pneumoniae pneumonia; CRP, C-reactive protein; WBC, white blood cell count; IL, interleukin; IL-2R, interleukin 2 receptor; LDH, lactic dehydrogenase; TNF, tumor necrosis factor.
The receiver operating characteristic curve
Although the characteristics of AVP and MPP were similar in many aspects, there were differences between the two groups. Therefore, a logistic regression model was developed to predict AVP through clinical features. To develop the model, clinically and statistically significant variables from the previous univariate analysis, such as male, age, hypoxemia, dry cough, moist rales, severe pneumonia, bilateral pneumonia, ground-glass attenuation, interstitial reticulation opacities, consolidation, atelectasis, CRP, neutrophil, IL-2R, IL-10 and LDH were considered as potential predictors. Then, age, severe pneumonia, bilateral pneumonia, ground-glass attenuation, consolidation, atelectasis, CRP and LDH were selected for inclusion in the final predictive model (Table 4). The receiver-operating characteristic curve was analyzed (Figure 2) and each respective AUC was calculated to evaluate the discriminant ability of the prediction model. The AUC was 86.6% (95% CI: 83.1–90.1%). The optimal cutoff of the scale for predicting AVP was 0.364. The corresponding sensitivity and specificity were 67.3% and 92.0%, respectively. The Youden index was 59.4%.
Table 4
Variables | OR (95% CI) | P value |
---|---|---|
Age, years | 0.910 (0.825–1.004) | 0.06 |
Severe pneumonia | 12.462 (5.334–29.114) | <0.01 |
Bilateral pneumonia | 6.112 (3.079–12.133) | <0.01 |
Ground-glass attenuation | 0.352 (0.203–0.612) | <0.01 |
Consolidation | 0.073 (0.040–0.132) | <0.01 |
Atelectasis | 0.233 (0.062–0.875) | 0.03 |
CRP, mg/L | 0.987 (0.975–0.999) | 0.03 |
LDH, U/L | 1.002 (1.001–1.003) | <0.01 |
Constant | ··· | 0.31 |
OR, odds ratio; CI, confidence interval; CRP, C-reactive protein; LDH, lactic dehydrogenase.
Discussion
In this study, we evaluated the differences in clinical presentation, radiographic features, and biomarkers between AVP and MPP. Then, a logistic regression model was used to select variables for prediction. The role of this predictive model is to assist clinicians differentiate between the two types of pneumonia from another perspective. It is especially suitable for areas where pathogen detection is difficult to carry out, such as primary care hospitals and resource-limited countries. In addition, even though advanced hospitals conduct microbiological tests, each microbiological test method has limitations (5). Current diagnostic tests, such as M. pneumoniae DNA positive in blood sample or upper respiratory tract sputum, do not reliably differentiate between M. pneumoniae infection and carriage (11). Therefore, the combination use of the two methods can more accurately determine the real pathogen. Furthermore, differentiate AVP from MPP in the “early stage” may avoid inappropriate empirical use of antibiotics at the beginning of treatment. M. pneumoniae as well as several different bacteria and viruses can produce lower respiratory infections with similar clinical manifestations (12). Previously, clinicians were accustomed to empirically using macrolide antibiotics in the face of lung consolidation and severe pneumonia before microbiological results. However, the etiological results showed that it was actually an adenovirus infection a few days later.
The peak periods of AVP and MPP has been reported in each season (13-16). This study found that most of the children developed symptoms in the spring, which may be related to the fact that our patients come from southern China, where the climate is relatively warm. It was consistent with multicenter epidemiological study (17). The significant clinical differences between AVP and MPP include differences in the age distributions (7), total fever duration (18) and presence of an irritant dry cough. These characteristic differences were also observed in this study. Irritant dry cough after M. pneumoniae infected, associated with M. pneumoniae adhere to pseudostratified columnar ciliated epithelia, resulting in ciliary destruction and reduced wobble (19). However, generally AVP was associated with a more severe illness than MPP. In this study, children with AVP had longer duration of fever, larger range of lung lesions, higher proportion of bilateral pneumonia and severe pneumonia than children with MPP. This may because adenovirus directly infects lung tissue and causes cytolysis and necrosis, and activates the immune response of the body, leading to a severe inflammatory response (20). Another evidence was that the rate of hypoxemia in the AVP group was significantly higher than in the MPP group. Hypoxemia, important in the clinical diagnosis of pneumonia (21), is another manifestation of severe disease and indicates the risk of death is increased (22). The reason may be that AVP causes necrotizing bronchointerstitial pneumonia with hemorrhage and edema that is capable of impairing oxygenation of blood.
This study found that children with AVP and MPP had several radiographic similarities. Lobar pneumonia and even consolidation could be found both in AVP and MPP. Adenovirus is the only known human virus to cause lobar pneumonia, which is an imaging/gross pathologic feature typically reserved bacterial bronchopneumonia. In addition, more than half of children had multilobar infiltration, bilateral pneumonia, consolidation, and ground-glass attenuation in AVP and MPP. The high rate of multilobar infiltration and bilateral pneumonia indicates that these two types of pneumonia also had a wide range of lung involvement and differ from lobar pneumonia caused by Streptococcus pneumoniae, which is generally unilateral (23). Ground-glass attenuation and consolidation could be caused by non-cellular infiltrate, such as edema or hemorrhage. They can also be caused by exudate and neutrophil infiltration wihin bronchiolar and alveolar lumens (24). Biopsy is required for a definitive histopathologic correlation. Combined with the increase of Th1/Th2 cytokine profiles, this indicates that the radiographic manifestations of adenovirus or M. pneumoniae infection were related to the inflammatory response (25). The CT imaging features of MPP include ground-glass attenuation, consolidation, thickened bronchial wall, and nodules (26). This study found that children with MPP also had interstitial reticulation opacities and atelectasis. The prevalence of these six radiographic abnormalities were considerably higher in the MPP group than in the AVP group, indicating that the imaging performance of MPP was more serious. Consolidation, atelectasis as well as cough, being more common findings in MPP makes sense acknowledging this bacterial disease causes obstructive suppurative bronchopneumonia. This may be related to adhesion damage (27) and inflammatory damage (28) after M. pneumoniae infection. Inflammatory damage manifested as an increase in the levels of IL-2R, IL-6, and TNF-α in this study.
In this study, biomarkers such as CRP and WBC were not useful for judging the severity of AVP or MPP. Inflammatory markers are often considered as parameters of predictive models of lung infection and sepsis (29). Multiple cytokines stimulated by severe MPP can be used as indicators to predict the disease of children (30). During the period of adenovirus infection in children, the host immune system also activates many inflammatory cytokines (31). In this study, a large number of cytokines were elevated in both the AVP and MPP groups. The IL-2R, IL-10 and LDH in the AVP group were significantly higher than those in the MPP group. The increase of IL-2R indicates that IL-2 promotes the expression of IL-2R in B lymphocytes, which promotes the proliferation of B lymphocytes and the production of immunoglobulins. And it stimulates macrophages to improve their phagocytic ability. IL-10 is a multicellular and multifunctional cytokine. Both type 2 T-helper cells and regulatory T cells can produce IL-10 (32). IL-10 plays an important anti-inflammatory role in B cell-induced immune phenotype. It was characterized by a significant reduction in levels of proinflammatory cytokines and augmented production of anti-inflammatory IL-1 receptor antagonist (33). However, the overexpression of IL-10 can inhibit the clearance of pathogens, leading to secondary bacterial lung infection (34). Increased LDH is related to inflammation and cellular hypoxia, and can be used as a biomarker to predict refractory MPP (35). The high expression of biomarkers indicated that AVP produced a stronger inflammatory response.
The manifestations of pneumonia caused by various pathogens are often non-specific. There is no single symptom or index that can differentiate between different types of pneumonia. Hence, many researchers have built models to predict various types of pneumonia, such as MPP (36), refractory MPP (37,38), and AVP (20). Most previous studies of preductors have small sample sizes, or the prediction effect was not as expected. Large studies such as this study are rare. Thus, we built this prediction model with 8 prognostic variables, including age, severe pneumonia, bilateral pneumonia, ground-glass attenuation, consolidation, atelectasis, CRP and LDH. Clinical features combined with CT images and laboratory results can improve the accuracy of prediction models (36). The predictive power of the scale developed in this study had good performance on AVP. The AUC was 86.6%, with moderate sensitivity and high specificity. The variables included in this study are relatively simple to measure and readily available, which makes the predictors suitable for application in clinical work.
This study has several limitations. First, MPP data were collected from 2015 to 2019, while AVP data from 2018 to 2019. Although there was an AVP epidemic in East China from January 2018 to December 2019, data on AVP from 2015 to 2017 are still lacking. The size of the AVP was smaller than that of the MPP group. Additionally, even though we detected adenovirus, we did not proceed with any further typing. The severity of AVP differs according to the adenovirus type. Previous studies have shown that adenovirus-7 causes the most severe disease in Mainland China (39,40). Furthermore, although parameters such as hospitalization length, ventilator use, and PELOD-2 scores were used to evaluate the severity of disease among the two groups, the frequency of use of non-invasive respiratory support, such as oxygen therapy and nasal continuous positive airway pressure, was not compared. Similarly, inflammatory markers such as procalcitonin and serum amyloid A were not considered.
Conclusions
In conclusion, compared with MPP, AVP affects younger children, presents a more severe respiratory tract involvement, shows a larger range of lung injuries on CT imaging, and is associated with higher levels of inflammatory biomarkers. Our predictive model used a combination of clinical and radiographic features, and biomarkers. It may help pediatricians in differentiating AVP from MPP in the early stage.
Acknowledgments
We thank all participants and staff of this study and the physicians at the Xinhua Hospital affiliated to Shanghai Jiao Tong University School of Medicine.
Funding: This work was supported by the National Natural Science Foundation of China (No. 81874265), Shanghai Municipal Health Commission (No. 2017YQ033), Shanghai Municipal Human Resources and Social Security Bureau (No. 2018003), Shanghai Jiao Tong University School of Medicine (No. BYH20180206), Shanghai Science and Technology Commission (Nos. 18411966600 and 19410740800), National Natural Science Foundation of China (No. 81971929) and Wenzhou Municipal Science and Technology Bureau (No. Y20190003).
Footnote
Reporting Checklist: The authors have completed the STARD reporting checklist. Available at https://tp.amegroups.com/article/view/10.21037/tp-22-6/rc
Data Sharing Statement: Available at https://tp.amegroups.com/article/view/10.21037/tp-22-6/dss
Conflicts of Interest: All authors have completed the ICMJE uniform disclosure form (available at https://tp.amegroups.com/article/view/10.21037/tp-22-6/coif). LH reports funding supported by National Natural Science Foundation of China (No. 81874265), Shanghai Municipal Health Commission (No. 2017YQ033), Shanghai Municipal Human Resources and Social Security Bureau (No. 2018003), Shanghai Jiao Tong University School of Medicine (No. BYH20180206), and Shanghai Science and Technology Commission (Nos. 18411966600 and 19410740800). YC reports funding supported by National Natural Science Foundation of China (No. 81971929) and Wenzhou Municipal Science and Technology Bureau (No. Y20190003). The other authors have no conflicts of interest to declare.
Ethical Statement:
Open Access Statement: This is an Open Access article distributed in accordance with the Creative Commons Attribution-NonCommercial-NoDerivs 4.0 International License (CC BY-NC-ND 4.0), which permits the non-commercial replication and distribution of the article with the strict proviso that no changes or edits are made and the original work is properly cited (including links to both the formal publication through the relevant DOI and the license). See: https://creativecommons.org/licenses/by-nc-nd/4.0/.
References
- He C, Liu L, Chu Y, et al. National and subnational all-cause and cause-specific child mortality in China, 1996-2015: a systematic analysis with implications for the Sustainable Development Goals. Lancet Glob Health 2017;5:e186-97. [Crossref] [PubMed]
- Izumikawa K, Izumikawa K, Takazono T, et al. Clinical features, risk factors and treatment of fulminant Mycoplasma pneumoniae pneumonia: a review of the Japanese literature. J Infect Chemother 2014;20:181-5. [Crossref] [PubMed]
- Jobran S, Kattan R, Shamaa J, et al. Adenovirus respiratory tract infections in infants: a retrospective chart-review study. Lancet 2018;391:S43. [Crossref] [PubMed]
- Søndergaard MJ, Friis MB, Hansen DS, et al. Clinical manifestations in infants and children with Mycoplasma pneumoniae infection. PLoS One 2018;13:e0195288. [Crossref] [PubMed]
- Meyer Sauteur PM. Challenges and Progress Toward Determining Pneumonia Etiology. Clin Infect Dis 2020;71:514-6. [Crossref] [PubMed]
- Gao J, Xu L, Xu B, et al. Human adenovirus Coinfection aggravates the severity of Mycoplasma pneumoniae pneumonia in children. BMC Infect Dis 2020;20:420. [Crossref] [PubMed]
- National Health Commission of the People’s Republic of China, State Administration of Traditional Chinese Medicine. Guideline for diagnosis and treatment of community-acquired pneumonia in Children (2019 version). Chin J Clin Infect Dis 2019;12:06-13.
- National Health Commission of the People’s Republic of China, State Administration of Traditional Chinese Medicine. Guideline for diagnosis and treatment of adenovirus pneumonia in Children (2019 version). Chin J Clin Infect Dis 2019;12:161-6.
- Bradley JS, Byington CL, Shah SS, et al. The management of community-acquired pneumonia in infants and children older than 3 months of age: clinical practice guidelines by the Pediatric Infectious Diseases Society and the Infectious Diseases Society of America. Clin Infect Dis 2011;53:e25-76. [Crossref] [PubMed]
- Leteurtre S, Duhamel A, Salleron J, et al. PELOD-2: an update of the PEdiatric logistic organ dysfunction score. Crit Care Med 2013;41:1761-73. [Crossref] [PubMed]
- Spuesens EB, Fraaij PL, Visser EG, et al. Carriage of Mycoplasma pneumoniae in the upper respiratory tract of symptomatic and asymptomatic children: an observational study. PLoS Med 2013;10:e1001444. [Crossref] [PubMed]
- Waites KB, Xiao L, Liu Y, et al. Mycoplasma pneumoniae from the Respiratory Tract and Beyond. Clin Microbiol Rev 2017;30:747-809. [Crossref] [PubMed]
- Luby JP. Pneumonia caused by Mycoplasma pneumoniae infection. Clin Chest Med 1991;12:237-44. [Crossref] [PubMed]
- Johnson DH, Cunha BA. Atypical pneumonias. Clinical and extrapulmonary features of Chlamydia, Mycoplasma, and Legionella infections. Postgrad Med 1993;93:69-72, 75-6, 79-82. [Crossref] [PubMed]
- Lieberman D, Lieberman D, Friger MD. Seasonal variation in hospital admissions for community-acquired pneumonia: a 5-year study. J Infect 1999;39:134-40. [Crossref] [PubMed]
- Xie L, Zhang B, Xiao N, et al. Epidemiology of human adenovirus infection in children hospitalized with lower respiratory tract infections in Hunan, China. J Med Virol 2019;91:392-400. [Crossref] [PubMed]
- Duan YL, Zhu Y, Xu BP, et al. Multicenter study of human adenovirus infection in pediatric community-acquired pneumonia in China. Zhonghua Er Ke Za Zhi 2019;57:27-32. [PubMed]
- Zhao MC, Wang L, Qiu FZ, et al. Impact and clinical profiles of Mycoplasma pneumoniae co-detection in childhood community-acquired pneumonia. BMC Infect Dis 2019;19:835. [Crossref] [PubMed]
- Jiang Z, Li S, Zhu C, et al. Mycoplasma pneumoniae Infections: Pathogenesis and Vaccine Development. Pathogens 2021;10:119. [Crossref] [PubMed]
- Sun J, Xiao Y, Zhang M, et al. Serum Inflammatory Markers in Patients with Adenovirus Respiratory Infection. Med Sci Monit 2018;24:3848-55. [Crossref] [PubMed]
- Shah SN, Bachur RG, Simel DL, et al. Does This Child Have Pneumonia?: The Rational Clinical Examination Systematic Review. JAMA 2017;318:462-71. [Crossref] [PubMed]
- Zampoli M, Mukuddem-Sablay Z. Adenovirus-associated pneumonia in South African children: Presentation, clinical course and outcome. S Afr Med J 2017;107:123-6. [Crossref] [PubMed]
- Haroon A, Higa F, Fujita J, et al. Pulmonary computed tomography findings in 39 cases of Streptococcus pneumoniae pneumonia. Intern Med 2012;51:3343-9. [Crossref] [PubMed]
- Tanaka H. Correlation between Radiological and Pathological Findings in Patients with Mycoplasma pneumoniae Pneumonia. Front Microbiol 2016;7:695. [Crossref] [PubMed]
- Zhao JL, Wang X, Wang YS. Relationships between Th1/Th2 cytokine profiles and chest radiographic manifestations in childhood Mycoplasma pneumoniae pneumonia. Ther Clin Risk Manag 2016;12:1683-92. [Crossref] [PubMed]
- Miyashita N, Sugiu T, Kawai Y, et al. Radiographic features of Mycoplasma pneumoniae pneumonia: differential diagnosis and performance timing. BMC Med Imaging 2009;9:7. [Crossref] [PubMed]
- Shimizu T, Kimura Y, Kida Y, et al. Cytadherence of Mycoplasma pneumoniae induces inflammatory responses through autophagy and toll-like receptor 4. Infect Immun 2014;82:3076-86. [Crossref] [PubMed]
- Narita M, Tanaka H. Cytokines involved in the severe manifestations of pulmonary diseases caused by Mycoplasma pneumoniae. Pediatr Pulmonol 2007;42:397. [Crossref] [PubMed]
- Zhang Y, Mei S, Zhou Y, et al. Cytokines as the good predictors of refractory Mycoplasma pneumoniae pneumonia in school-aged children. Sci Rep 2016;6:37037. [Crossref] [PubMed]
- Chkhaidze I, Kapanadze N. Cytokines as the predictors of severe mycoplasma pneumoniae pneumonia in children (review). Georgian Med News 2017;89-95. [PubMed]
- Wang H, Zhou Q, Dai W, et al. Lung Microbiota and Pulmonary Inflammatory Cytokines Expression Vary in Children With Tracheomalacia and Adenoviral or Mycoplasma pneumoniae Pneumonia. Front Pediatr 2019;7:265. [Crossref] [PubMed]
- Uyeda MJ, Freeborn RA, Cieniewicz B, et al. BHLHE40 Regulates IL-10 and IFN-γ Production in T Cells but Does Not Interfere With Human Type 1 Regulatory T Cell Differentiation. Front Immunol 2021;12:683680. [Crossref] [PubMed]
- Stanic B, van de Veen W, Wirz OF, et al. IL-10-overexpressing B cells regulate innate and adaptive immune responses. J Allergy Clin Immunol 2015;135:771-80.e8. [Crossref] [PubMed]
- Goulding J, Godlee A, Vekaria S, et al. Lowering the threshold of lung innate immune cell activation alters susceptibility to secondary bacterial superinfection. J Infect Dis 2011;204:1086-94. [Crossref] [PubMed]
- Lu A, Wang C, Zhang X, et al. Lactate Dehydrogenase as a Biomarker for Prediction of Refractory Mycoplasma pneumoniae Pneumonia in Children. Respir Care 2015;60:1469-75. [Crossref] [PubMed]
- Meyer Sauteur PM, Krautter S, Ambroggio L, et al. Improved Diagnostics Help to Identify Clinical Features and Biomarkers That Predict Mycoplasma pneumoniae Community-acquired Pneumonia in Children. Clin Infect Dis 2020;71:1645-54. [Crossref] [PubMed]
- Zhou Y, Wang J, Chen W, et al. Impact of viral coinfection and macrolide-resistant mycoplasma infection in children with refractory Mycoplasma pneumoniae pneumonia. BMC Infect Dis 2020;20:633. [Crossref] [PubMed]
- Bi Y, Zhu Y, Ma X, et al. Development of a scale for early prediction of refractory Mycoplasma pneumoniae pneumonia in hospitalized children. Sci Rep 2021;11:6595. [Crossref] [PubMed]
- Fu Y, Tang Z, Ye Z, et al. Human adenovirus type 7 infection causes a more severe disease than type 3. BMC Infect Dis 2019;19:36. [Crossref] [PubMed]
- Wen S, Lin Z, Zhang Y, et al. The Epidemiology, Molecular, and Clinical of Human Adenoviruses in Children Hospitalized With Acute Respiratory Infections. Front Microbiol 2021;12:629971. [Crossref] [PubMed]